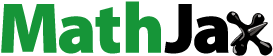
Abstract
Skid resistance of road surfaces is an important safety parameter. Decreasing skid resistance correlates with increasing number of accidents. The presented study aims for a correlation between lab-based polishing simulation and skid resistance measurement and evolution of the skid resistance under traffic in the field. A prediction model for skid and polishing resistance based on the Wehner/Schulze device was developed. The lab procedure as a basis for the model works on the hypothesis that a maximum level of skid resistance exists that can be regenerated even from a highly polished state by sandblasting of the surface. This hypothesis was verified for an asphalt and concrete surface material. The prediction model was set up by correlating field data on skid resistance and traffic volume from 14 test sections to cores taken from these test sections and tested in the lab. A linear regression links the cumulated traffic volume in the field with the number of polishing passes in the Wehner/Schulze device. Thus, it is possible to simulate millions of wheel passes within a couple of hours in the lab to generate equal skid resistance level and predict the skid resistance level of a road surface after years under traffic.
1. Introduction
The skid resistance of road surfaces has a crucial impact on road safety in general (Hatherly et al. Citation1969). Various studies show significant correlations between the skid resistance of surface layers and the number of road accidents (Kuttesch Citation2004, Davies et al. Citation2005, Kudrna et al. Citation2006). This brings the need for providing road surfaces with both, a high initial skid resistance and resistance to polishing due to climate and traffic to ensure long-lasting friction values on roads and therefore, sufficient safety for users.
Continuous monitoring of the skid resistance in the field can be carried out by different devices. Commonly used are the SCRIM (Side force Coefficient Routine Investigatory Machine) (Oliver Citation2009, Echaveguren et al. Citation2010), the Skiddometer (Leandri and Losa Citation2015), or the GripTester (Flintsch et al. Citation2009; Najafi et al. Citation2013). For the assessment of the polishing and skid resistance in the laboratory, the polished stone value (PSV) method has been in use for decades (Perry et al. Citation2001, Erichsen Citation2009, Shabani et al. Citation2013, Perry Citation2014, Dokic et al. Citation2015). However, the PSV method is limited to the coarse aggregate fraction and does not take into account the actual surface texture of a road pavement including the binder component, filler and fine aggregates. The Wehner/Schulze device, developed in the 1960s in Germany (Wehner Citation1968, Schulze Citation1968), is a combined test stand for simulating polishing and skid resistance measurement on flat, circular surfaces and can thus be employed for studying arbitrary surfaces, including aggregates, but also actual road surface layers from field cores or lab produced samples. The Wehner/Schulze device has been increasingly used in research in recent years to study skid resistance of aggregates, surface layers and correlations between lab and field measurements (Do et al. Citation2007, Kane et al. Citation2008, Wang, Yin, et al. Citation2011, Wang, Chen, et al. Citation2015, Wang, Xie, et al. Citation2015, Kane et al. Citation2013).
In recent years, research studies tend to work towards correlation between laboratory assessment of skid and polishing resistance and the actual skid resistance situation in the field incorporating information on aggregate mineralogy, surface texture, traffic and climate situation into the studies (Panagouli and Kokkalis Citation1998, Do, Tang, et al. Citation2007, Asi Citation2007, Do, Kane, et al. Citation2009, Seneviratne et al. Citation2009, Kane et al. Citation2012). The main objectives of these studies are to understand the impact of employed materials and field conditions on the evolution of the skid resistance on the one hand, and how to simulate these conditions in a realistic way in the laboratory on the other hand.
The study presented in this paper aims to contribute to the work on correlating laboratory and field measurements of skid and polishing resistance by developing a prediction model (PROGRIP) for the skid and polishing resistance based on the Wehner/Schulze device and actual traffic data from the field.
1.1. Motivation and objectives
The present situation in many road design guidelines, e.g. (FSV Citation2015), is to set requirements regarding the skid and polishing resistance of the coarse and/or fine aggregate fraction of a surface layer in identification testing, mostly by PSV testing. For acceptance testing, the actual skid resistance of the surface layer in the field is measured. In addition, a minimum remaining skid resistance at the end of the warranty period required to ensure sufficient polishing resistance. However, skid resistance quality of the aggregate fraction in the lab does not necessarily correlate with the skid resistance of a surface layer in the field. This is especially true for more recently developed materials, e.g. high rate use of recycling material for asphalt mixtures.
To overcome this obstacle, a recently completed research project (Kugler et al. Citation2015) worked towards two main objectives:
• | Analyse correlations between skid resistance measurements of road surfaces in the lab by the Wehner/Schulze device and in the field using RoadSTAR. The RoadSTAR is a skid resistance measurement device for routine monitoring on network level used in Austria (FSV Citation2004) based on a measurement wheel with fixed slip. | ||||
• | Develop a lab procedure based on the Wehner/Schulze device to predict the evolution of the skid resistance under traffic in the lab and calibrate it to field data. |
This paper concentrates on the second objective of the study. The outcomes of the study allow for a realistic prediction of the skid resistance on lab scale. Compared to full scale measurements on the road, the presented approach is a quick and easy method with good correlation to field measurement.
