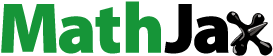
1. Introduction
Tibial plateau fractures correspond to articular injuries. It represents 1.03% of all bone fractures in general population and 8% in the elderly population. The most frequent tibial plateau fractures are known as type II in the Schatzker classification (Kfuri and Schatzker Citation2018). This type of fracture corresponds to the splitting and depression of the lateral tibial plateau.
In 2013, Vendeuvre et al. published a new minimally invasive technic for Schatzker type II fractures, using an expanding balloon for the fracture reduction, facilitating possible PMMA (PolyMethylMeth-Acrylate) cement injection and bi-cortical screws for stabilization (Vendeuvre et al. Citation2013). Full-weight-bearing is recomanded 84 days after the surgery.
The aim of this study is to assess the effect of PMMA cement injection and the influence of the length of the screw used for stabilization of tibial plateau fracture type Schatzker II (Kfuri and Schatzker Citation2018), on the risk of implant failure (PMMA and screw) and the risk of fracture instability during full weight bearing at different time after the surgery, including earlier full weight bearing, recommended full weight bearing and year(s) after the surgery walk.
2. Methods
The studied tibial plateau fracture, type Schatzker II, came from a male patient of 40-year-old who weighted 80 kg and was 1.82 m tall. The fracture was treated following Vendeuvre et al. (Citation2013) method. The stabilization consisted of PMMA injection and implantation of one bi-cortical screw. The 3D image was acquired some minutes after the surgery with a medical 3D X-Ray CT-Scan (O-ARM® IMAGING SYSTEM, Medtronic). Image resolution: ( Reconstructed matrix size: (
We extracted the bone geometries and the PMMA cement form the 3D image with 3D Slicer Software (V4.10.1). This segmentation step was realized by a semi-automatic method based on region growing algorithm. Two segmentations were produced. For the first one, we segmented 5 five geometries (unbroken tibia; fibula; PMMA cement, separated fragment, and depressed fragment; ). It models the discontinuities observed between bone fragments until 12 weeks after the surgery. The second segmentation considered a single geometry for the tibia which represents fragment merging a year after the surgery. This second segmentation was composed of three geometries [tibia; fibula and PMMA cement]. The screw’s axis was identified to place the imported screw into the segmented geometries along the axis. We choose to import the real screw geometry to ensure better fidelity of the screw thread and to control its length as a parameter of the study.
Figure 1. Schematic representation of the bone fragments and surgical materials in the situation with PMMA cement and one bi-cortical screw (L = 74 mm).
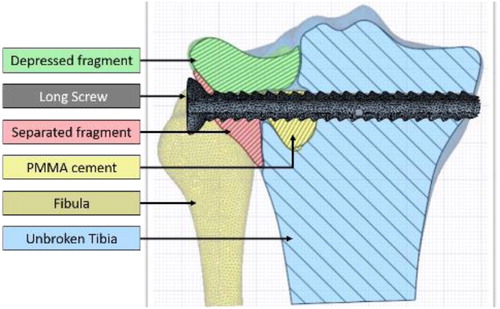
All the geometries were imported in Simpleware™ software (Version 2019.09; Synopsys, Inc., Mountain View, USA) to perform contact matching, meshing and material allocation: Boolean operations were applied to ensure good connectivity between the geometries. The selected mesh leaded to a structured tetrahedral elements mesh. The bone material properties were assigned to mesh elements depending on the Hounsfield units of the image. The relationship between local Hounsfield units and the Bone Mineral Density (BMD) () were calibrated with an Electron Density Phantom [CIRS Model 062M]. The relationship between local BMD and local Young Modulus was defined by two functions (Carter and Hayes Citation1977; Rho et al. Citation1995).
