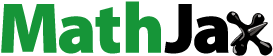
1. Introduction
The temporomandibular joint (TMJ) disc is a fibrocartilaginous intra-articular tissue that connects the skull and mandible, and ensures the correct motion of the jaw (Tappert et al. Citation2017). Unphysiological mechanical stress and trauma, such as in car crash, sport accidents or whiplash, are well-known causes of permanent TMJ disorders (Pérez del Palomar and Doblaré Citation2008). To date, the TMJ disc mechanical behavior has been mainly documented under reduced strain (e.g., 10%) and at low strain rates (<1 s−1), far to be representative of trauma and unphysiological conditions (Fazaeli et al. Citation2019). The exact aetiology of such trauma-induced disorders is complex and remains largely unresolved as a paucity of investigations exists into TMJ disc high rate behavior. In this study, we investigate the compressive mechanical properties of porcine TMJ discs at strain rates of 1118 ± 56 and 2553 ± 281 s−1. We used a modified split-Hopkinson pressure bar (SHPB) embedded with quartz crystal transducers to measure the loading forces. The displacements were obtained using digital image correlation (DIC). The experimental data were, then, fitted with established hyperelastic models, and parameters were extracted for 3-dimensional (3-D) finite element (FE) modelling (Pérez del Palomar and Doblaré Citation2008).
2. Methods
2.1. TMJ disc sample preparation
A total of 6 cylindrical TMJ discs were carved out from three porcine heads by punching the intermediate zone. The nominal punch diameter was either 5.08 (small) or 7.62 mm (large). The disc samples were directly stored in a −20 °C freezer for 7 days at most. On the day of testing, the samples were allowed to thaw in a pre-warmed 39 °C water bath, and their actual diameter and thickness were carefully determined on photographs by image analysis (e.g., 5.53 ± 0.25 and 8.53 ± 0.18 mm for the small and large diameter, respectively). Just prior to the compressive experiments, any pre-strain due to the setup was determined using a calliper.
2.2. High strain rate compressive loading
The high strain rate compressive experiments were performed on a modified ½ inch SHPB system instrumented with inertia-compensated quartz crystal transducers and a charge amplifier (Sory Citation2017). The input bar (IB) and output bar (OB) were made of 7068 Al alloy and were 1500 mm and 1000 mm long, respectively. The compressive loadings were generated by impacting the IB at velocities up to 6.40 ± 0.10 m.s−1 with a 560 mm long projectile. The force at the IB- and OB-sample interfaces, FIB and FOB respectively, were obtained from the quartz transducers using pre-established ‘voltage-force’ calibration relationships, and then processed into average engineering stress-history as:
where As is the sample cross-sectional area. The displacements at the IB- and OB-sample interfaces, uin and uout respectively, were recorded on a Phantom camera at 93137 frames per second, and then computed from digital image correlation (Ncorr, MatLab). The sample average engineering strain-history was processed as:
where Ls is the sample length. Even though the sample undergoes compression, the curves are presented with positive values for better reading.
2.3. TMJ disc hyperelasticity modelling
The experimental stress-strain data were fitted with the incompressible uniaxial hyperelastic Mooney-Rivlin model with the engineering stress defined as:
where
is the stretch while
and
are parameters related to the shear modulus such as:
The parameter least square optimization has been done with a custom-made Python script through Scipy library on complete loading curves.
3. Results and discussion
Our results are indicative of testing up to 20% and 40% strain at strain rate regimes around 1118 ± 56 s−1 and 2553 ± 281 s−1, respectively (). The experimental data showed a consistent material response characterized by a J-shaped, monotonically upward, non-linear stress-strain behavior (Tappert et al. Citation2017). This increasingly marked J-shaped behavior with increased testing strain rate is consistent with previous findings, as it confirms the strong strain-hardening and strain rate sensitivity characteristics of the TMJ disc material. Depending on the sample, our results suggested a 6- to 20-fold increase in strength at 20% strain, when the strain rate increased from 1118 ± 56 to 2553 ± 281 s−1.