For the study, the following steps were taken to set up the model:
• | Selection of 14 representative test sections (bitumen and cement bound surface layers) on the high level road network | ||||
• | High resolution skid resistance measurements in the field by RoadSTAR | ||||
• | Sampling of the test sections by coring | ||||
• | Development of the model by adapting sandblasting and polishing simulation for the Wehner/Schulze device | ||||
• | Run the approach on cores from the test sections to correlate the procedure to traffic volume data from the test sections |
2. Materials & test devices
2.1. Surface layers
The Austrian highway network consists of a total length of about 2200 km, one third of the network is constructed with concrete pavement structures, the other two thirds with bituminous bound mixtures. Regarding surface layers, the majority of the bituminous bound surface layers is made of stone mastic asphalt with a maximum nominal aggregate size of 11 mm (SMA 11) and a nominal void content ranging from 3 to 6 Vol.%. Concrete pavements have been carried out as exposed aggregate concrete surface with a maximum nominal aggregate size of 8 or 11 mm (EACS 8 and EACS 11) exclusively since the early 1990s. For these reasons, the study concentrated on SMA 11 and EACS 8.
2.2. Skid resistance measurement in the field – RoadSTAR
The RoadSTAR skid resistance measurement device (Figure ) measures the longitudinal skid resistance of a pavement in the right wheel path using a modified Stuttgarter skiddometer. It uses a PIARC ribbed tire with a controlled vertical load of 3500 N (CEN Citation2009). The skid resistance is determined using a fixed slip of 18% and a water film of 0.5 mm. Measurements are carried out in fluent traffic with a standard speed of 60 km/h. For the speed range of 30 to 60 km/h, the measurement results can be converted to the standard speed to allow for speed variation due to traffic. The braking torque and vertical load are recorded in intervals of 0.1 m travelled distance and the skid resistance coefficient μfield is calculated as follows:(1)
(1)
Figure 1. Routine monitoring device RoadSTAR (left); detail of the skid resistance measurement unit (right).
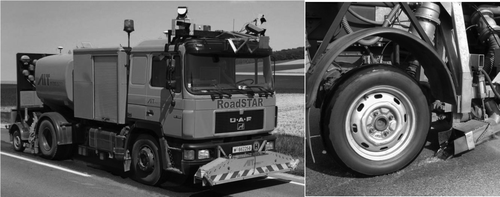
where; μfield Skid resistance coefficient [–]; MB Braking torque [Nm]; r radius of measurement tire [m]; FN vertical load [N].
The measurement simulates a full braking of a passenger car (similar slip and vertical load) on a wet pavement. From the measured skid resistance, achievable decelerations of braking passenger cars and thus braking distances can be calculated (Maurer Citation2008).
2.2. Skid resistance measurement in the lab – Wehner/Schulze device
The Wehner/Schulze device (Figure ) combines polishing and skid resistance measurement in one test stand. Any flat, circular surface with a diameter of 225 mm can be tested. The polishing is carried out by a rotating head with three rubber covered conical rollers rotating at 500 rpm on the specimen surface (left in Figure ). Thus, 90,000 polishing passes can be applied per hour. To increase the polishing effect, a water-quartz powder slurry is added. After any user-defined number of polishing passes, the polishing can be stopped, the test specimen is washed to remove remaining quartz powder and the skid resistance is measured using a measurement head with three testing rubbers (right in Figure ). For the skid resistance measurement, the rotating head is accelerated to a tangential speed of 100 km/h in a first step. Subsequently, water is sprayed onto the specimen surface before the rotating head is lowered onto the surface. As the test head decelerates the friction value μ is recorded. According to the European standard procedure (CEN Citation2014), the friction value at 60 km/h is reported as the μPWS (Polishing value Wehner/Schulze). This value is used within this study as well to describe the skid resistance of lab tested specimens.
Presently, there are about 20 Wehner/Schulze devices in operation throughout Europe.
Although both devices differ in terms of measurement principle (slip level), contact area and temperature conditions, a high correlation between friction measurement in the field by RoadSTAR and in the lab by the Wehner/Schulze device on cores can be observed. A detailed study on this issue can be found in (Hofko et al. Citation2017).
