1. Introduction
Abdominal blunt injuries occur in 9% of road traffic accidents and are responsible of a significant overmortality (Cheynel et al. Citation2011). About the third most commonly injured organ in blunt trauma is the small bowel injury (SBI). The difficulty of the diagnosis and delays in accessing care induces a high mortality rate (19–29%) (Fakhry et al. Citation2003). A common injury defined as SBI is the perforation which however the injury mechanism and injury threshold are unclear.
Bourgouin et al. described human small bowel behaviour under dynamic tensile conditions (Bourgouin et al. Citation2012). Previous studies focused also on digestive tract characterization under hyperpressure conditions, mainly on animal tissue and under physiological conditions (Stavropoulou et al. Citation2009; Sommer et al. Citation2013; Sokolis Citation2017). The aim of our study is to evaluate human small bowel behavior under static inflation conditions.
2. Methods
Tests were conducted on fresh human tissue. Small bowel procurement was obtained as part of the multiorgan retrieval procedure in accordance with the French Agency of Biomedecine. The protocol was approved by the agency’s scientific council. After sampling, small bowel was preserved in physiological saline solution at 4 °C prior to be tested within 6 hours. Experimental test () consists in insufflating of 10 cm small bowel segment under quasi-static (2.5 kPa/s) inflation until rupture. The technical testing allowed applying and controlling progressive intraluminal pressure into small bowel sample by filling with phosphate -buffered saline solution. Pressure was recorded with an analog pressure sensor. Bowel tissue strain was determined in three dimensions using high-speed cameras (Fastcam SA3, Photron, Tokyo, Japan) and Vic3D digital image correlation software (Correlated Solutions, Inc., Irmo, USA). Image correlation was applied on each image to define local displacement. Green-Lagrange major strain field (E1) was then computed by Vic 3 D software (http://correlatedsolutions.com/support/index.php?/K. nowledgebase/Article/View/2/0/strain-tensors-in-vic). The analysis focused on major strain (e1) versus pressure.
3. Results and discussion
A representative preliminary result of pressuremaximal deformation curve is given in . Pressure to ultimate failure was lower for jejunum compared to ileum. Pressure mean value at the failure was 19.8 ± 1.9 kPa for jejunum and 42.2 ± 6.4 kPa for ileum.
Figure 2. (A) Pressure-maximal deformation curve. (B) Example of Green-Lagrange strain fields in the principal direction E1.
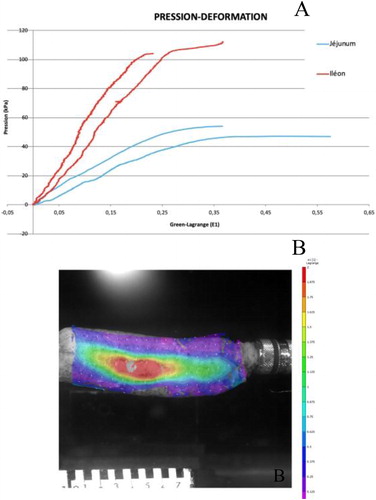
Displacement and Green-Lagrange strain fields were computed and an example of the major principal strain fields E1 for a sample is shown in . As expected strain fields show maximum strain concentrations around the rupture area just before failure. Concerning the failure area, samples systematically failed on the mesenteric side suggesting a greater fragility of this part of small bowel.
The biomechanical difference between jejunum and ileum was previously reported in rat by Dou et al. (Dou et al. Citation2003). It could be explained by layer anatomicalcharacteristics and physiological differences, mucosa and sub-mucosa of jejunum being more developed to allow absorption and ileum acting as a reservoir.
4. Conclusions
The experimental setup proposed in this study allows applying and control intraluminal pressure in small bowel sample in view to determine small bowel wall behavior and provides a realistic idea of the effective strain state of the sample according to the intraluminal pressure.
Acknowledgements
Authors would thank Max PY for his help in the experimental campaign.
References
- Bourgouin S, Bège T, Masson C, Arnoux P-J, Mancini J, Garcia S, Brunet C, Berdah SV. 2012. Biomechanical characterisation of fresh and cadaverous human small intestine: applications for abdominal trauma. Med Biol Eng Comput. 50(12):1279–1288.
- Cheynel N, Gentil J, Freitz M, Rat P, Ortega Deballon P, Bonithon Kopp C. 2011. Abdominal and pelvic injuries caused by road traffic accidents: characteristics and outcomes in a French cohort of 2,009 casualties. World J Surg. 35(7):1621–1625.
- Dou Y, Zhao J, Gregersen H. 2003. Morphology and stress-strain properties along the small intestine in the rat. J Biomech Eng. 125(2):266–273.
- Fakhry SM, Watts DD, Luchette FA, EAST MultiInstitutional Hollow Viscus Injury Research Group 2003. Current diagnostic approaches lack sensitivity in the diagnosis of perforated blunt small bowel injury: analysis from 275,557 trauma admissions from the EAST multi-institutional HVI trial. J Trauma. 54(2):295–306.
- Sokolis DP. 2017. Experimental study and biomechanical characterization for the passive small intestine: Identification of regional differences. J Mech Behav Biomed Mater. 74:93–105.
- Sommer G, Schriefl A, Zeindlinger G, Katzensteiner A, Ainödhofer H, Saxena A, Holzapfel GA. 2013. Multiaxial mechanical response and constitutive modeling of esophageal tissues: Impact on esophageal tissue engineering. Acta Biomater. 9(12):9379–9391.
- Stavropoulou EA, Dafalias YF, Sokolis DP. 2009. Biomechanical and histological characteristics of passive esophagus experimental investigation and comparative constitutive modeling. J Biomech. 42(16):2654–2663.