- Trabecular bone:
- Cortical bone as
The BMD limit between trabecular and cortical bone was set as to correspond to the interception between the two functions. We used standard values of Young modulus and the Poisson ratio of the PMMA and titanium screw. The boundary conditions were defined to correspond to the maximum load during gate, which correspond to 2.59 times the body weight distributed between the lateral and the medial plateau [37–63%] and were applied on surfaces tibial plateau surfaces [
]. Distal surfaces of the tibia and the fibula were considered with zero displacements. To avoid local BMD error due local non-correspondence between the bone geometries and the Hounsfield values in the 3D image, we defined a threshold for which the higher value is defined as homogeneous trabecular bone BMD.
In men, BMD decreases of about 1% per year soon after a peak of bone mass, which is estimated at age 40 years (Walsh and Eastell Citation2013). In order to take into account, the effect of BMD evolution during aging, we apply a coefficient the calibrated Bone Mineral Density. The effect of the time after the surgery was represented by the contact behaviour between the geometries and the decrease of BMD ().
Table 1. Contacts and BMD according to the time after the surgery.
In this study, we varied three parameters in the model. The first and the second were linked to modifications of the type of stabilization: the length of the screw [ or
] and the cavity filling or not by PMMA cement. The third was the time after the surgery as presented in the . The mechanical stability of the bony structures was evaluated with comparison of the stress fields and regarding to the local yield strength of the bone (Cook and Zioupos Citation2009; Havaldar et al. Citation2014). This expressed as the volume bone with a stress value above the local yield strength ().
3. Results and discussion
In all scenarii, we found that the titanium screw and the PMMA cement can handle the mechanical stress regarding to their yield strength. The stress in bone was maximized in earlier full-weight-bearing, particularly in the surgical procedure including uni-cortical screw and no PMMA cement injection (). We found that the length of the screw had no significative influence in the situations from 84 days to 10 years. With PMMA filling and a bi-cortical screw, we found that a 21-day full weight bearing is equivalent to one at 84 days (). For surgeons, it is desirable that elderly people be able to support their weight sooner because prolonged bed rest often leads to complications.
On the other hand, for young people, surgeons wish to be as minimally invasive as possible. In this clinical application, the nonabsorbable nor removable PMMA is a question. Our model shows that after 84-days the full weights bearing with and without PMMA stabilization are equivalent.
4. Conclusions
This personalized specific model was based on the geometry, the bone density, the fracture and the weight of the patient. We simulated the full weight bearing of the patient at five different times after the surgery. We evaluated the influence of the PMMA filling and the screw length on stress in the bone, PMMA and screw. We found that the risk of implant failure is null in all situations and that the PMMA injection allows earlier full weight bearing but is not essential in current clinical protocol. These results, albeit model-dependent, could be valuable for clinical practitioners to understand the consequences of the type of stabilization in the patient’s continuation of life.
Disclsoure statement
The authors would like to thank ANSYS France.
References
- Carter DR, Hayes WC. 1977. The compressive behavior of bone as a two-phase porous structure. J Bone Joint Surg Am. 59(7):954–962.
- Cook RB, Zioupos P. 2009. The fracture toughness of cancellous bone. J Biomech. 42:2045–2060.
- Havaldar R, Pilli SC, Putti BB. 2014. Insights into the effects of tensile and compressive loadings on human femur bone. Adv Biomed Res. 3(1):101–997.
- Kfuri M, Schatzker J. 2018. Revisiting the Schatzker classification of tibial plateau fractures. Injury. 49(12):2252–2263.
- Rho JY, Hobatho MC, Ashman RB. 1995. Relations of mechanical properties to density and CT numbers in human bone. Med Eng Phys. 17(5):347–355.
- Vendeuvre T, Babusiaux D, Brèque C, Khiami F, Steiger V, Merienne J-F, Scepi M, Gayet LE. 2013. Tuberoplasty: minimally invasive osteosynthesis technique for tibial plateau fractures. Revue de Chirurgie Orthopédique et Traumatologique. 99(4):S47–S272.
- Walsh JS, Eastell R. 2013. Osteoporosis in men. Nat Rev Endocrinol. 9(11):637–645.