3. Approach
3.1. Test sections
Since 1999, routine skid resistance surveys are carried out on the Austrian highway network in 5-year intervals. In addition, acceptance testing and testing at the end of the warranty period is carried out on all newly constructed or resurfaced sections since 2004. From these measurements, a total of 4000 data sets with a total length of measurement of 13,000 km are available. They were the basis for selecting suitable test sections for the presented study. For pre-filtering, only sections meeting the following requirements were taken into consideration:
• | Surface layer SMA 11 or EACS 8 | ||||
• | Average annual daily traffic (AADT) > 20,000 and share of heavy goods vehicles (HGV) > 14% of AADT | ||||
• | Linearity of the road axis: no horizontal curvature, max. slope ±1% | ||||
• | Homogeneity of the skid resistance derived from RoadSTAR data to avoid outliers when sampling |
From this pre-filtering, 60 sections remained to be considered for sampling. Taking into consideration additional criteria for the test sections, i.e. age of the surface layer and evolution of the skid resistance with time, 14 test sections were finally selected, seven of them being SMA 11 and seven EACS 8. The year of construction or resurfacing varies from 1994 to 2012.
3.2. Skid resistance measurements on the test sections
The standard resolution of skid resistance measurements by the RoadSTAR are 50 m values. To ensure that all samples are cored from a homogeneous region regarding the skid resistance, high resolution measurements were carried out using 1 m values for all 14 test sections. The measurements were run on the right wheel path of the main lane, as well as on the emergency lane to receive skid resistance data for the polished, as well as for the non-polished part of the surface layer. Based on the skid resistance data, optimal spots for coring were determined.
3.3. Sampling
Cores were taken from the right wheel path of the main lane to receive polished samples. In addition, unpolished samples were taken either from the emergency lane or, for those sections, where the emergency lane was not constructed with the same surface layer as the main lane, from between the wheel paths of the main lane. It is known that the age of the binder film on the surface layer has a significant impact on the pavement friction (Kane et al. Citation2010, Citation2013, Lopes et al. Citation2014).The developed approach (see Section 3.4.4) consists of sandblasting the samples before applying the prediction model and therefore removing the surface binder film. Thus, the impact of the surface binder film seems to be of minor relevance for the presented approach.
From each test section, 4 polished and 4 non-polished samples were cored for further analysis in the lab.
3.4. Developing the procedure
3.4.1. Initial approach
The initial approach was based on the most obvious idea: Both, the polished and non-polished samples from each test section i would be tested in the Wehner/Schulze device for skid resistance to obtain the friction values μ for the initial condition before traffic (μ0,i, non-polished) and after a certain traffic volume (μp,field,i polished samples). Subsequently, each non-polished sample would be polished in the Wehner/Schulze device until the friction value of the lab-polished sample μp,lab,i would equal the polished field sample μp,field,i. With the information on the necessary number of polishing passes in the Wehner/Schulze to reach μp,lab,i and the cumulative AADTi for each test section i, a correlation analysis would produce a relationship between the traffic loading in the field (cumulative AADTi) and the number of polishing passes in the Wehner/Schulze device. This approach, however, was not successful due to the following reason: In many cases (6 out of 14), the emergency lane was not constructed in the same way as the main lane. Thus, most of the non-polished samples had to be cored from between the wheel paths on the main lane. First skid resistance testing in the lab showed that these samples could not be considered as non-polished, since the friction value of these samples did not differ significantly from the values of samples cored from the wheel path. Therefore, a more complex approach was needed.
3.4.2. Approach based on maximum skid resistance level
The hypothesis for the new approach was that a level of maximum (specimen- and material-specific) skid resistance μmax,lab exists that can be reproduced even from a highly polished state by sandblasting the surface and therefore, restore a certain, artificial non-polished surface texture. This would allow the study to bring all non-polished cores, even those taken from between the wheel paths, to a comparable and well-defined initial skid resistance level. The polishing simulation could then be carried out from this initial skid resistance level for each of the test sections regardless of the polishing state of the cores.
To verify this hypothesis, a preliminary test program was carried out on industrially produced granite floor tiles. The reason for using floor tiles instead of road surfaces was the higher homogeneity and planarity of floor tile surfaces and thus, a better reproducibility of results. Floor tiles were sandblasted in a standard sandblasting cabinet according to (CEN Citation2014). For blasting, corundum 0.5/1 mm was used. The nozzle has an outlet of 6.3 mm diameter and works with an air pressure of 5.0 bar. The distance between nozzle and the specimen is 63 mm and the angle between direction of the blasting and the specimen surface is 75°. An automatically rotating table in the cabinet ensures that the surface is uniformly blasted after 110 sec. The following test program was run on the floor tiles:
• | Sand blasting according to the parameters described above | ||||
• | Rinsing the surface to remove remaining corundum | ||||
• | Skid resistance measurement in the Wehner/Schulze device to obtain the initial friction value μ0, lab | ||||
• | Adding 1500, 4000 and 9000 polishing passed on the surface with intermediate skid resistance measurements to obtain μk,lab, where k is the total number of polishing passes |
Figure shows results of this preliminary test program. The diagram contains the μk,lab values for each sandblast and polishing cycle. A total of 16 cycles was run on the specimen. As it can be seen from the data, after 4 cycles of sandblasting and polishing, the specimen reaches a maximum level of skid resistance after sandblasting. This maximum level is constant from cycle nine on. From this data, it can be concluded that a maximum skid resistance μmax,lab can be realised by the described method. In addition, μmax,lab cannot only be reached once, but repeatedly after each polishing cycling. The data for μmax,lab shows a certain scattering after each cycle. When cycles nine to 16 are taken into account, the standard deviation for μ0,lab is 0.006. This scattering lies within the precision of the Wehner/Schulze skid resistance measurement device.
