Keywords:
1. Introduction
Kong-vault is a parkour technique commonly used by practitioners, called ‘traceurs’, to overcome obstacles in urban environments, like large barriers, rails and fences using support from the hands (). In gymnastics, jump techniques using hand support could be found when using pommel horses. They have been extensively analyzed in the literature. However, parkour vaulting techniques are still poorly understood and strategies coming from gymnastics cannot be directly extrapolated to parkour. In fact, the differences in environmental constrains could induce a different vaulting strategy (Irwin and Kerwin Citation2009; Heinen et al. Citation2013). While, in gymnastics, vaulting techniques are executed in controlled environments using springboards with cushioned tables and mats, in parkour, these techniques are performed in changing environments with hard surfaces. In this work, we present for the first time a biomechanical study of the take-off phase of the parkour kong-vault technique through a kinematics, kinetics and inverse dynamics analyses of the movement ().
2. Methods
2.1. Participants
Eleven healthy trained male traceurs (age: 22 ± 3.5 years, height: 1.72 ± 0.06 m, mass: 67 ± 7 kg) volunteered for the study. The traceurs’ experience in parkour was 5.5 + 2.3 years and their weekly conditioning was of 5 ± 2 hours. The participant exclusion criterion was based on history of lower extremity injuries or diseases that might affect kong-vault biomechanics. The experiments were conducted in accordance with the standards of the Declaration of Helsinki (rev. 2013) and approved by a local ethics committee.
2.2. Experimental protocol
A parkour tubular structure was assembled and adapted to the anthropometry of the volunteers. Participants performed a 5 min warming up session followed by self-stretching and a familiarization period. Traceurs were instructed to perform the kong-vault technique from an initial landmark on the floor, placed at a horizontal distance equal to half their height from the vertical projection of the handlebars. They were asked to land on a target landmark symmetrically positioned with respect to the vertical of the handlebars ().
2.3. Data acquisition
A total of 4 successful repetitions per participant were recorded. 3D whole-body kinematic data were collected using 14 infrared cameras sampling at 400 Hz (Vicon, Oxford Metrics, Oxford, UK) and recording 48 reflective markers placed on the participant’s body according to the ISB recommendations. Two force plates (AMTI, Watertown, MA, USA) embedded into the floor and two rigid handlebar sensors (SENSIX, Poitiers, Vienne, France, Ø 63 mm) sampling at 2000 Hz and fixed on the Parkour tubular structure, were used to record external forces and moments applied on the participants.
2.4. Data analysis
The motion was divided into three phases: take-off, flight and landing. In this work we focused on the takeoff phase only. The beginning of the take-off phase was defined as the moment when the ground reaction vertical force was higher than the participant weight. The end of the take-off phase was defined at the instant just before the hands release the handlebars (time at which the force in the handlebars is less than 10 N). Scaling, inverse kinematics and inverse dynamics were computed using a custom-made Python program, a physics engine and a whole-body skeletal model.
3. Results and discussion
Results from inverse kinematics showed that the take-off motion starting from a static state (as in our study), can be divided into two main sub-phases (the left and right areas divided by the shadow in ). At the beginning, both feet and hands are kept in contact with the ground and handlebars respectively, while the lower and upper limbs are being extended. Then the feet leave the ground and the hands are kept in contact with the handlebars while the lower body is flexed to pass over the handlebars. This second sub-phase arises at around 40% of the total time of the take-off.
Figure 2. Inverse dynamic analysis of the take-off phase. The skeleton on the top represents the results from the inverse kinematics for 1%, 20%, 40%, 60%, 80% and 100% of the take-off phase. The torques and forces displayed below correspond to mean values for right limbs and sensors respectively. The shadow area divides the take-off phase into two sub-phases.
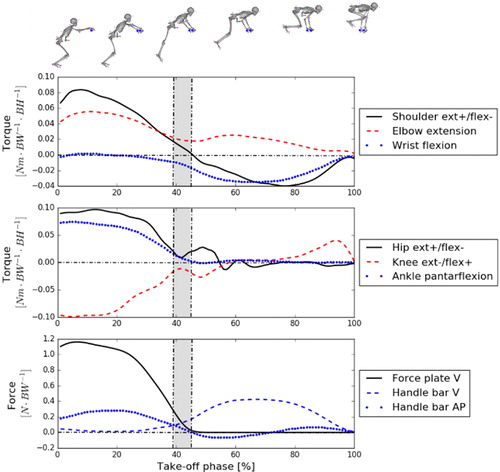
At the kinetic level, during the first sub-phase, the greatest part of the reaction forces is generated at the force plates (1.2 N.BW−1 vs. 0.3 N.BW−1 at the handlebars). As shown in , the antero-posterior component of the force generated at the handlebars is increased when the second sub-phase begins.
Besides, the inverse dynamics analysis showed that during the first sub-phase, the maximum torques developed at the ankle, the knee, the hip and the shoulder are equivalent and close to an absolute value of 0.09Nm.BW−1.BH−1. At the opposite, the wrist developed relatively low torque compared to other articulations. During the second sub-phase, because the feet left the ground, low joint torques were developed at the lower limbs (<0.04Nm.BW−1.BH−1). However, it is interesting to note that flexion/extension torques at the upper limbs also decrease during this sub-phase even though both hands are still in contact with the handlebars and in spite of the antero-posterior forces generated at the handlebars (0.4 N.BW−1).
4. Conclusions
During the take-off phase of the kong-vault technique, two sub-phases were observed. A first one involving both external contacts at the hands and the feet, and a second one where only the hands are in contact with the environment. The constraints observed at the joints reveal that both limbs developed their greater activity during the first sub-phase. After that, joint torques significantly decreased. Thus, it could be hypothesized that the total mechanical power is essentially developed during the first sub-phase at the upper and lower limbs level. During the second sub-phase, lower joint torques were observed. They might mostly contribute to accommodate the body posture for landing, and to regulate accurately the initial velocity and angle of the center of mass, which determines the flight phase trajectory of the traceur as previously observed in highly dynamic parkour jumps (Maldonado et al. Citation2018).
References
- Heinen T, Vinken PM, Jeraj D, Velentzas K. 2013. Movement regulation of handsprings on vault. Res Q Exerc Spor. 84(1):68–78.
- Irwin G, Kerwin D. 2009. The influence of the vaulting table on the handspring front somersault. Sports Biomech. 8(2):114–128.
- Maldonado G, Bailly F, Souères P, Watier B. 2018. On the coordination of highly dynamic human movements: an extension of the uncontrolled manifold approach applied to precision jump in parkour. Sci Rep. 8(1):12219.