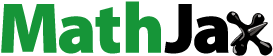
1. Introduction
Dental decays are one of the most widespread surgeries over the world. But half of the operation’s deals with the repair of a former restoration instead of curing an original decay. To reduce this problem, new dental composites are being developed, especially bulk materials with a higher depth of cure (5 mm). To speed up the process of development, it may be interesting to develop numerical models that can predict the mechanical behaviour of restored teeth and account for their microstructure.
Such models would require both macroscopic experimental tests to be validated, and microscopic parameters for simulations. In addition to the fillers properties and proportion, the behaviour of the interface between the matrix and the fillers is indeed a key linker point. The aim of this study is to propose an easy way to evaluate the interface quality from macroscopic tests, easier to perform than microscopic ones. In particular, the three-point bending test is the most common standard in dental materials studies. Turcsányi et al. (Citation1988) proposed a universal model to predict the yield stress of non-dental composites, knowing their filler ratio and the matrix yield stress. However, its validity has not been proven for filler ratios higher than 40 vol%, whereas dental composites contains more than 50 vol% of fillers. Moreover, the model was based on tensile tests and it was not proven that bending tests can lead to successful results. This is what we propose to check hereafter.
2. Methods
2.1. The Turcsányi model
Turcsányi et al. (Citation1988) established a relationship between the yield stress of a composite the yield stress of its matrix
and the filler volume ratio
(1)
(1)
(2)
(2)
A represents the packing of the fillers. It is the matrix content of the most filled section of the sample, with respect to its global volume ratio. The exponential part corresponds to an empirical result obtained from 25 different non-dental composites tested by Turcsányi. It appeared that the parameter B does not depend neither on the shape of the fillers, nor their size, nor their composition, nor the composition of the matrix. It only reflects the nature of the interface between the fillers and the matrix (Turcsányi et al. Citation1988). Low values of B (lower than 3) correspond to weak interfaces. Strong interfaces reach values from 3 to 6 (Liang and Li Citation1998; Saen et al. Citation2016).
2.2. Materials
An experimental material produced by DMG (Hamburg, Germany) was used for this study. The matrix is composed of bis-GMA, TEGDMA, UDMA and EBPADMA. Fillers are mostly random-shaped silanated barium glass particles, with an average size of 7 µm. Five mixtures of this material were prepared by the Laboratory of Multimaterials and Interfaces (Lyon, France), containing respectively 0 wt% (no filler), 20 wt%, 40 wt%, 60 wt% and 80 wt% of fillers. Samples were then made in a Teflon mould of 25 × 2 × 2 mm3, in a darkroom to avoid undesired polymerization. The curing was activated by bluelight with a polymerization lamp at 1470 mW/cm2. Both bottom and top sides were exposed 40 s from the top and then from the bottom. Finally, samples were stored 24 h in distilled water at 37 °C to complete the polymerization.
2.3. Bending test
The three-point bending tests were performed with a ZwickRoell ProLine static machine (BZ 2,5/TH1S, Ulm, Germany) according to the recommendations of the ISO 4049 norm. The two cylindrical supports are spaced 20 mm apart. The cross-head speed is 0.75 mm/min. The flexural strength and the strain under the loading roller were calculated by:
(3)
(3)
(4)
(4)
where F is the applied load, L the distance between the supports, b and h the width and the thickness of the sample respectively, and D the maximum deflection at the center of the beam. The yield stress was calculated from these formulas by the classic 0.2%–strain criterion. Fracture surfaces were finally examined with SEM after the samples were broken.
3. Results and discussion
The results of the tests are presented in the :
Table 1. Mechanical properties of resin composites measured using three-point bending test.
To determine the B value of the Turcsányi model, the evolution of the composite yield stress (Re(X%)) over the yield stress of the matrix (Re(0%)) was plotted with respect to the filler volume ratio (). The parameter B was calculated for the full data and for the limited [0; 60] wt% range, excluding the highest filler ratio.
Figure 1. Evolution of the yield stress of composite resins with respect to their filler volume ratio.
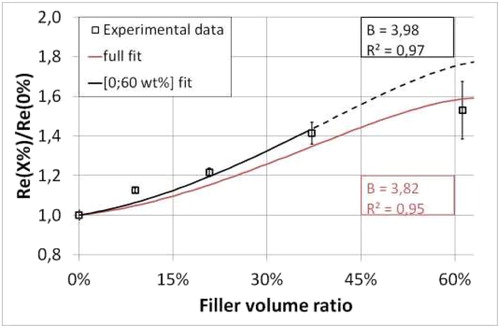
Coefficients of determination between experimental data and the model are good: higher than 0.95. This means that the Turcsányi model suits well dental composites tested by three-point bending test. It also confirms that, locally, the area which plasticizes first undergoes tensile loads. However, the fit is better without taking into account the most filled composite. Indeed, the yield stress for the 80 wt% compound may be underestimated as the samples broke before reaching plasticity. In these conditions, the yield stress is equal to the flexural strength. The 0 wt% value may be underestimated too. Indeed, these samples deformed much more than for the other ratios, and broke into three pieces instead of two. This proved that the hypothesis of the beam theory which led to formulae (2) and (3) were not fully satisfied. If biases are artificially removed from the , we can easily intuit an even better fit between the results and the model.
So far, the parameter B is estimated close to 4. This value corresponds to a good interface, which was expected because of the fillers silanization treatment.
SEM observations validate this strong interface (): particles as small as few micrometres can be seen on each side of the fracture area. This means that it costs less energy to propagate the cracks through the barium glass than bypassing the particle along its interface with the matrix. If it was not the case, a lot of particle footprints should be observed on opposite faces. These results showed that energy is well transmitted to the fillers by the interface.
4. Conclusions
These preliminary results are promising. Even if some uncertainties exist for unfilled and very high-filled composites, the Turcsányi model seems to be transposable to the common three-point bending test and to high-filled dental composites. The prediction of both yield stress and interface quality from a single macroscopic test could be of great help in the development of new dental composites. These data are also very important to validate numerical models.
Acknowledgements
Two other laboratories are involved, the LMI and MATEIS, which are acknowledged for their help in the preparation of the materials. DMG© is also acknowledged for having provided the raw materials and for their availability.
Additional information
Funding
References
- Liang J, Li R. 1998. Prediction of tensile yield strength of rigid inorganic particulate filled thermoplastic composites. J Mater Proc Technol. 83(1–3):127–130.
- Saen P, Atai M, Nodehi A, Solhi L. 2016. Physical characterization of unfilled and nanofilled dental resins: static versus dynamic mechanical properties. Dent Mat. 32(8):e185–e197.
- Turcsányi B, Pukánszky B, Tüdõs F. 1988. Composition dependence of tensile yield stress in filled polymers. J Mater Sci Lett. 7(2):160–162.