Figure 3. Results of 16 sandblast and polishing cycles on a floor tile with skid resistance measurements after 1500; 4000 and 9000 polishing passes.
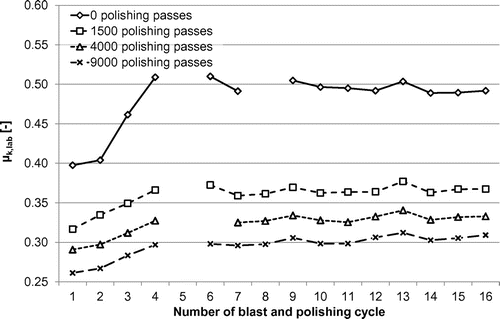
Another interesting point that can be shown from the diagram in Figure is that the four curves, which represent μ0,lab, μ1500,lab, μ4000,lab and μ9000,lab, run in parallel. This means that the skid resistance after equal polishing work depends strongly on the initial skid resistance value μ0,lab. In addition, it can be concluded that after the maximum skid resistance level is reached, same polishing work will lead to repeatable skid resistance levels on the same specimen. When the difference between μ0,lab and μ9000,lab is analysed, the standard deviation of this difference is 0.007 (data from cycles nine to 16 taken into account).
If these findings could be transferred from industrially produced floor tiles to actual road surfaces, then not only non-polished samples from the field or the lab could be used for polishing simulation but also polished samples could be employed. The initial, artificial maximum skid resistance value could then be regenerated by sandblasting and even polished samples re-used for testing purposes in the same way as non-polished samples.
Regarding the quality of the corundum for blasting, it was found in this preliminary test program that the material has to be replaced after ten blasting cycles, since the corundum deteriorates and loses its abrasion ability.
To verify this approach on actual road surfaces, lab produced SMA 11 and EACS 8 were tested following the same procedure as described above for the floor tiles. Different from this procedure, the surface was polished for up to 180,000 polishing passes. Figure presents the results exemplarily for the SMA 11. The diamonds show the initial skid resistance value after sandblasting μ0,lab. For the SMA 11, more than one blasting cycle was necessary to obtain μmax,lab. Especially after applying a high amount of polishing work (180,000 passes), two to three blasting cycles were necessary to reach μmax,lab again. However, even after applying high polishing work, the maximum skid resistance level can be repeatedly obtained and the skid resistance after polishing is repeatable as well. This proves that the described approach based on the hypothesis of a regenerative maximum skid resistance level is also valid for actual road surfaces.
3.4.3. Adapting the polishing procedure
In the standard procedure according to (CEN Citation2014), the polishing agent in the Wehner/Schulze device is a slurry of quartz powder and water with a concentration of 6% (2.4 kg of quartz powder in 40 l of water). The polishing agent is used to increase the polishing performance and make the method more time efficient. However, the standard concentration is too severe to adequately simulate polishing of traffic in the field. Only a couple of thousand polishing passes equal millions of wheel passes. Since the Wehner/Schulze polishing simulation leads to a strong decrease of the skid resistance within the first few thousand polishing passes, small errors in the number of polishing passes would lead to large errors in the correlation with polishing by traffic.
Therefore, a parametric study was carried out to find a more suitable polishing agent for the procedure to be developed. Four different agents, namely
• | pure water, | ||||
• | 0.6% quartz powder in water (0.24 kg in 40 l), | ||||
• | 2% quartz powder in water (0.8 kg in 40 l) and | ||||
• | 6% quartz powder in water (2.4 kg in 40 l) |
were employed. Polishing and skid resistance testing was carried out on lab produced SMA 11 and EACS 8 specimens. For each polishing agent, four specimens were tested.
