1. Introduction
Rowing is a very physical activity requiring full range of joint motion associate with the generation of large forces at the feet and hands. The low back joints act to transfer these forces between the upper and lower extremities. As a result, low back injuries are the most common injuries observed in rowing, comprising 25–45% of the reported injuries requiring a medical attention (Teitz et al. Citation2001).
Although many studies have been conducted on low back injuries in rowing during the last decades, only few clinical implications for the practitioners have been published until now. Like recent studies focused on asymmetry (e.g. Fohanno et al. Citation2015), the number and complexity of the structures involved make difficult to establish easy-to-use indexes that would help practitioners to prevent rowers from low back injuries. The estimation of low back muscle kinematics may help to better understand the mechanisms that lead to low back injuries. This approach, however, requires (i) adapting prior musculoskeletal models published in gait analysis to both rowing specificities and (ii) estimating the low back muscles maximum passive length to be used as the reference. This study aimed at assessing low back muscles kinematics during rowing. We hypothesized that the maximum lengths reached by the low back muscles during the rowing cycle are close to their respective maximum passive lengths and individual technique leads to differences between rowers.
2. Methods
A generic OpenSim musculoskeletal model (12 segments, 13 joints, 128 muscles) allowing the full range of joint movement of the lower-limb and trunk segments has been adapted from prior models developed in the litterature (Arnold et al. Citation2010; Raabe et Chaudhari, Citation2016). Note that this model included 4, 5, 7 and 20 heads for ilio-costalis, longissimus thoracis, quadratus lomborum and multifidus muscles, respectively, with respect to their proximal (pelvis or sacrum) and distal insertions (5 lumbar vertebrae). Each muscle lever arm of the model has been cautionnaly checked on the full range of joint movement and adjusted with respect to the data published in the litterature when discontinuities were observed.
Two males and one female (age: 18/24/18 years; mass: 83/70/60 kg; height: 1.78/1.74/1.70 m) national rowers were equipped with 66 reflective markers (pelvis (8), thorax (5), head (3), thighs (5 × 2), shanks (6 × 2), feet (6 × 2), upper arms (4 × 2), forearms (3 × 2) and hands (1 × 2)). The marker three-dimensional trajectories were recorded using a 20-camera motion analysis system sampling at 100 Hz (Vicon, UK).
The participants were recorded in a static anatomical position to scale the generic musculoskeletal model to the subject’s anthropometry. The joint centres were located by a fonctional algorithm using setup movements as performed in Fohanno et al. (Citation2014). The maximum passive lengths of 10 muscles were extracted from 3 static streching tests: sit and reach test (SRT), crouched test (CT) for the low back muscles, and passive straight leg raise test (PSLR) for hamstrings.
After a routine warm-up, participants were tested for 30 seconds at race pace on a Concept2 Dynamic Indoor Rower (Concept2, USA). Ten consecutive rowing cycles were selected and time-normalised [0, 100%] in the middle of the acquisition when the cycle length was stabilised. Muscle kinematics was normalised using the maximum passive length as the reference and then time-averaged for the 10 cycles.
3. Results and discussion
Comparison of low back muscle length profiles for the whole rowing cycle showed similar patterns between the 3 participants (see Figure as an example for left longissimus thoracis (head inserted on lumbar vertebrae 1)). The variations of length of the low back muscles were lower than 25% with respect to their maximum passive lengths. The highest values were reached at the catch of the rowing cycle while the lowest values were observed at the release. The maximal values were closed to 100%. Differences were observed, however, between the 3 participants at the catch. As an example, the maximal value of the left longissimus thoracis was 99%, 101% and 96% for participants 1, 2 and 3, respectively. The increase in length to a value close to the maximum, when the rower reverses its movement and the back muscles are strongly activated, is a situation at risk for the low back joints.
Figure 2. Left longissimus thoracis length pattern. The standard deviation interval (dotted lines) is shown only for participant 3 to improve clarity.
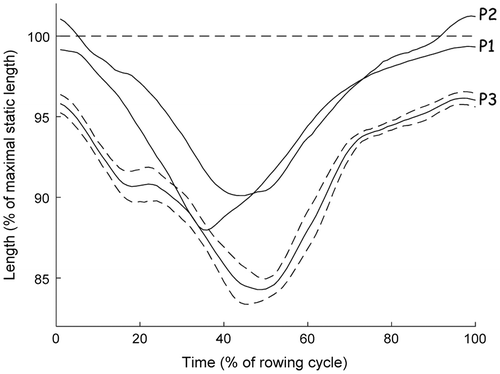
These values close or above 100% raise the question of the validity of the passive tests used to estimate the low back muscles maximum passive length. On one hand, the reference test published in the literature, SRT, showed a maximal lumbar flexion for only participant 3 (Table ). The lumbar flexion was maximal for participants 1 and 2 during the CT, a test not referenced in the literature. They reached respectively 89% and 95% of this maximal flexion during the SRT. On the other hand, hamstring lengths were maximal for participant 3 during SRT while these values were between 96% and 99% for participants 1 and 2.
Table 1. Flexion angles and muscle lengths from SRT and CT tests. Max is the maximum value including the PSLR test.
These results show that SRT is not well appropriate to estimate the low back muscles maximum passive length. They suggest the role of hamstrings while estimating this passive length. If they are fully stretched, 100% of the length extracted from PSLR, they limited the pelvis anteversion and the global flexion is mainly performed by the low back joints (participant 3). If they are not fully stretched as observed for participants 1 and 2, the global flexion is the result of the pelvis anteversion and then of low back joints. As a result, the low back muscles are not fully stretched. The CT is not universal test as shown by participant 3 who reached only 89% of his maximal lumbar flexion. Anthropometric characteristics, number of joints involved associated to the difficulty to maintain balance in this position are clear limitations for the reproducibility of this test.
4. Conclusions
Kinematics of the low back muscles was investigated in national rowers when rowing on a mobile ergometer at race pace. The results showed that the highest values were close to the reference extracted from passive tests and were subject-dependant. They highlighted that the passive test must evolve in order to be more specific to the target muscle. Further studies should be realised to confirm these promising findings and provide useful information to clinical practitioners for minimising rowing injuries.
References
- Arnold EM, Ward SR, Lieber RL, Delp SL. 2010. A model of the lower limb for analysis of human movement. Ann Biomed Eng. 38:269–279.10.1007/s10439-009-9852-5
- Fohanno V, Nordez A, Smith R, Colloud F. 2014. Estimating joint kinematics of a whole body chain model with closed-loop constraints. Multibody Syst Dyn. 31:433–449.10.1007/s11044-013-9366-7
- Fohanno V, Nordez A, Smith R, Colloud F. 2015. Asymmetry in elite rowers: effect of ergometer design and stroke rate. Sports Biomech. 14:310–22.10.1080/14763141.2015.1060252
- Raabe ME, Chaudhari A. 2016. An investigation of jogging biomechanics using the full-body lumbar spine model: Model development and validation. J Biomech. 49:1238–1243.10.1016/j.jbiomech.2016.02.046
- Teitz CC, O’Kane JW, Lind BK. 2003. Back pain in former intercollegiate rowers. A long-term follow-up study. Am J Sports Med. 31:590–595.10.1177/03635465030310041901