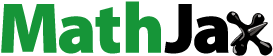
1. Introduction
Running involves repeated impacts between the foot and the surface. Such impacts are characterized by a transient peak in the ground reaction force (impact force), rapid deceleration of the lower extremity (impact shock), and the initiation of a wave of acceleration and deceleration (impact shock wave) that is propagated through the body. The loads produced by repeated impacts have been linked to degenerative joint diseases and athletic overuse injuries including for example stress fractures, shin splints, osteoarthritis and lower back pain. Although the exact mechanisms of impact related injury are relatively unknown and controversial evidence linking impact and injuries is well documented.
The purpose of this study was to determine the effects of increasing impact shock levels on the spectral characteristics in the body during treadmill running. The main aim is to analyse the ability of the human musculoskeletal system to attenuate the mechanical stresses resulting from the fatigue effect by Shock Responses Spectrum (SRS) of the foot strike–generated shock waves during running. SRS is a frequency-based function that is used to indicate the magnitude of vibration due to a shock or transient event. It’s an analysis method of transitional systems (shocks) developed by the military industry to determine how equipment reacts to repetitive stress. From there on, transposing this method to running gait analysis there is only one step.
2. Methods
2.1. Procedures
Twelve male runners who were free from musculoskeletal injury volunteered to take part in this study. The runners had a mean age of 30.3 (±4.9) years, stature 178.3 (±5.7) cm, and body mass 77.7 (±8.5) kg respectively.
One inertial sensor (HIKOB FOX®) was used and placed on the distal anteromedial portion of the tibia to collect data on the 3-axis acceleration measure. The MEMS accelerometer was placed on the skin using adhesive strapping band, such an attachment, as proven in earlier works can accurately measure shock wave amplitude according to Winslow and Shorten (Citation1989). The accelerometer was aligned along the longitudinal axis of the tibia to provide axial components of tibial acceleration. Participants were given a 5 minutes’ adaptation period, in which participants ran at the determined velocity of 3.3 m/s. The treadmill was then stopped for 30 s and participants dismounted the treadmill before mounting the treadmill for data recording. When participants indicated that they were ready to begin, the treadmill was restarted and the velocity of the belt was gradually increased until the speed reached 2.2 m/s for a first recording of 30 s then the speed was again increased to reach 3.3 m/s for a second recording of 30 s. To minimize possible gait modifications, the subjects were not aware of when exactly the data were acquired.
2.2. Spectral analysis of the impact shock wave
Spectral analysis is commonly used to study the structure of composite wave forms such as the impact shock waves. The primary tool of spectral analysis is the Fast Fourier Transformation (FFT) that enables us to determine the runner’s natural frequency () which corresponds to the peak of the Power Spectral Density.
Power Spectral Density (PSD) provides a convenient method of separating different frequency components in the impact shock wave such as acceleration moments due to impact shock.
The runner’s natural frequency then enables us to determine his time step and his running pattern. We then carry out the cross-correlation CC (τ) between the runner’s pattern and the recording’s duration a(t).
We observe that at each step, the convolution is maximum. For each maximum value of CC(τ) we calculate the SRS of each step and of the entire signal using Lalanne (Citation2009) algorithm for shock analysis. SRS enables us to determine the maximum acceleration a system will undergo when one knows the natural frequency f0 and the quality factor Q for each possible natural frequency. SRS can also be calculated for the entire duration of a recording (). We then observe the peaks at the fundamental and harmonic frequencies of the recorded signal. In this context SRS combines both the notion of transfer function and response to transient regimes.
Intra comparison of the SRS offers a lot more finesse to the analysis since the frequency is also taken into account. The aggressiveness of a running step is not only due to the value of the maximum acceleration, but also to the general shape of the movement, only the SRS allows this to be taken into account in the analysis.
3. Results and discussion
A goal of the present study was to analyze the effect of fatigue through SRS on the ability of the human musculoskeletal system to attenuate foot strike–generated shock waves. Fatigue hampers the ability of the human musculoskeletal system to protect itself from overloading due to foot strike–generated shock waves. Loss of protection may be manifested in an increased shock wave amplitude as measured on the tibial tuberosity. The results were obtained through this protocol while subjects ran on a motor-driven treadmill. While such a setup simplified data acquisition, the locomotion patterns obtained may differ from patterns for overground running. The runners in the present study were constrained to run at a constant speed, regardless of whether they were fatigued or not. Since one of the main purposes of studying SRS was to introduce the correlation between running fatigue and lower limb injuries it is important to note that this may slightly differ in overground running: When fatigue begins, runners may slow down as a protective means. The result could be moving away from the state of fatigue, in which case the acceleration data might not increase. According to the results in this study, for the acceleration data to increase, fatigue should be present. Thus, the results of this study can be extrapolated to overground running if fatigue indeed prevails. Also, most running injuries in overground running are lower extremity injuries, with a predominance of knee injuries and our results indicating an increase in acceleration data in the tibial tuberosity therefore tend to support this extrapolation. Previous studies have shown that the loading rate of the lower limb is directly and highly correlated with running speed, and the vertical impact force increased with increasing running velocity.
Muscles activation lowers the bending stress on bone and attenuate the peak dynamic loads that can damage musculoskeletal tissues. Previous studies have suggested that the fatigued muscles cannot support “optimal” running and they also suggested that fatigue of the runner may lead to modification of landing phase mechanics. It was also found that the transfer of mechanical energy between the eccentric and concentric phases is drastically reduced during muscle fatigue. Such changes may be involved in the development of injuries.
4. Conclusions
We may conclude that the musculoskeletal system becomes less capable of handling foot strike–induced shock waves when the muscles are significantly fatigued. Understanding the influence of SRS on fatigue and on the magnitude of dynamic loading on the human musculoskeletal system will allow the development of proper training procedures and exercises, and will reduce damage to the musculoskeletal tissues.
References
- Lalanne C. 2009. Mechanical vibration and shock analysis. Vol. 2, Mechanical shock. 2nd ed. Wiley, USA.
- Winslow D, Shorten M. 1989. Spectral analysis of impact shock during running. J Biomech. 22(10):1099.