Figure shows the results (mean values) exemplarily for the SMA 11 specimens. The skid resistance μk,lab is shown vs. the number of polishing passes. Obviously, pure water is an insufficient polishing agent leading only to a small decrease after a high number of polishing passes (540,000). Even a low concentration of 0.6% quartz powder strongly increases the polishing performance. A statistical t-test carried out for all data sets shows that significant differences from the standard polishing agent (6%) can be achieved when the polishing agent is reduced to 0.6% quartz powder or when pure water is applied. However, since pure water is too weak to induce polishing efficiently, it was decided to work with a 0.6% quartz powder concentration as a polishing agent. This brings two main advantages:
• | The polishing performance is decreased to a level that allows for better correlation to polishing in the field under traffic. Still, the polishing performance is strong enough to ensure a time efficient method. | ||||
• | The quartz powder slurry needs to be replaced after 180,000 polishing passes to compensate for material loss and gradual rounding of the grains with increasing polishing. A reduction of the concentration to 1/10 of the standard is beneficial in terms of material handling and economic considerations. |
3.4.4. Standard lab procedure
Based on the findings and conclusions described above, a standard lab procedure was defined to carry out a serial examination on field samples from the 14 test sections and finally, setup a correlation between polishing in the lab and in the field. Table shows the standard procedure in an overview. Each non-polished core from the field is tested in three cycles. The three cycles differ in the number of sandblastings before running the polishing simulation on the specimen. The polishing simulation in each cycle runs up to 720,000 polishing passes with intermediate skid resistance measurements at defined intervals. Prior to any skid resistance measurement, specimen and testing rubbers are prepared by rinsing them for 5 min with water at 10 °C. This ensures that the viscoelastic properties of the testing rubbers and in case of bituminous bound mixtures, the surface, are repeatable.
Table 1. Standard procedure.
For C 3, at least 5 sand blastings are run on each specimen. The initial skid resistance μ0,n,lab is determined after each blasting, where n is the total number of blasting. The acceptable difference Δμ0,lab is defined as follows:(2)
(2)
where n ≥ 5. By calculating the difference between the initial skid resistance after blasting n–2 and blasting n, Equation (Equation2(2)
(2) ) ensures that the initial skid resistance has reached a constant level and is not affected by blasting anymore.
Per definition, μmax,lab is reached, when the following two criteria are fulfilled:
• | Δμ0,lab < 0.015 | ||||
• | Standard deviation of μ0,n,lab, μ0,n–1,lab and μ0,n–2,lab < 0.015 |
If both criteria are fulfilled after the 5th sandblasting, μmax,lab is reached. If one or both criteria are not fulfilled, more blastings are needed until both criteria are fulfilled. In this case, the maximum skid resistance μmax,lab is equal to μ0,n,lab.
4. Field correlation
To setup an actual prediction model for skid resistance, the standard lab procedure as described in Section 3.4 was carried out on at least 3 of the 4 non-polished samples from each of the 14 test sections. The samples were polished until the skid resistance of the polished lab sample μp,lab equaled the skid resistance of the polished field sample μp,field. The number of polishing passes to reach this skid resistance was recorded. Each sample was tested three times, i.e. with all three cycles defined in Table . Thus, at least 9 data sets are available for each of the 14 test sections and a total of at least 126 data sets overall for correlation analysis.
Table gives the relevant traffic data for the test sections. These data are the basis for the correlation between traffic loading and polishing passes in the lab. AADT data were extracted from automatic traffic counts. The cumulated traffic volume is the total number of vehicles that have passed the test sections since construction or resurfacing. AADT data was available from 2009 to 2014. To achieve the cumulated traffic volume, these data was extrapolated and integrated back to the construction date. The share of vehicles on the main lane is estimated based on a respective model (Sammer et al. Citation1999) from the AADT, number of lanes and lane width.
Table 2. Traffic data from all 14 test sections.
Table presents data from the lab procedure to simulate polishing in the lab by the Wehner/Schulze device. It shows the test section and surface type of the test section, as well as the mean value of μp,field from four polished specimens and the mean polishing passes for each cycle that are necessary to bring the non-polished specimens from the artificial maximum skid resistance level down to μp,lab that equals μp,field. In addition the standard deviation of polishing passes from all cycles and specimens are given for each test section. A complete set of data with all raw data included is provided exemplarily for test Section 2 under (Hofko Citation2016).
Table 3. Necessary polishing passes to achieve μp,lab = μp,field.
For the correlation analysis, the weighted cumulated traffic volume (CTVw,HGV) was taken into consideration using CTV and a weighing factor that includes the share of HGV. Equation (Equation3(3)
(3) ) shows how this weighted traffic volume was calculated:
(3)
(3)
Figure shows the correlation between CTVw,HGV and the mean value of polishing passes. A linear regression links both parameters. In addition, the 80, 90 and 95% upper prediction interval is shown as well. The linear regression equals
Figure 6. Correlation between weighted cumulated traffic volume and polishing passes including 80, 90 and 95% upper prediction intervals.
