1. Introduction
Inhibition of TIEG1 gene provides changes within the musculoskeletal system. Recently, hypertrophy, hyperplasia and a metabolic glycolytic switch for slow and fast fibers has been observed in TIEG1 KO (knock- out) muscle (Kammoun et al. Citation2016). Previously, mechanical tests have been performed on single muscle and tendon fibers using stretch and relaxation tests in the direction of the main fiber filaments (Bensamoun et al. Citation2006; Kammoun et al. Citation2016). The functional properties of TIEG1 KO muscle fibers showed mechanical changes compared to WT (wild type). Thus, the objective is to complete these characterizations by applying transversal indentations to single muscle and tendon fibers using a new experimental protocol adapted to atomic force microscopy (AFM) (Kammoun et al. Citation2019).
2. Methods
All experiments were performed on a commercial AFM (NanoWizard 3, JPK Instruments, Berlin, Germany) combined with an inverted optical microscope (AxioObserver.Z1, Zeiss) driven by JPK NanoWizard software 6.0 and Zen Blue 2012. Data were acquired in Quantitative Imaging (QI) mode using PFQNM-LC cantilevers (Bruker, Santa Barbara, CA, USA). Cantilever spring constant and sensitivity were calibrated before each experiment using the thermal fluctuation method of the JPK software, yielding spring constant values ranging from 0.019 to 0.025 N/m.
Control (NWT_Tendon = 7, NWT_Soleus = 7, NWT_EDL = 7) and TIEG1 KO (NKO_Tendon = 7, NKO_Soleus = 7, NKO_EDL=7) fibers were extracted from slow (soleus: SOL) and fast (EDL: extensor digitorium longus) muscles as well as tail tendon.
Perfect immobilization of the sample is a prerequisite during each AFM experiment. This is particularly the case in experiments conducted on fibers where the slightest movement (vertical or lateral) is likely to generate artifacts during the acquisition of force curves, which will result in making the elasticity maps unusable. The absence of movement during transverse indentations is therefore a fundamental element of our experimental protocol. Fibers were immobilized on plexiglass, without any chemical agent or mechanical fixation, and submersed in a drop of PBS. Bright field imaging was used to identify the area of interest on fibers.
A visual control of the immobility of the fiber was performed using the optical microscope coupled with the AFM instrument. This ensured that only Quantitative Imaging (QI) files were recorded in a static condition. Subsequently, the AFM tip was engaged in the central portion of the fiber (to ensure a near plane contact surface to avoid curvature effects leading to artifacts) to scan an area of 15 µm × 15 µm. The AFM tip was scanned on three areas along each muscle and tendon fibers by applying a force trigger of 2 nN with a 3 µm Z ramp.
3. Results and discussion
The sarcomeric structure of muscle fibers was observed with this new experimental AFM protocol demonstrating its capability to measure the transversal elasticity of single muscle fibers (). The Young’s modulus was significantly decreased for TIEG1 KO muscle fibers isolated from both the soleus and the EDL muscles compared to WT fibers. This decrease in the Young’s modulus is represented by less intense color mapping for the TIEG1 KO fibers (). These analyses also revealed that slow twitch fibers isolated from WT soleus muscle have greater elasticity than fast twitch fibers isolated from WT EDL muscle (). Conversely, TIEG1 KO tendon fibers exhibited a significant increase in the Young’s modulus compared to WT tendon fibers (; ). The Young’s modulus of the WT tendon (53.2 kPa) and WT EDL (46.5 kPa) were nearly identical indicating a similar range of the transversal elastic modulus for these two tissues. Finally, these results indicate that the impact of TIEG1 expression on elasticity is tissue specific.
Figure 1. Elasticity maps (15 x 15 mm) obtained from the AFM protocol for slow (soleus, Sol: A-B) and fast (EDL: C-D) twitch muscle and tendon (E-F) of WT and TIEG1 KO fibers. The muscle and tendon fibers cartographies are represented by different colors which are representative of lower (blue/green) and higher (red/orange) elasticities.
