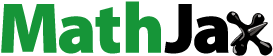
1. Introduction
Mathematical models are increasingly designed to guide experiments in biology, biotechnology, as well as to assist in medical decision making. We present a 3D high resolution Deformable Cell Model (DCM) integrating information from measurements in order to obtain a refined quantitative understanding of the cell mechanics. The showcase in this work is the regeneration of liver after drug-induced depletion of hepatocytes, in which surviving dividing and migrating hepatocytes must squeeze through a blood vessel network to fill the emerged lesions. Here, the cells’ response to mechanical stress might significantly impact on the regeneration process.
2. Methods
The model is a cell-based mathematical model, where the cell cortex-membrane structure is triangulated by nodes, endowing a deformable structure (Van Liedekerke et al. Citation2019), see . The movement of nodes is governed by an equation of motion of type:
Figure 1. Representation of the DCM (top). Simulation of cell growth, leading to cell division to yield a cell clump. During each cell division, the mother cell envelope is filled and replaced by two adhering daughter cells with half the volume of the mother cell (bottom).
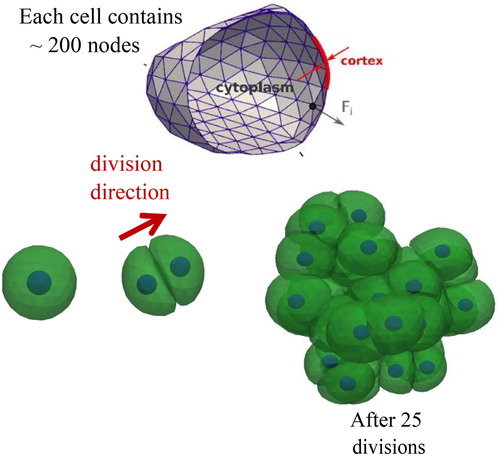
Where ζ is a friction matrix, V(x) is the velocity vector of the nodes x, and F(x) is the total force vector of the nodes. The friction matrix determines how much energy is dissipated in the system, while the force vector contains all contributions related to the internal structure of the cell as well as external forces. The internal force models are viscoelastic elements (e.g. type Kelvin-Voigt, Maxwell) for each node-node pair interaction, reflecting the viscoelastic properties of the cell cortex, the cytoplasm and the nucleus. External nodal forces originate from e.g. adhesion or migration models. The model can mimic active cell contraction, cell growth and division, and apoptosis. Interactions with media or substrates inducing cell migration can also be accounted for. Each cell can have a polarity that makes it possible to distinguish regions in the cell with different properties, or that can determine in which direction a cell divides or migrates. Interactions of cells with arbitrarily shaped structures such as blood vessels can be captured naturally ().
Figure 2. Top: Simulated optical stretcher experiment to determine force models for the viscoelastic behavior of the cell. Middle: Simulation of liver lobule regeneration Bottom: Simulated relative area of the necrotic lesion during the liver regeneration process, compared to data from Hoehme et al. (Citation2010).
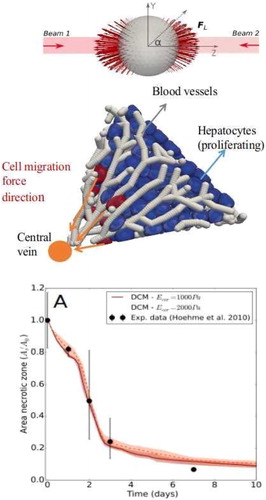
3. Results and discussion
For each cell, we must first infer the nature and model parameters of the mechanical elements from comparisons with optical stretcher experiments (see , top). In this experiment, the cells are deformed by forces upon interaction with a laser beam. A force-deformation plot can be extracted from these experiments.
With the so-calibrated model, we are able to perform multi-cellular simulations and validating them with in vivo data of a regenerating liver lobule as obtained in Hoehme et al. (Citation2010). The model predicts that next to cell growth and proliferation, typical migration forces of magnitude ∼0.1 nN are needed for a cell to exert on its environment in order move towards the central vein and to close a tissue lesion (Van Liedekerke et al. Citation2019).
4. Conclusions
We have constructed a generic high resolution computational model that can simulate cell mechanics and tissue mechanics with high accuracy. We have demonstrated that the model is capable of simulating complex tissue architecture as seen in liver lobules. Our results reveal that a realistic representation of cell shape is very important to quantify the mechanics in the tissue. We argue that this model type could become a future tool to perform realistic modeling for various mechano-biological questions (e.g. embryogenesis, mechano-transduction). We are currently embedding this model into our software platform called ‘TiSim’, which will be released as an integrated insight software for tissue simulations.
Acknowledgements
PVL and DD acknowledge support by BMBF - LiSym, INST.CANCER-PHYSCANCER, ITMO - INVADE, EU 7th Framework Programme (NOTOX), and ANR - iLite. DD gratefully acknowledges BMBF-VLN and Demonstrator Liversimulator. JN, SH and TJ gratefully acknowledge support by BMBF-LiSym, BMBF-VLN DFG-Emmy-Noether, and BMBF-Demonstrator Liversimulator.
References
- Hoehme S, Brulport M, Bauer A, Bedawy E, Schormann W, Hermes M, Puppe V, Gebhardt R, Zellmer S, Schwarz M, et al. 2010. Prediction and validation of cell alignment along microvessels as order principle to restore tissue architecture in liver regeneration. PNAS. 107(23):10371–10376.
- Van Liedekerke P, Neitsch J, Johann T, Warmt E, Gonzales Valverde I, Höhme S, Grosser S, Käs J, Drasdo D. 2019. Quantifying the mechanics and growth of cells and tissues in 3D using high resolution computational models. Available from: https://www.biorxiv.org/content/10.1101/470559v1.full.pdf.