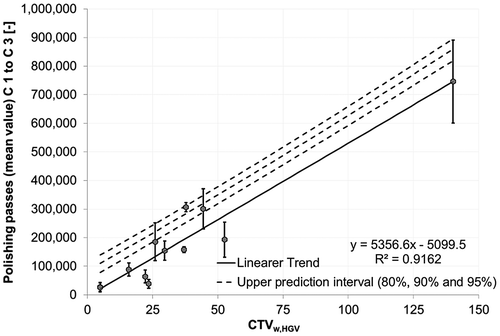
(4)
(4)
where PPC1–C3 is the number of polishing passes from cycle 1 to cycle 3.
The 80, 90 and 95% upper prediction intervals equal(5)
(5)
(6)
(6)
(7)
(7)
Equations (Equation4(4)
(4) )–(Equation7
(7)
(7) ) can be used to derive necessary polishing passes with higher statistical confidence for further application in the prediction procedure.
As it can be observed from the diagram, no distinction was made regarding the surface type. In other words, the correlation between polishing from traffic and the developed polishing simulation in the lab is valid regardless of the surface type. However, it must be noted at this stage that only two surface layer types, bituminous bound SMA 11 and cement bound EACS 8 are considered.
At the present point, the model is limited to road sections with linear axis, i.e. no curvature or slope higher than 1%. Also, it is limited to the climatic conditions in the region of the test sections, with warm, wet summers and cold, dry winters with more than 50 freeze/thaw cycles during the winter period.
4.1. Application
To give a practical example, a constant AADT of 40,000 vehicles/24 h is assumed with a 12.5% share of HGV (5000 HGV/24 h). If no traffic increase is assumed, this gives a CTV of 73 m vehicles 5 years after opening to traffic and a CTVw,HGV of 36.5. To simulate this traffic loading by the developed lab procedure, a mean value of 189,700 polishing cycles is necessary. For higher certainty of the procedure, the 80% percentile is 246,100, the 90% percentile is 277,300 polishing passes and the 95% percentile is 305,200 polishing passes. An SMA 11 or EACS 8 specimen that is tested according to the developed procedure listed in Section 3.4 can predict the remaining skid resistance after 5 years under traffic using these calculated polishing passes.
The results can be applied in different ways:
• | At the stage of mix design, the design can be optimised in the lab to meet certain skid resistance requirement at the end of the warranty period of e.g. 5 years with high certainty. | ||||
• | As a tool for identification and acceptance testing on lab scale, requirements for the minimum skid resistance after a certain cumulated traffic volume can be set. | ||||
• | In condition assessment, even polished cores from the field can be employed, since a regeneration of the maximum skid resistance level is possible. Thus, the developed procedure can be used to predict the remaining life-time of a surface layer before critical skid resistance values will be reached. This allows for a more efficient maintenance planning. |
5. Summary and conclusions
This paper presents the main outcomes of a study with the aim to develop a lab procedure (PROGRIP) based on the Wehner/Schulze device to predict the evolution of the skid resistance under traffic in the lab and to calibrate it to field data.
The lab procedure follows the hypothesis that a level of maximum skid resistance μmax,lab exists that can be reproduced even from a highly polished state by sandblasting the surface and therefore, restore a certain, artificial non-polished surface texture. This hypothesis was verified in a preliminary test program on industrially produced granite floor tiles and on SMA 11 and EACS 8 specimens afterwards. Thus, not only non-polished field samples could be used for polishing simulation but also polished samples could be employed and re-used for multiple polishing simulations since μmax,lab is regenerative.
The standard polishing procedure according to (CEN Citation2014) was adapted by reducing the quartz powder concentration in the slurry used to enhance the polishing performance to 1/10. Thus, the polishing performance is decreased to a level that allows for better correlation to polishing in the field under traffic.
The standard procedure was developed using samples (cores) from 14 test sections. The test sections had to fulfill certain requirements regarding the traffic volume, linearity of the axis and homogeneity of the skid resistance. Samples were taken from the right wheel path (polished samples) and from the non-polished part of the cross section.
Polished field samples were tested for skid resistance in the Wehner/Schulze device. Non-polished field samples were sandblasted to reach μmax,lab and polished to reach an equal level of skid resistance as the polished field samples. The necessary number of polishing passes was correlated to the cumulated traffic volume on the test sections. A linear regression links the number of polishing passes with the cumulated traffic volume with high coefficient of correlation (R² = 0.92). The regression is valid independently from the surface type, i.e. SMA 11 and EACS 8. Thus, the approach can be used to simulate field polishing in the lab in an efficient and economical way. Millions of wheel passes in the field can be applied in the Wehner/Schulze device within a couple of hours.
The approach can be employed at the stage of mix design to optimise the skid and polishing resistance of a surface. It can also be used for identification and acceptance testing on lab scale and for condition assessment of existing pavement surfaces for a more efficient maintenance planning.
