1. Introduction
Cancer has remained one of the most indomitable enigmas for scientists over centuries and despite decades of research, it remains a colossal global threat (Chowdhury et al. Citation2016). Tumor cells have lower stiffness compared with normal ones (Cross et al. Citation2007), due to the requirement of cancer cells to migrate through tissues and adapt to changing environments, becoming softer as they pass from blood to new tissues. For this reason, the study of the mechanical properties of cancer cells (Abidine et al. Citation2015, Citation2018) has been a starting point to overcome the limitations of modern cancer therapies with the ultimate goal of predicting tumor growth, making diagnosis and personalized therapy. In this study, 3D tumor spheroids (Delarue et al. Citation2014; Guillaume et al. Citation2019) are used. They tend to grow in close contact, reproducing physical communications and signaling pathways observed in solid tumors. Growth can be divided into two main stages: in the initial growth period, the volume increases exponentially, and this is known as the avascular growth. Afterwards, the vascular growth phase begins with the formation of new vessels induced by the secretion of pro-angiogenic factors and ECM remodeling. A similar growth pattern is found in spheroids. Here we present the first viscoelastic results on tumor spheroids as measured using our microrheology technique (Abidine et al. Citation2015) adapted for spheroids. Moreover, several experiments were carried out observing the role of spheroid size which is important in tumor growth.
2. Methods
2.1. Cells and spheroids
T24 (epithelial bladder cancer cells) cells were grown in complete culture medium RPMI 1640 (Gibco, Saint Aubin, France) supplemented with 10% SBF and 1% penicillin/streptomycin). Around 5.0 105 cells in 1.5 mL medium were then seeded into Petri dishes covered with agarose gel (1.5%). Cells packing was observed and spheroids were formed after a few days. After that, the rate of volume growth started to decrease, reaching the spheroid growth plateau (Van Dijk et al. Citation2013). Spheroids in culture medium (including 1% HEPES 1 M) were set in a Petri dish at 37 °C ready for AFM and spred on the bottom plastic surface.
2.2. Microrheology
Atomic Force Microscopy (AFM) allows indenting samples in order to evaluate their mechanical properties. AFM is an interesting tool used to investigate live cells (Abidine et al. Citation2015, Citation2018) and provides accurate small force measurements (F) combined with nanometric displacements converted into indentation values (δ). This provides classical curves F(δ) allowing determination of the elastic modulus E, using Hertz model. In this study, the analysis of mechanical properties of tumor spheroid was achieved in oscillatory regime, following an initial indentation δ0. This gives the complex shear modulus G*(ω)=G’(ω)+iG’’(ω)=(1-ν)/4/(Rδ0)1/2{F*/δ*-iωb(0)}, where F* is the complex force, δ* the complex displacement, b(0) the drag coefficient, ν the Poisson coefficient and R is the spheroid radius. Force measurements in liquids are subjected to the hydrodynamic drag force, and b(0) involves calibrating at different distances which are extrapolated when the cantilever gets close to the sample (Alcaraz et al. Citation2003). Stiff cantilevers (Nanosensors, TL-NCL model) were chosen with stiffness around 30 N/m and resonant frequency f0 ∼ 150 kHz.
3. Results and discussion
Spheroids mechanical properties – prepared under the same conditions – were compared in order to estimate the role of size, keeping the other parameters constant. An approach setpoint of 300nN was used generally, giving indentations in the range 1–6µm. shows the setup with the spheroid adhering at the bottom of the Petri dish, and the cantilever coming from above. The hydrodynamic drag was estimated at different heights (0.5–1–2–3–5µm) and extrapolated to give b(0)=3.45 10−5 N.s.m−1. Finally, corrected values of viscous and elastic moduli were computed. The results are shown in for four different spheroids (single measurement), where viscoelastic moduli G’(f) and G”(f) are plotted.
Figure 1. Phase contrast image of the spheroid (size ∼ 200 µm) with AFM cantilever (Nanosensors, TL-NCL, length 225 µm) on top.
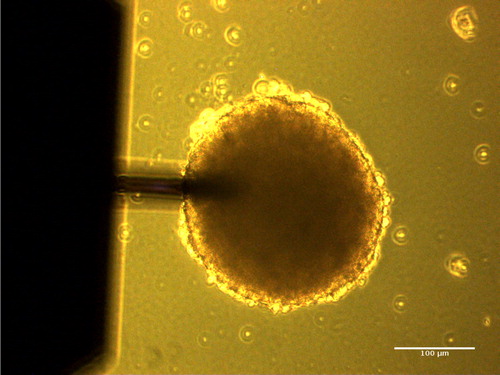
Figure 2. Moduli G’(f) and G”(f) as a function of frequency f (Hz) for different spheroids of various sizes.
