1. Introduction
Recording the motion of the scapula during manual wheelchair (MWC) propulsion is of particular interest but remains a challenge due to the soft tissue artefact. For that purpose, several authors recommend the use of multibody kinematics optimization (MKO) (Naaim et al., Citation2017) but the way to adapt the generic model to the subject (scaling) is not as trivial as it can appear (Duprey et al., Citation2017). In the description of the upper limbs, the main challenge is to accurately model the shoulder complex, including sternoclavicular, scapulothoracic, acromioclavicular and glenohumeral joints. In the field of MWC propulsion research, several upper extremity models have been used. The simplest had modelled the shoulder complex as a ball joint between the thorax and the humerus located on the glenoid centre. A more complex model implemented regression equations allowing sternoclavicular and scapulothoracic coupled coordinates (Holzbaur et al., Citation2005). Finally, others authors had proposed to guide the motion of the scapula on an ellipsoid to represent the scapulothoracic joint (Van der Helm Citation1994; Seth et al., Citation2016). However, the personalization of the ellipsoid parameters is not obvious.
The aim of this work was to evaluate the influence of the ellipsoid parameters (centre location and radii) on kinematics reconstructed using MKO during MWC propulsion.
2. Methods
2.1 Experimental data
One able-bodied subject (22 y.o, 1.75m, 62 kg) participated in this preliminary study. During the experiment, the subject was asked to perform several propulsion cycles on a MWC ergometer. 36 reflective markers were placed on the upper limbs, and their 3D locations were recorded at 100 Hz by an 8-cameras optoelectronic motion capture system (Vicon system, hardware: T40 cameras; Nexus software; Oxford Metrics, UK).
2.2 Musculoskeletal model
A three-dimensional linked-segment model of the upper limbs was used to compute biomechanical parameters. The model included hand, forearm bones, humerus, clavicle, scapula and thorax. The latter were derived from the generic scapulothoracic joint model (Seth et al., Citation2016), which was symmetrised using a custom routine. This model was scaled to the subject using the algorithm implemented in OpenSim software (Delp et al., Citation2007), and the location of the acromio-clavicular contact point was scaled with a custom program.
2.3 Data processing
Joint kinematics was obtained for a steady-state propulsion cycle, using an inverse kinematics algorithm implemented in OpenSim software. The impact of the estimation of scapulothoracic joint parameters was evaluated with a full factorial design with 6 parameters on two levels. Parameters were the 3 radii of the ellipsoid and the 3 coordinates of its centre in the thorax frame; and were alternately defined at 80% and 120% of the initial values obtained from the default scaling step.
For each of the 64 resulting models, the overall root mean squared error (RMSE) between reconstructed and experimental markers was computed, and compared to the value obtained with the initial default scaled model.
3. Results and discussion
The results showed that among the 64 models, only eight induced a lower error between the reconstructed and the experimental position of the scapula markers with respect to the default scaled model. As depicted in Figure , most of the modified models induced a lower RMSE during a propulsion cycle for the arm (number of such case: 34), forearm (33) and hand (51), reaching up to a 5% improved reconstruction for the arm. A lower number of markers improved the kinematics reconstruction of the thorax (12) and clavicle (25), but the clavicle markers reconstruction reached 9% of improvement depending on the ellipsoid parameters chosen. Among the combinations that enhanced the scapula markers, several ellipsoid parameters led to a better RMSE for multiple body segments, with two distinct situations: either improving thorax/scapula only, or improving all the segments except the thorax.
Figure 1. Distribution of the ratio between the markers RMSE obtained for the 64 models and with the default scaled model. Results were presented for each segment. Value lower than 1 means improvement through the modified model
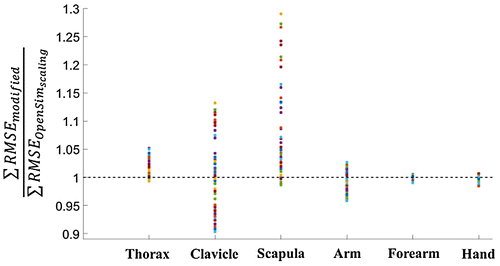
The impact of ellipsoid parameters on generalized coordinates is shown on Figure , for two selected degrees of freedom of the scapula, namely abduction and elevation. The ellipsoid parameters from the OpenSim default scaling (plain blue line) were obviously within the standard deviation of the 64 modified parameters (light blue space), whereas the parameters that optimized the global marker RMSE (red dashed line) displayed a different behaviour along the propulsion cycle.
Since many models resulted in higher RMSE than the default model (Figure ), it could be assumed that the default scaling method implemented in OpenSim provided a reasonable kinematics reconstruction. However, by slightly changing ellipsoid parameters, it was shown that RMSE could be reduced. With the hypothesis that a lower marker error denotes a better kinematic chain, this result implied that ellipsoid parameters are key elements that should be subject-specifically defined.
The differences in kinematics can be explained from geometric interpretation of ellipsoid parameters: the ellipsoid that minimized the global marker RMSE placed the scapulothoracic joint centre more downwards and backwards in the thorax, which explained why the resulting scapula elevation is higher (Figure ). Moreover, for some degrees of freedom, like scapula abduction, the standard deviation resulting from the different models was reduced around the middle of the propulsion cycle, whereas it increased at the beginning or the end of the propulsion cycle. This could be explained by the scapula motion that exhibit a lower amplitude in the middle of the propulsion cycle, while it reaches greater angles when the arm moves backwards to allow the rim grasp.
4. Conclusions
A full factorial design showed that scapular ellipsoid parameters can result in significant improvement in the kinematics reconstruction during MWC propulsion. In this perspective, subject-specific imaging methods coupled to optimization algorithms could be of great interest. This study could also be extended with the clavicle length which was already showed as critical (Duprey et al., Citation2017).
References
- Delp SL, Anderson FC, Arnold AS, Loan P, Habib A, John CT, Guendelman E, Thelen DG. 2007. OpenSim: Open-Source Software to Create and Analyze Dynamic Simulations of Movement. IEEE Transactions on Bio-Medical Engineering. 54(11):1940–50.
- Duprey S, Naaim A, Moissenet F, Begon M, Chèze L. 2017. Kinematic Models of the Upper Limb Joints for Multibody Kinematics Optimisation: An Overview. Journal of Biomechanics.
- Holzbaur KRS, Murray WM, Delp SL. 2005. A Model of the Upper Extremity for Simulating Musculoskeletal Surgery and Analyzing Neuromuscular Control. Annals of Biomedical Engineering. 33(6):829–40.
- Naaim A, Moissenet F, Duprey S, Begon M, Chèze L. 2017. Effect of Various Upper Limb Multibody Models on Soft Tissue Artefact Correction: A Case Study. Journal of Biomechanics.
- Seth A, Matias R, Veloso AP, Delp SL. 2016. A Biomechanical Model of the Scapulothoracic Joint to Accurately Capture Scapular Kinematics during Shoulder Movements. PLOS ONE. 11(1).
- Van der Helm FCT. 1994. A Finite Element Musculoskeletal Model of the Shoulder Mechanism. Journal of Biomechanics. 27(5):551–555.