At this stage, PROGRIP is based on two frequently used surface layers, an asphalt mixture SMA 11 and a concrete pavement EACS 8. It is limited to road sections with linear axis, i.e. no curvature or slope higher than 1%. Also, it is limited to the climatic conditions in the region of the test sections, with warm, wet summers and cold, dry winters with more than 50 freeze/thaw cycles during the winter period. In the future, the approach will be expanded by increasing the sample size, taking into account other surface types and special road sections, like ramps and curves with a stronger evolution of the skid resistance. In addition, aggregate mineralogy and seasonal variations due to impact of the climate will have to be taken into account for an extension of the developed approach. Also, the impact of sandblasting material on the achievable maximum skid resistance that can be regenerated, will be taken into consideration by future research.
Disclosure statement
No potential conflict of interest was reported by the authors.
Acknowledgments
The authors would like to express their gratitude to ASFINAG and Mr. Mario Krmek for the great support of the study by providing traffic data. In addition, the authors would like to express their gratitude to ASFINAG, the Austrian ministry of transportation (BMVIT), Gestrata, VOEZ, OEBA and OEBV for financing significant parts of this study.
References
- Asi, I.M. , 2007. Evaluating skid resistance of different asphalt concrete mixes. Building and Environment, 42 (1), 325–329.10.1016/j.buildenv.2005.08.020
- CEN , 2009. TS15901-1: road and airfield surface characteristics – Part 1: procedure for determining the skid resistance of a pavement surface using a device with longitudinal fixed slip ratio (LFCS): RoadSTAR. Brussels, Belgium: European Commission.
- CEN , 2014. EN 12697-49: Bituminous mixtures – test methods for hot mix asphalt – Part 49: determination of friction after polishing. Brussels.
- Davies, R.B. , Cenek, P.D. , and Henderson, R.J. , 2005. The effect of skid resistance and texture on crash risk. International surface friction conference, Christchurch, New Zealand.
- Do, M.T. , et al. , 2007. Pavement polishing – development of a dedicated laboratory test and its correlation with road results. Wear, 263, 36–42.10.1016/j.wear.2006.12.086
- Do, M.T. , et al. , 2009. Physical model for the prediction of pavement polishing. Wear, 267 (1–4), 81–85.10.1016/j.wear.2008.11.012
- Dokic, O. , et al. , 2015. Influence of engineering properties on Polished Stone Value (PSV): a case study on basic igneous rocks from Serbia. Construction and Building Materials, 101, 1088–1096.10.1016/j.conbuildmat.2015.10.033
- Echaveguren, T. , de Solminihac, H. , and Chamorro, A. , 2010. Long-term behaviour model of skid resistance for asphalt roadway surfaces. Canadian Journal of Civil Engineering, 37 (5), 719–727.10.1139/L10-021
- Erichsen, E. , 2009. Relationship between PSV and in situ friction: a Norwegian case study. Bulletin of Engineering Geology and the Environment, 68 (3), 339–343.10.1007/s10064-009-0193-2
- Flintsch, G.W. , et al. , 2009. Evaluation of international friction index coefficients for various devices. Transportation Research Record: Journal of the Transportation Research Board, 2094, 136–143.10.3141/2094-15
- FSV , 2004. RVS 11.06.65: skid resistance measurement by RoadSTAR. Vienna, Austria.
- FSV , 2015. RVS 08.97.05: technical contract conditions, requirements for bituminous mixtures. Vienna, Austria.
- Hatherly, L.W. , Mahaffy, J.H. , and Tweddle, A. , 1969. Skid resistance of city streets and road safety. Journal of the Institution of Highway Engineers, 16 (4), 7.
- Hofko, B. 2016. Raw data of PROGRIP research project [online]. Available from: http://tinyurl.com/zestqnt. [Accessed 27 February 2017].
- Hofko, B. , et al. , 2017. Correlating field and lab measurements of skid resistance by skiddometer and Wehner/Schulze device. Proceedings of the 96th Transport Reserach Board Annual Meeting, Washington, DC: Transport Research Board.
- Kane, M. , Do, M.T. , and Piau, J.M. , 2008. Modeling of the road surface polishing based on contact-mechanics approach. Road Materials and Pavement Design, 9, 305–318.10.1080/14680629.2008.9690171
- Kane, M. , et al. , 2010. Exploring the ageing effect of binder on skid resistance evolution of asphalt pavement. Road Materials and Pavement Design, 11, 543–557.10.1080/14680629.2010.9690346
- Kane, M. , et al. , 2012. Laboratory evaluation of aggregate polishing as a function of load and velocity. Application to the prediction of damages on skid resistance of road surfaces due to trucks and passenger cars. Road Materials and Pavement Design, 13 (2), 312–326.10.1080/14680629.2011.649424
- Kane, M. , Artamendi, I. , and Scarpas, T. , 2013. Long-term skid resistance of asphalt surfacings: Correlation between Wehner–Schulze friction values and the mineralogical composition of the aggregates. Wear, 303 (1–2), 235–243.10.1016/j.wear.2013.03.022
- Kane, M. , et al. , 2013. Development of an accelerated pavement test reproducing the effect of natural ageing on skid resistance. Road Materials and Pavement Design, 14 (1), 126–140.10.1080/14680629.2012.749804
- Kudrna, J. , et al. , 2006. Road skid resistance influence on the number of crash accidents. International Conference on Safe Roads, Budapest, Hungary.
