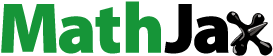
1. Introduction
The biomechanical study of the body center of mass (BCoM) may reveal crucial information about gait impairment in people with amputation (Al Abiad et al. Citation2020). The 3D kinetics of the BCoM are usually acquired with either force plates or the combination of inertial models and optical motion capture system (OMCS), generally not available in clinical routine (cost, set-up time, …). Therefore, the use of magneto-inertial measurement units (MIMUs) has been proposed as a wearable alternative for the estimation of BCoM-derived parameters. While single-sensor approaches may not be accurate enough for pathological gait, limiting the number of MIMUs is essential (Ancillao et al. Citation2018). Shahabpoor et al. (Citation2018) recently proposed a method to select a reduced number of MIMUs for the estimation of 3D BCoM acceleration. While the need to consider the 3D nature of BCoM movement has been widely acknowledged (Minetti et al. Citation2011), the method was validated using MIMU signals only for the vertical component of the acceleration and in an asymptomatic population. To overcome this limitation, a MIMU-based framework to obtain accurate 3D BCoM acceleration is proposed and validated on one person with transfemoral amputation.
2. Methods
2.1. Framework overview
The developed framework can be divided in three steps aiming at: i) computing the 3D acceleration of each segment’s center of mass (sCoM) from MIMUs data, ii) merging sCoM accelerations in a consistent common global frame and iii) estimating the 3D BCoM acceleration from a weighted average of selected sCoM accelerations. Adapting the approach from Shahabpoor et al. (Citation2018), the trunk, pelvis, thighs and feet were identified as the major contributors in 3D BCoM acceleration in people with transfemoral amputation. Therefore, 6 MIMUs, manually aligned with the longitudinal axes of the segments, are adopted.
Computing 3D sCoM acceleration: following Pillet et al. (Citation2010), a 15-segment subject-specific inertial model, personalized using calibrated photographs in a static posture, is used to obtain the sCoM positions in an OMCS reference frame
The MIMU positions are manually identified on the photographs, which allows to compute their relative position with respect to the sCoM (
) in
The transformation matrix from to
can be approximated at the beginning of each acquisition using the a priori known static posture and manual alignment of MIMUs on the segments. This approximation is corrected using the accelerometers of MIMUs as inclinometers.
It is then possible to express and to compute sCoM accelerations in
as follows:
where
and
are respectively the acceleration and the angular velocity measured by the MIMUi in the sensor frame
and
is obtained using a 5-point stencil differentiation.
Merging sCoM accelerations in a consistent common global frame: since multiple synchronized MIMUs may sense different earth-fixed global frames
due to ferro-magnetic disturbances (Picerno et al. Citation2011),the global frame sensed by the pelvis MIMU is chosen as the common global reference frame
The constant transformation matrix
between each MIMU global frame
and the common global reference frame
is obtained during the static posture of each acquisition using the orientation in
of both the pelvis MIMU
and the MIMUi
as well as the MIMUi orientation output (
Estimating 3D BCoM acceleration: sCoM accelerations expressed in
are used to compute the BCoM acceleration, with
and
being the masses of the ith segment and of the whole body derived from the personalized inertial model:
2.2. Framework implementation
2.2.1. Experimental protocol
One male individual with transfemoral amputation (mass: 83 kg, stature: 1.69 m) gave his written informed consent to participate in the study. He was instrumented with a full-body marker set (Al Abiad et al. Citation2020) and 6 MIMUs (Xsens, 100 Hz) on the feet, thighs, pelvis, and trunk, each mounted on a 3D-printed plastic support with housings for 4 reflective markers. An OMCS (VICON, 200 Hz) recorded the markers’ positions while 4 photographs (front, back, both sides) were taken. Then, starting from a static standing posture, the participant walked at self-selected speed along an 8 m pathway, with 3 force plates (AMTI, 1000 Hz) in the middle. Synchronization between instruments was achieved by a trigger signal. Only trials with three successive foot contacts on the force plates (i.e., a complete stride), were considered for further analysis.
2.2.2. Data processing
Data were filtered using a zero-phase 4th-order Butterworth filter (cut-off frequencies identified using a trial-and-error approach: 5 Hz markers and MIMUs, 10 Hz force plates). Reference sCoM accelerations were obtained by double derivation of OMCS-based sCoM positions. Reference BCoM acceleration was computed from force plates’ signals. Reference and MIMU-based sCoM and BCoM accelerations were compared using Pearson’s correlation coefficient ρ and peak-to-peak normalized root-mean square errors (NRMSE) averaged over the trials.
3. Results and discussion
Seven strides were analyzed. Similar results were obtained for sCoM and BCoM accelerations () in terms of accuracy, with BCoM results in agreement with those reported in healthy subjects using OMCS-based accelerations (Shahabpoor et al. Citation2018). High consistency between reference and MIMU 3D BCoM acceleration patterns is also observed ().
Table 1. Segment (sCoM) and body center of mass (BCoM) accelerations (acc.) accuracy and correlations with respect to reference values.
These results are encouraging and suggest that MIMUs may be a valid alternative to lab-based instruments when the 3D BCoM acceleration is targeted. The accuracy of derived parameters (internal power or BCoM trajectory) remains to be investigated. It has to be noted that the developed framework relies on an OMCS for photograph calibration. To be fully wearable, the inertial model could be directly built by fitting volumes on face and profile photographs, calibrated using objects of known dimensions.
4. Conclusions
The framework’s results are encouraging, even if they need to be confirmed on a larger cohort. Future studies will aim at assessing i) the accuracy achieved when the calibration frame is defined without an OMCS and ii) the impact of MIMU misplacement.
Acknowledgements
Study financed by the Fondation des Amputés de Guerre de France and the Université Franco-Italienne.
Disclosure statement
No potential conflict of interest was reported by the authors.
References
- Al Abiad N, Pillet H, Watier B. 2020. A mechanical descriptor of instability in human locomotion: Experimental findings in control subjects and people with transfemoral amputation. Appl Sci. 10(3):840.
- Ancillao A, Tedesco S, Barton J, O’Flynn B. 2018. Indirect measurement of ground reaction forces and moments by means of wearable inertial sensors: A systematic review. Sensors. 18(8):2564.
- Pillet H, Bonnet X, Lavaste F, Skalli W. 2010. Evaluation of force plate-less estimation of the trajectory of the centre of pressure during gait. Comparison of two anthropometric models. Gait Posture. 31(2):147–152.
- Picerno P, Cereatti A, Cappozzo A. 2011. A spot check for assessing static orientation consistency of inertial and magnetic sensing units. Gait Posture. 33(3):373–378.
- Minetti AE, Cisotti C, Mian OS. 2011. The mathematical description of the body centre of mass 3D path in human and animal locomotion. J Biomech. 44(8):1471–1477.
- Shahabpoor E, Pavic A, Brownjohn JMW, Billings SA, Guo LZ, Bocian M. 2018. Real-life measurement of tri-axial walking ground reaction forces using optimal network of wearable inertial measurement units. IEEE Trans Neural Syst Rehabil Eng. 26(6):1534–4320.