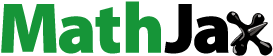
Keywords:
1. Introduction
Vibration could affect the health and the comfort of manual wheelchairs (MWC) users (Garcia-Mendez et al. Citation2013). Literature already showed that during the daily use of a MWC, users are exposed to higher vibration level (Aseat = 0.83 m/s2) than recommended by the international standard ISO-2631 on human vibration exposure (Garcia-Mendez et al. Citation2013). This standard specifies an acceptable vibration dose of 0.5 m/s2 for an 8-h exposition. Until now, most studies focused on vibration exposure of a seated occupant and factors (e.g. cushioning, wheels, speed) that could affect this vibration exposure (Requejo Citation2008). Such empirical analyses cannot be performed systematically to assess the effect of each MWC element on the exposure to vibration. This is, however, a critical step for a better understanding of vibration transmission and, therefore, encountered settings allowing performance and comfort improvements of MWC. For this purpose, a better understanding of the MWC dynamical behaviour is required.
Under this framework, the present study aimed to identify the vibrational content that could be transmitted from the MWC structure to the users’ body through an experimental modal analysis.
2. Methods
2.1. Mesh
The experimental modal analysis was done on a standard MWC. This method allows identification of vibration parameters (eigenfrequencies, damping ratios and modes shapes). Fifty-five points distributed over the MWC structure were highlighted with colour stickers. A 3D scan was performed (iSense iPad 4G, 3D Systems) and 3D coordinates of the 55 points were extracted from the 3D mesh using a picking and colour identification procedure.
2.2. Measurements
Three mono-axial accelerometers (PCB Piezotronics; ±500 g pk; [1 − 8000] Hz; sampling rate 20 kHz) were fixed to the right seat support and aligned as defined by the ISO-2631. The 55 points of the mesh were successively excited with an impact hammer (PCB Piezotronics; ± 444 N pk; resonant frequency ≥ 15 kHz) to obtain a force signal with an acceptable bandwidth, a low noise level and no secondary impact; the MWC resting with the four wheels on the floor.
2.3. Data processing
To remove the noise, a uniform decay window was applied on force data. Also, an exponential window was applied on acceleration data to reduce leakage. To obtain eigenfrequencies, damping ratios and mode shapes, frequency response functions (FRF) Hij were calculated as
(1)
(1)
where f is the frequency vector, Aij the acceleration of the point i measured by the accelerometer j, and Fi the impact force at point i. FRFs were analysed up to 80 Hz, accordingly to the seated human body sensitivity response to vibrations (Griffin Citation1990). Modal analysis parameters were identified using the Structural Dynamic Toolbox (Balmes Citation1997) running on MATLAB R2019b. Besides, although the modal analysis was carried out on the whole MWC, results focused on parts directly in contact with the users: the seat and the backrest.
3. Results and discussion
A typical magnitude curve with modes shapes obtained by the modal analysis is presented in . Eigenfrequencies and damping factors are provided in .
Figure 1. Mean of FRFs of the MWC under simply supported boundary conditions. Twisting (T) and fore-aft bending (B) modes of the couple backrest/seat are presented. Modes shapes are illustrated for each eigenfrequency, the black dashed wheelchair referred to the rest position and the blue lines indicated its deformation.
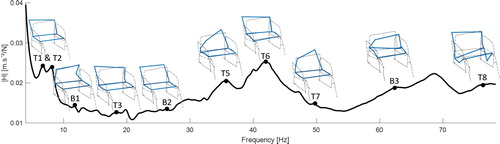
Table 1. Modal analysis result of the wheelchair.
3.1. Frequency and damping ratio
The first two eigenfrequencies were in the range of the human seated fundamental modes frequencies (0–10 Hz) (Griffin Citation1990). This means that vibration might be amplified by the MWC and the users body. Frequencies of backrest mode shapes T1 and T2 are near from transmissibility peaks frequencies reported in the literature (5–7 Hz, 8 Hz and 13–15 Hz) in a study about the transmissibility of MWC/users couple (Maeda et al. Citation2003).
3.2. Mode shape
At eigenfrequencies, deformations of the seat and the backrest occurred, which could affect the comfort. Two types of mode shapes were identified: fore-aft bending and twisting modes. Lo et al. (Citation2013) did not identify mode below 20 Hz or above 65 Hz but they also observed fore-aft bending mode around 33.3–48 Hz and twisting mode around 42.4–62.4 Hz for car seat structure. Unlike MWC, car seat have a lateral bending mode. Differences observed between MWC and car seat modal analysis could be explained by the structural differences. For a human/seat structure, Lo et al. observed similar mode shapes but with lower eigenfrequency.
4. Conclusions
This study aimed at carrying out a modal analysis of a standard MWC. MWC seat structure exhibited many eigenfrequencies in the range of human frequency sensitivity that could damage user’s health and comfort. This preliminary study outlined the interest of modal analysis to understand, and then, enhance the design of future MWC with respect to vibration exposure. As the user’s body most probably modifies the dynamical response of the structure, future work will include the user’s effect through an operational modal analysis.
References
- Balmes E. 1997. GARTEUR group on ground vibration testing. Results from the test of a single structure by 12 laboratories in Europe. Proceedings of 15th IMAC. USA. pp. I346–1352.
- Garcia-Mendez Y, Pearlman JL, Boninger ML, Cooper RA. 2013. Health risks of vibration exposure to wheelchair users in the community. J Spinal Cord Med. 36(4):365–375.
- Griffin MJ. 1990. Handbook of human vibration. London: Academic Press.
- Lo L, Fard M, Subic A, Jazar R. 2013. Structural dynamic characterization of a vehicle seat coupled with human occupant. J Sound Vib. 332(4):1141–1152.
- Maeda S, Futatsuka M, Yonesaki J, Ikeda M. 2003. Relationship between questionnaire survey results of vibration complaints of wheelchair users and vibration transmissibility of manual wheelchair. Environ Health Prev Med. 8(3):82–89.
- Requejo PS. 2008. Effect of rear suspension and speed on seat forces and head accelerations experienced by manual wheelchair riders with spinal cord injury. J Rehabil Res Dev. 45(7):985–996.