- Kugler, H. , Hofko, B. , and Spielhofer, R. , 2015. Prognose der Griffigkeit von Fahrbahnoberflächen – Entwicklung eines Labor-Verfahrens – PROGRIP [Prediction of skid resistance of road surfaces - Development of a laboratory procedure - PROGRIP]. Vienna: Austria.
- Kuttesch, J.S. , 2004. Quantifying the relationship between skid resistance and wet weather accidents for Virginia data. Virginia Polytechnic Institute and State University: Blacksburg, VA.
- Leandri, P. and Losa, M. , 2015. Peak friction prediction model based on surface texture characteristics. Transportation Research Record: Journal of the Transportation Research Board, 2525, 91–99.10.3141/2525-10
- Lopes, M. , et al. , 2014. Characterisation of ageing processes on the asphalt mixture surface. Road Materials and Pavement Design, 15 (3), 477–487.10.1080/14680629.2014.922656
- Maurer, P. , 2008. The new Austrian skid resistance evaluation background based on the correlation of skid resistance values μRoadSTAR and braking decleration of passenger cars. 6th Symposium on pavement surface characteristics: SURF 2008, Portoroz, Slovenia.
- Najafi, S. , Flintsch, G.W. , and McGhee, K.K. , 2013. Assessment of operational characteristics of continuous friction measuring equipment (CFME). International Journal of Pavement Engineering, 14 (8), 706–714.10.1080/10298436.2012.667097
- Oliver, J.W.H. , 2009. Factors affecting the correlation of skid-testing machines and a proposed correlation framework. Road & Transport Research, 18 (2), 39–48.
- Panagouli, O.K. and Kokkalis, A.G. , 1998. Skid resistance and fractal structure of pavement surface. Chaos Solitons & Fractals, 9 (3), 493–505.10.1016/S0960-0779(97)00085-4
- Perry, M.J. , 2014. Role of aggregate petrography in micro-texture retention of greywacke surfacing aggregate. Road Materials and Pavement Design, 15 (4), 791–803.10.1080/14680629.2014.923781
- Perry, M.J. , Woodside, A.R. , and Woodward, W.D.H. , 2001. Observations on aspects of skid-resistance of greywacke aggregate. Quarterly Journal of Engineering Geology and Hydrogeology, 34, 347–352.10.1144/qjegh.34.4.347
- Sammer, G. , et al. , 1999. Entscheidungshilfe für die Mitverwendung von Abstellstreifen im Fließverkehr [Decision Guidance for use of emergency lance of highway traffic]. Vienna, Austria.
- Schulze, K.-H. , 1968. Typen der Oberflächenfeingestalt und ihre Wirkung auf den Reibungswiderstand bei Nässe [Types of surface microstructure and their impact on skid resistance at wet conditions]. Berlin: Internationales Colloquium über Straßengriffigkeit und Verkehrssicherheit bei Nässe.
- Seneviratne, H.N. , Rajapakshe, M.P.N. , and Gunaratne, M. , 2009. Field calibration of an analytical model for pavement friction testing applications. Journal of Testing and Evaluation, 37 (1), 21–30.
- Shabani, S. , Ahmadinejad, M. , and Ameri, M. , 2013. Developing a model for estimation of polished stone value (PSV) of road surface aggregates based on petrographic parameters. International Journal of Pavement Engineering, 14 (3), 242–255.10.1080/10298436.2012.693179
- Wang, D.W. , et al. , 2011. Evaluating on the skid resistance of the aggregates based on mineralogy. Advanced Building Materials, 250–253 (1–4), 3646–3651.
- Wang, D.W. , et al. , 2015. A study of the laboratory polishing behavior of granite as road surfacing aggregate. Construction and Building Materials, 89, 25–35.
- Wang, D.W. , et al. , 2015. Influence of the gritting material applied during the winter services on the asphalt surface performance. Cold Regions Science and Technology, 112, 39–44.10.1016/j.coldregions.2015.01.001
- Wehner, B. , 1968. Strassengriffigkeit und Verkehrssicherheit bei Nässe [Skid resistance and road safety at wet conditions]. Berlin: Internationales Colloquium über Straßengriffigkeit und Verkehrssicherheit bei Nässe.