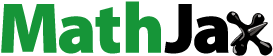
1. Introduction
Cyclist position on his bicycle and individual anthropometric parameters are very important to optimise pedalling biomechanics (Bini et al. Citation2011). Postural imbalance can lead to increase joint stress in the lower limbs. Therefore, different methods are used to reduce these joint stresses such as chiropractic, kinesio taping or the use of foot orthotics. The latter seem to be of interest to reduce parasitic movements during the pedalling cycle (e.g. pelvis tilt) and to restore the symmetry of the movements, both during the pushing and pulling phases of the pedal.
Hice et al. (Citation1985) measured a significant decrease in oxygen consumption (VO2) and heart rate with foot orthotics in laboratory. Moreover, Yang (Citation2013) showed that, when cycling with personal bicycle, the contribution of foot orthotics had beneficial effects on muscular activity of vastus medialis, vastus lateralis and gastrocnemius medialis which are recruited during the pushing phase. The activation time of those muscles decreases, which leads to a decrease in muscular fatigue, while the peak muscle power increases.
The scientific literature is controversial on the influence of foot orthotics in cycling. Therefore, the aim of our study was to analyse the acute effect of foot orthotics on gross efficiency (GE) and comfort in cyclists affected by an anatomic asymmetry in TT position. We hypothesize that foot orthotics could increase comfort and GE with a decrease in VO2 considering better stability on the saddle.
2. Methods
Twelve cyclists volunteered to participate in the study. All participants were licensed to the French Cycling Federation and were assigned into two groups, a test group (TG; n = 6) and a control group (CG; n = 6) based on their anatomic asymmetry. All participants gave their written informed consents after received full explanation of the protocol.
The participants performed two 11-min testing sessions separated by one hour (before and after orthopaedic correction) in TT position using their personal TT bicycle fixed on a Hammer Direct Drive Trainer (CycleOps, Madison, USA) at three different intensities (3, 3.5 and 4 W kg−1). Each intensity lasted 2 min. These intensities were separated by 1 min at 2 W kg−1 whereas the first intensity was preceded by 3 min at 2 W kg−1. The pedalling cadence was free during the first testing session and the cyclists had to reproduce the same pedalling cadence and TT position during the second session.
All participants have been examined between the two testing sessions 1) to detect a lower limb length inequality (LLLI) with current clinical assessments (Brady et al. Citation2003). Firstly, clinical exam on the table validates a LLLI higher than 5 mm. Secondly, standing clinical exam validates the pelvis tilt induced by the LLLI on the same side. Thirdly, cycling clinical exam validates the pelvis tilt on the saddle. The cyclists without pelvis tilt were included in the CG and received no change for the second testing session. The cyclists with the three signs of pelvis tilt are included in the TG and received custom foot orthotics molded under the feet with medial arch support and a 3 millimeters’ spacer under the shoes for the side of the lower limb. The cyclists were aware of these changes.
The workload was automatically adjusted by the Hammer Direct Drive Trainer via Vitual Training software and recorded on the same platform. Metabolic measurements were done with a breath-by-breath device (Metalyzer 3B-R3, Cortex, Leipzig, Germany). Metalyzer 3B-R3 measures breathing flow through bi directional digital turbine and 2.2 m sample line tube collect inspired and expired air to measure O2 and CO2 concentrations. O2 consumption (VO2, L min−1) and CO2 production (VCO2, L min−1) were calculated using standard metabolic algorithms. GE was calculated from measures of energy expended (EE, J s−1) and mechanical power (PO) averaged over the last minute of each intensity. As presented previously (Moseley and Jeukendrup Citation2001):
With EE = [(3.869 × VO2) + (1.195 × VCO2)] × [(4.186/60) × 1000].
Finally, a visual analogue scale from 0 (very uncomfortable) to 10 (very comfortable) was used to assess the comfort of fourteen different anatomical locations at the end of each session: left and right feet, left and right lower limbs, saddle, back, head, left and right upper limbs, left and right elbows, left and right hands and general comfort. All these data were averaged to obtain a unique comfort rating.
The data of the three intensities were averaged and analyzed with a paired t-test. We used effect size (ES, Cohen’s d) to estimate the magnitude of the difference between the two testing sessions in GE and comfort. The difference was considered trivial when ES ≤ 0.2, small when ES ≤ 0.5, moderate when ES ≤ 0.8, and large when ES > 0.8.
3. Results and discussion
The Figure shows that the mean GE did not change significantly in the TG (+1.1%, p = 0.608 and ES = 0.205) after the laying of the foot orthotics whereas it was a tendency to decrease in the CG (-4.6%, p = 0.058 and ES = 0.452). The decrease in GE in the CG could be explained by fatigue.
Figure 1. Scatterplot representing the acute effect of biomechanical foot orthotics on gross efficiency (CG: control group; TG: test group). Grey lines: individual values; black lines: mean values.
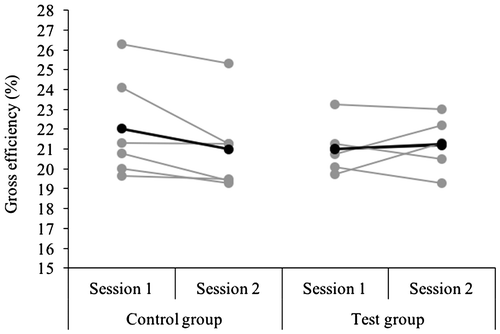
Individual responses are measured in TG because three participants increased their GE in the second session while the other three decreased their GE. Moreover, the perceived comfort did not change significantly in both CG (7.6 vs. 7.3, p = 0.363 and ES = 0.406) and TG (8.0 vs. 7.3, p = 0.102 and ES = 0.478) in the second test. By considering the difference between TG and CG, this study shows a trend for which biomechanical foot orthotics, in cyclists affected by a LLLI, slightly improve the GE (+5.7%) and comfort (+4.5%) in TT position.
4. Conclusions
This study demonstrates a trend for biomechanical foot orthotics to improve both the GE and comfort, which are determinant in cycling performance. However, future studies with a larger sample should investigate this topic considering the individual responses measured.
Acknowledgements
The authors wish to thank the participating cyclists for their cooperation and the Matsport Company for its material support.
References
- Bini RR, Hume PA, Croft JL. 2011. Effects of bicycle saddle height on knee injury risk and cycling performance. Sports Med. 41:463–476.10.2165/11588740-000000000-00000
- Brady RJ, Dean JB, Skinner TM, Gross MT. 2003. Limb length inequality: clinical implications for assessment and intervention. J Orthop Sports Phys Ther. 33:221–234.10.2519/jospt.2003.33.5.221
- Hice G, Kendrick Z, Weeber K, Bray J. 1985. The effect of foot orthoses on oxygen consumption while cycling. J Am Podiatr Med Assoc. 75:513.10.7547/87507315-75-10-513
- Moseley L, Jeukendrup AE. 2001. The reliability of cycling efficiency. Med Sci Sports Exercise. 33:621–627.10.1097/00005768-200104000-00017
- Yang S. 2013. The efficacy of arch support sports insoles in increasing the cycling performance and injury prevention. Footwear Sci. 5:S107–S109.10.1080/19424280.2013.799586