1. Introduction
Numerous diseases are associated with (or due to) a pathologic calcification of the skeleton. It may involve a defect in calcification (as in bone in osteoporotic patients) or on the contrary, an excess of calcification, or even the appearance of calcification in a normally non-calcified tissue. Understanding the effect of calcification on skeletal tissue properties is therefore a milestone to propose preventive or therapeutic strategies. In vitro studies involving murine models with normal or pathologic calcification have been used (Leroux-Berger et al. Citation2011) but results are generally limited to histological observations. In addition to such observations, mechanical testing may give an access to the macroscopic mechanical response of small skeletal samples and permit comparative studies, but does not provide with a characterization of local tissue properties. Alternatively, various studies (Laurent et al. Citation2016) have emphasized that Computed Tomography (CT) may be used to predict overall bone stiffness using subject-specific Finite Element (FE) models. FE models may then be used to identify bone properties using an inverse method, based on experimental biomechanical data, in order to identify bone properties. However, in the case of non-calcified tissue, the geometries issued from CT may be insufficient, and completed by Magnetic Resonance Imaging (MRI) (Singh et al. Citation2018) in order to characterize immature bone or fibrocartilage. In this study, combined CT-MRI imaging are used to characterize normal and pathologic calcification, and then enables to build subject-specific FE models of murine femurs to identify corresponding skeletal tissue properties.
2. Methods
Normal and pathological calcifications were studied by comparing a mouse model deficient in matrix Gla protein (Mgp) (a calcification inhibitor) with a non-mutated control. Such a mutation has indeed been proved to lead to a massive calcification of arteries (Leroux-Berger et al. Citation2011). Femurs were harvested at 2 (n = 4), 3 (n = 4) and 4 (n = 4) weeks for both pathologic (Mgp -/-) and normal (Mgp +/+) models. Right femurs were used for three-points bending mechanical testing (Zwicki Z0.50 TN with a 100 N load cell) as commonly reported in bone studies (Hambli and Thurner Citation2013), while left femurs were used for histological analysis and 3 D imaging using both CT (EasyTom Nano, RX Solutions) and MRI (Bruker Avance III 600WB spectrometer) modalities.
Bone geometries were reconstructed using previously reported methods (Laurent et al. Citation2016) to build a subject-specific FE model of each femur, with density-elasticity relationships that constituted the unknown to be identified together with the yield stress, considered as a function of the elastic modulus of each element. The elastic properties of skeletal tissue were assessed from inverse method based on the experimental bending stiffness and the information available in calibrated CT, considering that the FE models provide with reliable bending response prediction as reported in (Laurent et al. Citation2016). The effect of calcification was taken into account in the computations by introducing an isotropic damage variable (Mengoni and Ponthot Citation2010), taking the fully calcified bone as the undamaged reference.
3. Results and discussion
Murine femurs were reconstructed from both MRI and CT images. Differences were observed between the two imaging modalities for both normal and pathologic mice at the level of articular cartilage and growth plates, while the overall reconstructed geometries were similar for both imaging modalities ().
Figure 1. Method for tissue properties characterization at various stages of calcification. Mutated and normal mice were subject to MRI/CT combined imaging and three-points bending testing, in order to identify skeletal tissue properties from an inverse approach based on FE results.
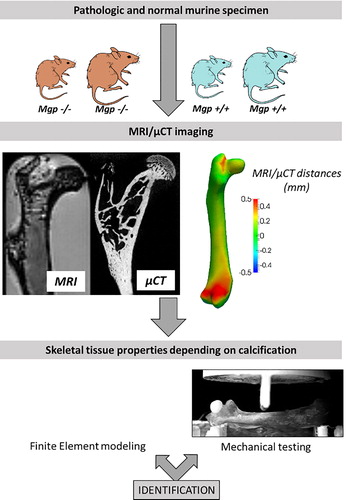
The skeletal properties were affected by the pathologic calcifications since Mgp -/- mice showed an increased stiffness and brittleness. Histological observations emphasized the altered calcification in Mgp -/- model. Subject-specific FE models were satisfyingly reconstructed () and the inverse approach using FE model enabled to identify a suited density-elasticity relationship for murine mature skeleton. As an illustration, the correspondence between best-fitted FE results and experimental results for non-pathological femurs is represented in with the corresponding density-elasticity relationship. For this sample, yield stress was set at 3.6% of Young for each element, and the identified elastic modulus was 678 ± 265 MPa.
4. Conclusions
This work constitutes a first step towards a simple and efficient identification method of the skeletal tissue mechanical properties in response to a simulated pathology, based on validated computational methods. The first results concerning the evolution of skeletal properties during calcification may help to understand the mechanisms underlying mechanical modifications of pathological tissues. This constitutes a solid database in order to build constitutive laws in the framework of continuum damage mechanics to take into account skeletal calcification. From an ethical point of view, the presented approach also enables to take full advantage of the necessary use of animal models by extending the range of standard methods to non-invasive computational modelling.
Additional information
Funding
References
- Hambli R, Thurner PJ. 2013. Finite element prediction with experimental validation of damage distribution in single trabeculae during three-point bending tests. J Mech Behav Biomed Mater. 27:94–106.
- Laurent CP, Böhme B, Mengoni M, d’Otreppe V, Balligand M, Ponthot J-P. 2016. Prediction of the mechanical response of canine humerus to three-point bending using subject-specific finite element modelling. Proc Inst Mech Eng H. 230(7):639–649. ].
- Leroux-Berger M, Queguiner I, Maciel TT, Ho A, Relaix F, Kempf H. 2011. Pathologic calcification of adult vascular smooth muscle cells differs on their crest or mesodermal embryonic origin. J Bone Miner Res. 26(7):1543–1553.
- Mengoni M, Ponthot JP. 2010. Isotropic continuum damage/repair model for alveolar bone remodeling. J Comput Appl Math. 234(7):2036–2045.
- Singh S, Bray TJP, Hall-Craggs MA. 2018. Quantifying bone structure, micro-architecture, and pathophysiology with MRI. Clin Radiol. 73(3):221–230.