1. Introduction
Eccentric (ECC) contraction corresponds to when an active muscle is stretched. This mode of contraction is commonly encountered in sports or occupational activities, for example when resisting or lowering a load or in the deceleration phase of throwing. ECC contractions have been associated with poor force control, variable motor output and with altered and variable movement kinematics. Actually, inhibitory and excitatory influences on the motoneurons and the control strategies associated with ECC contractions proved to be distinct from concentric (CON) or isometric contractions (Duchateau and Enoka Citation2008).
In particular, lowering a load, which involves mainly ECC contractions, has been reported as being more painful than raising it in individuals with impingement syndrome (Borstad and Ludewig Citation2002) suggesting that ECC contractions might bring specific biomechanical constraints to the shoulder complex. However, there is a scarcity of study having specifically investigated the differences in coordination of the shoulder complex muscles between movements involving CON and ECC contractions. In the present study we used the synergy analysis to investigate the muscle activation strategies associated with the tasks of lifting and lowering a loaded box with the arms, which involve mainly CON and ECC contractions, respectively.
2. Methods
2.1. Participants
Thirty subjects (16 men) aged of 20–30 years (24.0 ± 3.6) participated in the study after signing informed consent forms. The protocol was approved by the University Ethics Committee (N_11-068-CERSS-D).
2.2. Task description
The task consisted in lifting (CON) or lowering (ECC) a loaded box of 6, 12 or 18 kg from one shelf to another (3 conditions). Women performed the tests with only the 6 and 12 kg boxes. The shelves were placed at three different heights, corresponding to the hip, shoulder and eye levels of each participant. The dimensions of the boxes were 0.345 × 0.395 × 0.08 m (length, width and height). Subjects were standing in front of the shelves at their preferred horizontal distance, with their feet parallel and naturally spaced. Subjects performed three trials for each condition of height, weight and direction (lifting or lowering).
2.3. Data recording and analysis
Surface EMGs were recorded at a sampling frequency of 2 kHz from 10 shoulder muscles (right side). Wireless surface electrodes (Trigno™ IM, Delsys Inc., Boston, MA) were placed over the anterior (DeltA), middle (DeltM) and posterior (DeltP) parts of the deltoid, the long heads of the biceps brachii (BB) and triceps brachii (TB), the superior (TraS) and lower (TraL) parts of the trapezius, the serratus anterior (SerrA), the pectoralis major (sternal portion – Pect) and the latissimusdorsi (LD). In a subset of 10 subjects, additional EMGs (Trigno™ Spring; Delsys Inc., Boston, MA) were recorded from the supraspinatus (SupS), infraspinatus (InfS), and lower subscapularis (SubS) using fine-wire intramuscular electrodes (30 mm, 27 gauge; CareFusion) inserted into the muscles.
EMG signals were band-pass filtered (4th-order Butterworth) between 20 and 400 Hz. When needed, electrical noise was removed using a notch filter at 60 ± 0.3 Hz. Integrals of the rectified EMG signals were computed (35-ms window) to obtain the EMG profiles (iEMG). iEMGs were normalized by the maximum iEMG extracted from maximal voluntary contractions (MVC).
The forces applied to the right handle were simultaneously recorded (custom-made 6-dof force sensor by Sensix, France) at a sampling frequency of 1 kHz. EMGs were analysed only when external forces were applied to the box.
Muscle synergies were extracted using non-negative matrix factorization (NNMF – Lee and Seung Citation2001). The accuracy of the model reconstruction was measured using the variance accounted for (VAF), computed as: VAF = 1-SSE/SST. SSE is the sum of squared residuals and SST the total sum of the squared values. The number of synergies was defined as the minimal number for which the lower bound of 95%-confidence interval of the total VAF was greater than 90%. As we compared only CON and ECC, analysis was performed on concaneted data, including the three loads and the three heights conditions.