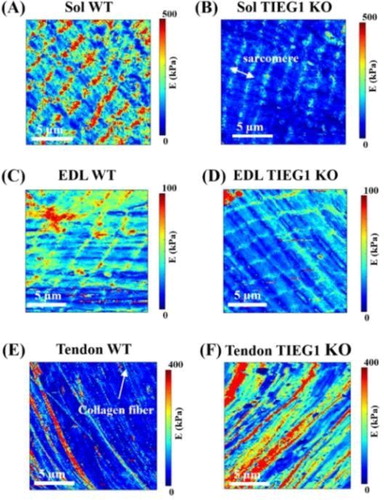
Figure 2. Quantification of Young modulus (E) obtained via AFM for WT (NWT_Tendon = 7, NWT_SOL = 7, NWT_EDL = 7) and TIEG1 KO (NKO_Tendon = 7, NKO_SOL = 7, NKO_EDL = 7) fibers. ***p < 0.001 between indicated groups.
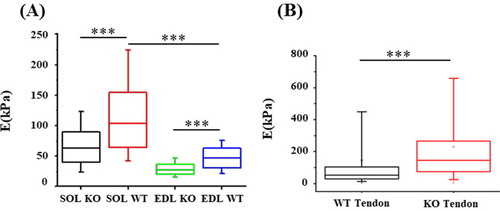
The AFM technique, which is primarily used to image molecular and cellular systems, is currently poorly utilized for analysis of mechanical properties in fibrous tissues. Past studies have employed AFM on muscle tissue cryosections (Marturano et al. Citation2013) or following tendon fixation (Rigozzi et al. Citation2013) at resolutions of the whole tendon (diameter units: millimeter) or at the tendon fibril level (diameter units: nanometer). For the first time, we report the development of a novel protocol for the use of AFM on living (i.e. without treatment, fixation …) muscle and tendon tissues.
However, it is difficult to compare the elastic values measured with AFM to other studies due to the experimental conditions such as preparation and fixation of the sample and type of cantilever used.
4. Conclusions
These results demonstrate that the efficiency of the AFM protocols (experimental and acquisition) are representative of the main structures of muscle and tendon fibers with regard to the elastic cartography allowing for the avoidance of topographic acquisition that is currently performed with AFM.
Additional information
Funding
References
- Bensamoun SF, Tsubone T, Subramaniam M, Hawse JR, Boumediene E, Spelsberg TC, An KN, Amadio PC. 2006. Age-dependent changes in the mechanical properties of tail tendons in TGF-beta inducible early gene-1 knockout mice. J Appl Physiol. 101(5):1419–1424.
- Kammoun M, Meme S, Meme W, Subramaniam M, Hawse JR, Canon F, Bensamoun SF. 2016. Impact of TIEG1 on the structural properties of fast and slow twitch skeletal muscle. Muscle Nerve. 55(3):410–416.
- Kammoun M, Pouletaut P, Canon F, Subramaniam M, Hawse JR, Vayssade M, Bensamoun SF. 2016. Impact of TIEG1 deletion on the passive mechanical properties of fast and slow twitch skeletal muscles in female mice. PloS One. 11(10):e0164566.
- Kammoun M, Ternifi R, Dupres V, Pouletaut P, Meme S, Meme W, Szeremeta F, Landoulsi J, Constans JM, Lafont F, et al. 2019. Development of a novel multiphysical approach for the characterization of mechanical properties of musculotendinous tissues. Sci Rep. 9(1):7733.
- Marturano JE, Arena JD, Schiller ZA, Georgakoudi I, Kuo CK. 2013. Characterization of mechanical and biochemical properties of developing embryonic tendon. Proc Natl Acad Sci USA. 110(16):6370–6375.
- Rigozzi S, Muller R, Stemmer A, Snedeker JG. 2013. Tendon glycosaminoglycan proteoglycan sidechains promote collagen fibril sliding-AFM observations at the nanoscale. J Biomech. 46(4):813–818.