Figure 1. TMJ stress-strain behavior at two strain rate regimes. The photograph inserts depict the compression of a sample prior to and at the end of the testing. A Mooney Rivlin model (MR model) is fitted on the experimental data and average parameters’ values allowed plotting trend curves (solid and dashed black lines).
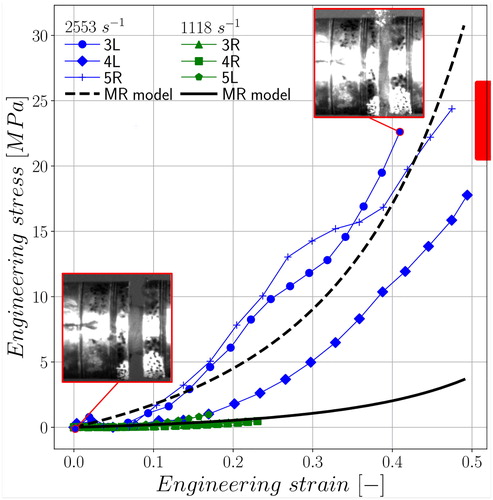
Our results also showed the presence of a ‘kink’ (e.g., a sudden decrease in stiffness followed by a recovery) at ∼25% strain in the 3 L and 5 R stress-strain responses (). As opposed to the high strain rate data, the low strain rate curves did not show ‘kink’ (20.5 ± 0.3% maximum average strain).
Based on their strain rate history (), we hypothesized that this was an indicator of partial failure inception and permanent damage upon compression beyond 25% strain. Further histology and microstructure analysis post-loading are required to confirm our hypothesis. The hyperelastic model fitting highlights the consistency of the Mooney Rivlin model (). Interestingly the parameters values were lower as compared to already reported data in previous high rate FE models (Pérez del Palomar and Doblaré Citation2008). The shear moduli obtained were 4.73 ± 1.41 MPa for the 2553 ± 281 s−1 strain rate and 0.56 ± 0.31 MPa for the 1118 ± 56 s−1 one. Considering an incompressible material with a Poisson’s ratio of 0.5, these results lead to a Young’s modulus ranged between 1.68 and 14.22 MPa. Being in the literature range, these values appeared higher than recorded in quasi-static testing (Tappert et al. Citation2017) owing to the visco-elastic characteristics of the tissue (Fazaeli et al. Citation2019).
4. Conclusions
For the first time, our study introduces the mechanical stress-strain behavior of TMJ discs at high strain rates. Based on experimental data, we computed initial hyperelastic model parameter values to be used in simulation taking also into account the material viscous behavior. Further ex vivo testing is warranted on TMJ discs preserved in the jaw in order to further characterize its mechanical properties along with any tissue damages and changes in microstructure upon impact. This proof of concept opens a new window to address fundamental questions regarding the actual behavior and modeling of TMJ discs in trauma.
Acknowledgements
The authors would like to acknowledge the support the Royal British Legion Centre for Blast Injury Studies at Imperial College London.
References
- Fazaeli S, Ghazanfari S, Mirahmadi F, Everts V, Smit TH, Koolstra JH. 2019. The dynamic mechanical viscoelastic properties of the temporomandibular joint disc: The role of collagen and elastin fibers from a perspective of polymer dynamics. J Mech Behav Biomed Mater. 100:103406.
- Pérez del Palomar A, Doblaré M. 2008. Dynamic 3D FE modelling of the human temporomandibular joint during whiplash. Med Eng Phys. 30(6):700–709.
- Sory DRR. 2017. Dynamic loading of periosteum-derived mesenchymal stromal cells [PhD thesis]. London: Imperial College.
- Tappert LK, Baldit A, Rahouadj R, Lipinski P. 2017. Local elastic properties characterization of the temporo-mandibular joint disc through macro-indentation. Comput Methods Biomech Biomed Engin. 20(Sup1):201–202.