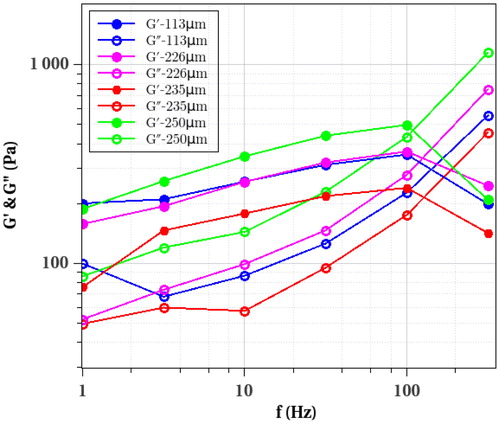
There is some variability in the data, due to cell differences, as classically observed with cell measurements. The effect of size does not seem to matter so much on the data, since the smallest spheroid (diameter of 113 µm) does not show important differences with the largest spheroid (diameter of 250 µm). Altogether, the viscoelastic data gives elastic moduli G’ in the range 100–500 Pa with an important viscous component G”, this data being similar to previous one obtained with cell aggregates using a classical rheometer (Iordan et al. Citation2008). Finally, G’ and G” were also evaluated changing the approach force setpoint (between 100–400 nN). Results showed that applying too small setpoints are not within the accuracy limit, and that too high setpoints are in the elasticity nonlinear range. Therefore reasonable values of δ0 in the range 3–6µm are required to obtain such relevant data.
4. Conclusions
By developing this AFM-based microrheology technique, information on the viscoelastic behavior can be reported and compared for 3D tumor spheroids of different sizes. This information can be coupled with viscoelastic models to extract parameters related to spheroids viscoelasticity. This opens a new methodology to explain physiological or pathological mechanisms occurring during biological processes. Furthermore, the effect of ECM in spheroids will be investigated and the analysis of tumor spheroids prepared under different conditions can be considered in the next future.
Acknowledgements
The authors thank the Nanoscience foundation for support of the AFM.
Disclosure statement
No potential conflict of interest was reported by the authors.
Additional information
Funding
References
- Abidine Y, Constantinescu A, Laurent VM, Rajan VS, Michel R, Laplaud V, Duperray A, Verdier C. 2018. Mechanosensitivity of cancer cells in contact with soft substrates using AFM. Biophys J. 114(5):1165–1175.
- Abidine Y, Laurent V, Michel R, Duperray A, Verdier C. 2015. Local mechanical properties of bladder cancer cells measured by AFM as a signature of metastatic potential. Eur Phys J Plus. 130:202.
- Alcaraz J, Buscemi L, Grabulosa M, Trepat X, Fabry B, Farré R, Navajas D. 2003. Microrheology of human lung epithelial cells measured by atomic force microscopy. Biophys J. 84(3):2071–2079.
- Chowdhury RM, Schumann C, Bhakta-Guha D, Guha G. 2016. Cancer nanotheranostics: Strategies, promises and impediments. Biomed Pharmacother. 84:291–304.
- Cross SE, Jin Y-S, Rao J, Gimzewski JK. 2007. Nanomechanical analysis of cells from cancer patients. Nat Nanotechnol. 2(12):780–783.
- Delarue M, Montel F, Vignjevic D, Prost J, Joanny J-F, Cappello G. 2014. Compressive stress inhibits proliferation in tumor spheroids through a volume limitation. Biophys J. 107(8):1821–1828.
- Guillaume L, Rigal L, Fehrenbach J, Severac C, Ducommun B, Lobjois V. 2019. Characterization of the physical properties of tumor-derived spheroids reveals critical insights for pre-clinical studies. Scientific Rep. 9:6597.
- Iordan A, Duperray A, Verdier C. 2008. Fractal approach to the rheology of concentrated cell suspensions. Phys Rev E Stat Nonlin Soft Matter Phys. 77(1 Pt 1):011911.
- Van Dijk M, Göransson SA, Strömblad S. 2013. Cell to extracellular matrix interactions and their reciprocal nature in cancer. Exp Cell Res. 319(11):1663–1670.