2.4. Statistics
Data normality was verified using Shapiro-Wilk tests. Pearson’s r was used to compare the synergy vectors. Muscle activation and synergy activation levels were compared between CON and ECC using paired t-tests. ECC synergy patterns were reversed in time in order to compare the activations relative to the position on the trajectory (up or down). For multiple comparisons α-value (0.05) was adapted using the Holm–Bonferroni method.
3. Results and discussion
Muscle activity was less than 20% MVC on average and the most activated muscles were DeltA, DeltM, TraS, SerrA and SupE (∼10–20% MVC). Muscle activity was greater in some muscles during ECC () but with differences less than 1.46 ± 1.66% MVC (DeltA).
Figure 1. Difference in mean muscle activation levels between ECC and CON. Positive values indicate greater muscle activity during ECC. *: p < 0.05. values are presented as mean ± SD.
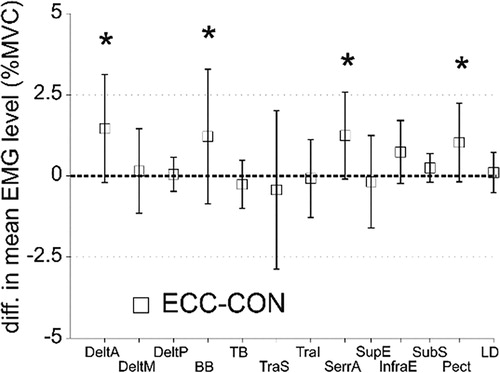
The number of synergies varied between 1 and 3 across subjects with a modal value of s = 2 observed in ∼75% of the subjects. Adding intramuscular recordings (rotator cuff muscles) did not change this distribution, showing that they do not belong to specific synergies. The number of synergies did not vary between CON and ECC (p = 1). The first synergy mainly activated prime mover muscles, i.e., the anterior and medial deltoids, the trapezius superior and the serratus anterior, suggesting a role in driving the movement. The second and third synergies co-activated most of the recorded muscles, suggesting a role in stabilizing the glenohumeral joint. For reasons of statistical power, we focused the rest of the analysis on the 75% of subjects with 2 synergies. Synergy vectors were similar between CON and ECC, i.e. r = 0.96 ± 0.04 and 0.84 ± 0.14 for synergy #1 and #2, respectively. Activation of synergy #1 was lower in ECC at the lowest position on the trajectory (arms down; ). Activation of synergy #2 was greater at times corresponding to mid-range of motion (∼20–55%) and end-range of motion (∼90–100%). Mid-range and end-range of motion correspond to when the tension provided by the glenohumeral ligaments is negligible and to positions at which glenohumeral dislocations usually occur, respectively. These observations strongly support the hypothesis that synergy #2 participates in glenohumeral stabilization.
4. Conclusions
Lifting (CON) and lowering (ECC) a load requires two synergies in most subjects (∼75%). The number and structure of the synergies were similar in CON and ECC suggesting that contraction mode has negligible effects on the synergies. However, muscle activation levels differ between CON and ECC. Synergy #1–that we suggested could drive the movement–was slightly less activated during ECC. Conversely, synergy #2–that we suggested could stabilize the glenohumeral joint–was more activated in critical phases of the lowering movement at which the joint is known to be the most unstable.
The synergy analysis might be able to disentangle and quantify the activity of the shoulder muscles associated with either the stabilization of the shoulder or with the movement itself.
Additional information
Funding
References
- Borstad JD, Ludewig PM. 2002. Comparison of scapular kinematics between elevation and lowering of the arm in the scapular plane. Clin Biomech (Bristol, Avon). 17(9–10):650–659.
- Duchateau JRM, Enoka RM. 2008. Neural control of shortening and lengthening contractions: influence of task constraints. J Physiol. 586(24):5853–5864.
- Lee DD, Seung HS. 2001. Algorithms for non-negative matrix factorization. In: Advances in neural information processing systems. Cambridge, MA: MIT Press; p. 556–562.