Keywords:
1. Introduction
Knee injury is common during sports activities such as basketball, football, volleyball or skiing (De Loës Citation2000). Knee braces seem to be a solution to prevent this kind of injury. Some in vitro studies have already been conveyed to evaluate knee braces (Bakker et al. Citation2016). However, these works are limited by the quality of tissues and the poor reproducibility of tests. An alternative method to overcome these limitations is to use a knee surrogate. A substitute allows to perform easily reproducible mechanical tests using knee braces. In the literature, many substitutes have been developed allowing the evaluation of medical knee orthoses (Cawley et al. Citation1989) or specific braces for sports equipment protection, for football (France et al. Citation1987) or skiing (Nusser et al. Citation2016) for instance. However the validation of these functional prototypes is in most cases specific to one application.
Based on these studies, a surrogate knee has been developed in our laboratory. Anterior drawer and varus valgus tests, performed in vitro in the literature, were reproduced on the surrogate to evaluate ligament laxity and quantify the effect of a knee brace.
2. Methods
2.1. Knee surrogate
An anatomical model was built based on CT scans and bi-planar radiographic acquisitions of the right lower limb from an asymptomatic male subject (25 years old, 1.81 m, 78 kg). This model allowed to extract the bones geometry, the location of the ligaments’ insertions and the external body shapes. The anatomy of the distal and proximal bone parts (femur and tibia) were extracted and 3D printed in Polylactic Acid (PLA) and fixed to aluminum profiles. For the knee surface articulation: a femoral implant Hermec 2C (Ceraver) was used. A 3D printed tibial plateau was fixed in order to ensure the rotational guidance in flexion extension (). The four main ligaments (cruciate and collateral) were represented by a cable in series with a spring to reproduce their non-linear behavior. The initial stroke was adjustable with a screw.
A polyurethane thermoformed sleeve, representing the skin, was linked to the aluminum profiles via 3D printed PLA supports.
2.2. Kinematic evaluation and passive tests
Kinematics were measured through motion capture with Polaris® (NDI measurement). Two tripods were fixed on the aluminum profiles, one for the femur and one for the tibia. The positions of technical frames compared to anatomical frames were previously registered using the EOS system. The passive kinematics during the drawer and varus-valgus tests were based on in vitro tests from Noé and Poirier (Citation2008) at 30° of knee flexion.
The varus-valgus angle was computed with the mobile sequence axes xyz from the angular position matrix of the tibia with respect to the femur in the tibia anatomical frame.
The force applied during each test was measured by a uniaxial S-type load cell (3138). For the drawer test, a quasi-static loading from 0 to 150 N was applied on the tibial tubercle, perpendicularly to the tibial axis. For the varus-valgus test, successive loads from 0 to 40 N and −40 N to 0 N were applied on the distal third lower part of the tibia, representing a 15Nm moment at the knee.
2.3. Ligaments configurations and knee brace
The ligaments of the surrogate were adjusted to replicate in vitro behavior quantified by Noé and Poirier (Citation2008). The anterior cruciate ligament (ACL) was adjusted to reproduce the behavior of an intact and a damaged ACL. Collateral ligaments were set to replicate the varus valgus behavior.
The influence of a knee brace (STRONG 700, TARMAK) was then evaluated, based on passive knee kinematics using the intact or damaged ACL configurations.
3. Results and discussion
For the surrogate limb, the anterior translation curve displayed a non-linear behavior due to the spring in series with the cable, representing ligaments ().
Figure 2. The anterior translation behavior of the surrogate limb under 150 N loading for various configurations.
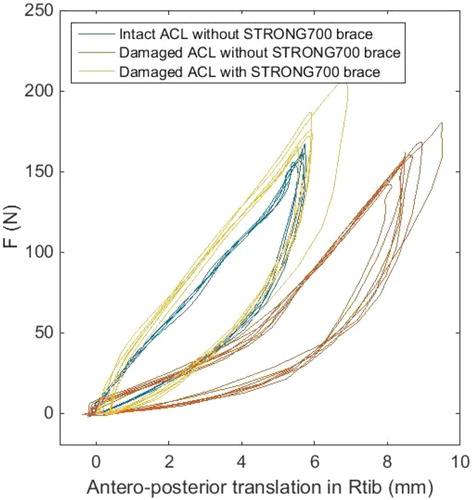
Amplitudes obtained with the surrogate were compared to the mean results of Noé and Poirier (Citation2008) in .
Table 1. The surrogate’s amplitudes in different configurations.
Concerning the effect of the knee brace, the anterior translation of the surrogate in the damaged ACL configuration equipped with the knee brace was reduced by 60% during the anterior drawer test compared to the surrogate in the same configuration without the knee brace ().
4. Conclusions
This study led to the design of a knee surrogate presenting ligament laxity in agreement with in vitro data for anterior drawer and varus valgus tests. A first sensitivity evaluation was conducted to evaluate if the surrogate was able to quantify the effect of a knee brace.
Compared to in vitro evaluation, the surrogate is reproducible and can be adjusted to various ligament laxities. The main limitation in this passive evaluation is that it does not take into account muscle activity. Recent work from Bakker et al. (Citation2016) proposed to combine in vitro evaluation with in vivo and in sillico determination of muscular activity. This approach can be adapted to be used with a surrogate knee to allow a parametric evaluation of knee braces.
Acknowledgements
The authors are grateful to DECATHLON for providing the knee braces and for supporting this study.
References
- Bakker R, et al. 2016. Effect of sagittal plane mechanics on ACL strain during jump landing. Wiley Online Library, p. 1636–1644.
- Cawley PW, France EP, Paulos E. 1989. Comparison of rehabilitative knee braces. Am J Sports Med. 17(2):141–146.
- De Loës M. 2000. A 7-year study on risks and costs of knee injuries in male and female youth participants in 12 sports. Scand J Med Sci Sports. 10:90–97.
- France EP, Paulos LE, Jayaraman G, Rosenberg TD. 1987. The biomechanics of lateral knee bracing Part II: impact response of the braced knee. Am J Sports Med. 15(5):430–438.
- Noé and Poirier. 2008. Mise au point d’un banc d’évaluation de la laxité ligamentaire du genou : Etude préliminaire ex vivo [Dissertation]. Paris: ENSAM.
- Nusser M, Hermann A, Senner V. 2016. Artificial knee joint and ski load simulator for the evaluation of knee braces and ski bindings. Proc Eng. 147:220–227.