1. Introduction
In the human body, assessing muscle forces can be done using either experimental or mathematical approaches. The former often relies on the use of surface electromyography (EMG) measurements, although they are indirect measurements of the force and their signal needs to be treated. Moreover, experimental approaches have a limited scope as some muscles are not accessible and the number of subjects is often low. On the opposite, mathematical approaches are used to develop generic musculoskeletal models embracing a larger population. These models are often based on - or derived from - the Hill model. However, their constitutive parameters are numerous and delicate to estimate. Furthermore, due to muscle redundancy, there is an infinity of solutions and the found solution may not be physiological.
So, hybrid approaches are of particular interest (Hinnekens et al. Citation2019). The one used in the frame of this research lies on making the most of EMG signals to enrich the model inputs and to narrow the optimization parameters space when dealing with muscle redundancy.
The hybrid approach is used to quantify trunk muscle forces involved in the Sorensen test (). This test was chosen for its simplicity, the perfect knowledge of the external force (gravity) and the great muscle forces involved. The present paper focuses on the calibration step, i.e., identifying muscle forces involved in the static configuration of the Sorensen test and correlating them to their corresponding EMG signals.
2. Methods
Seventeen healthy female (age: 21.9 ± 2.6 years; mass: 63.6 ± 5.8 kg; height: 1.68 ± 0.05 m) and 19 healthy male (age: 22.7 ± 2.1 years; mass: 73.9 ± 8.7 kg; height: 1.81 ± 0.05 m) subjects were recruited for this experiment. After an appropriate warm-up, each subject completed the whole experiment which consisted in three maximum isometric voluntary contractions (MVC) followed by five static loaded contractions (from 0 to 8 kg in 2 kg steps).
MVC tests () required a particular attention to be as standardized as possible through all the subjects. So, three MVC tests were performed for each subject to have two close and usable MVC tests to compute their mean. To ensure the static position of the posture, subjects were linked to the ground via a slack. A force sensor, located in series with the slack, recorded the force developed during each MVC test. The force was plotted in real time on a screen placed on the ground in order to give the subject a visual feedback. Finally, a standardized auditive feedback was also given: the experimenter counted from 0 to 6 seconds during the contraction with a neutral but loud voice.
During the whole experiment, muscle activity was recorded by EMG measurements (FreeEMG, BTS, 1000 Hz), bilaterally placed on the rectus abdominis, the external oblique muscle, the lumbar and dorsal paravertebral muscles, the latissimus dorsi and the quadratus lumborum. EMG signals were rectified, filtered, smoothed and normalized. Their activation level was obtained by computing the root-mean-squared (RMS) value over the activation time. From EMG signals assessed during the five static loaded contractions, a numerical regression was performed between loaded masses and activation levels to study the influence of adding external masses.
3. Results and discussion
The force measured by the sensor during MVC tests gives a first indication of the force that each subject can develop with his trunk muscles, which are counterbalancing this force and the trunk weight. It confirmed that males were stronger than females () as previously showed (Larivière et al. Citation2006).
Table 1. Forces recorded from the sensor (mean ± SD).
For static tests, results in all subjects showed an increase of the EMG amplitude, especially in lumbar paravertebral muscles (). The most the muscle is loaded with mass, the highest the magnitude of the EMG signal is, which corresponds to an increase in the number of active units in this muscle (Hof Citation1997). This linear increase has already been showed in a previous study (Hinnekens et al. Citation2019) but it is confirmed here on a larger population. Beyond this expected behavior, it is interesting to notice how well the linear regression fitted experimental data ().
Figure 2. Linear regression between loaded masses and activation levels for the right lumbar paravertebral (LPR) muscles for (left) men and (right) women. Mean linear regression for each gender is showed in red.
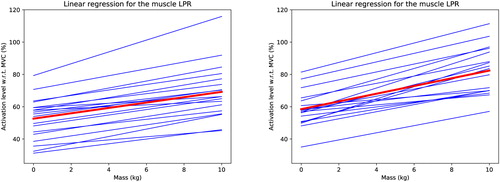
Figure 3. Linear regression between loaded masses and activation levels for both right and left lumbar paravertebral muscles for two male subjects (one in each color).
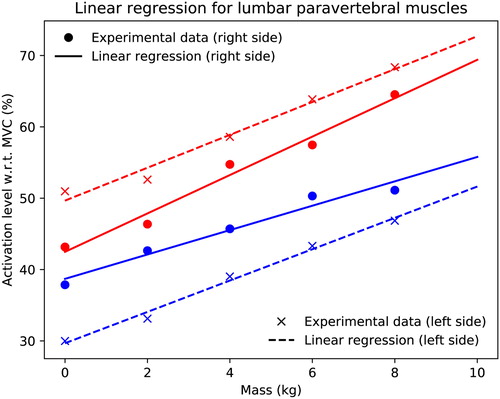
Results from also highlighted that EMG signals depended on multiple factors such as the EMG localization on the skin and skin surface properties, which resulted in different skin impedances. Consequently, the calibration step cannot be dissociated from the subject and overall from the full experimental process. That being agreed, EMG signals (and their linear relationship with the load) become a valuable input for musculoskeletal models and can, in particular, narrow the optimization parameters space to quantify muscle forces through an inverse dynamics approach (Raison Citation2009).
4. Conclusions and prospects
After completing this experimental calibration step, future work will focus on the development of the musculoskeletal multibody model, for the computation of muscle forces. Doing so, we will finalize the whole calibration process, the latter being required for the subsequent multibody dynamic optimization that will consider the body motion. This will finally lead to the development and the quantification of dynamic movements, e.g., arch-up tests.
Acknowledgements
The main author gratefully thanks Julie Bara and Pauline Scheen, master students, for their contribution to the present work as part of their master thesis.
Disclosure statement
No potential conflict of interest was reported by the authors.
References
- Hinnekens S, Mahaudens P, Detrembleur C, Fisette P. 2019. EMG Measurements as inputs for a musculoskeletal model: quantification of abdominal and back muscle forces in static postures. Comput Methods Biomech Biomed Eng. 22(S1):147–149.
- Hof AL. 1997. The relationship between electromyogram and muscle force. Sportverletz Sportschaden. 11(03):79–86.
- Larivière C, Gravel D, Gagnon D, Gardiner P, Bertrand Arsenault A, Gaudreault N. 2006. Gender influence of fatigability of back muscles during intermittent isometric contractions: a study of neuromuscular activation patterns. Clin Biomech. 21(9):893–904.
- Raison M. 2009. On the quantification of joint and muscle efforts in the human body during motion [dissertation]. Ottignies-Louvain-la-Neuve: Université catholique de Louvain.