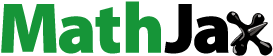
ABSTRACT
Oxidative aging is responsible for the irreversible asphalt stiffening and embrittlement leading to asphalt pavements of increased susceptibility to fatigue and thermal cracking. In recent years, various flexible binders have been introduced in asphalt industry to produce long-life pavements and the epoxy asphalt binders are among them. Nevertheless, in-depth understanding of the oxidative aging mechanism of epoxy asphalt binders and binding systems is still needed to enable reliable predictions of material degradation through service life. In this research, the compositional and rheological changes of epoxy asphalt, with and without filler, were analysed by means of Fourier Transform Infrared spectroscopy and Dynamic Shear Rheometer. Especially, kinetics (Arrhenius) parameters of epoxy asphalt have been determined by tracking the chemical composition changes. Oxidation of carbon species in epoxy asphalt is compositional dependent, and low values of activation energy accompany in low values of reaction rate are shown by adding epoxy in asphalt. Furthermore, the epoxy asphalt mastics (binders with filler) have been subjected to rheological testing to evaluate the stiffening effect as oxidation proceeds. Increase of modulus over a wide range of frequencies, decrease of frequency dependency of modulus of epoxy asphalt and shifting of phase angle to lower values are some important observations noticed as well. Overall, oxidation in epoxy asphalt materials occurs slowly yielding to oxygen-resistant binders when epoxy compounds are incorporated in asphalt binders.
Introduction
Nowadays, the whole asphalt industry has been focused on exploring new binders and modification technologies in an effort to offer a panoply to pavements against the climate change and the continuously increasing traffic volumes. Asphalt binder is a product of petroleum refining process comprising in majority of non-polar, high molecular weight hydrocarbons, and currently it is the most dominant binding material used in pavements. Nevertheless, most asphalt pavements exhibit shortcomings in terms of durability and together with the top priority of road administrations to minimise the regular maintenance and reconstruction operations, super-durable pavement materials are highly demanding.
In this context, long-life pavements have attracted the interest of road agencies and policy makers all over the world. Pavement structures of enhanced longevity would be expected to reduce the major repair needs justifying their high initial costs. New or relatively new binding systems specially designed to produce super-durable and long-lasting pavement materials have been proposed. One promising technology is the epoxy polymers which are able to lower the fracture sensitivity of asphalt materials (Widyatmoko et al. Citation2006, Youtcheff et al. Citation2006, Herrington and Alabaster Citation2008, Luo et al. Citation2015, Wu et al. Citation2017, Chen et al. Citation2020, Apostolidis et al. Citation2020a), and it has been utilised successfully as premium surfacing solution for bridge (Balala Citation1969, Gaul Citation1996, Huang et al. Citation2003, Lu and Bors Citation2015), airfield (Burns Citation1964, Joseph Citation1965) and roadway pavements (ITF Citation2017, Zegard et al. Citation2019, Dinnen et al. Citation2020).
In comparison with the thermoplastic block co-polymers, epoxy polymers are thermosetting materials which cannot be re-melted once they are fully cured. Particularly, the epoxy resins are inherently brittle amorphous or (semi)-crystalline polymers of long sequences of covalently linked monomers, the so-called macromolecules. The process to formulate a macromolecule from monomers is named curing. Thermoplastics, which are composed of linear or branched chains, can melt and dissolve in suitable solvents under certain energy conditions, thus they can be processed after curing. Opposite to thermoplastic polymers, during curing of a thermosetting, such as an epoxy binder, a covalently linked three-dimensional network is formed preventing melting (Bauer Citation1979, Pascault and Williams Citation2010).
In practice, the curing of thermosetting epoxy asphalt materials is influenced by several factors, such as the added polymer, the temperature, the mixing time in-plant and the time needed for mix transport and compaction on the road. Therefore, the road contractors could face on high manufacturing risks due to the uncontrolled temperature-driven irreversible curing of epoxy asphalt. The relatively slow curing of these binders could lead to mixtures that may segregate after compaction due to the low viscosity. Moreover, if the in-plant production and transport temperature of epoxy asphalt is too high, the material could be fully cured before paving which will make the material compaction impossible (ITF Citation2017). As a consequence, it is crucial to have full control over the whole production of epoxy asphalt (Apostolidis et al. Citation2018, Citation2019b, Citation2020b).
Apart from the high manufacturing risks of thermosetting epoxy asphalt materials, the wider utilisation of this technology has been prohibited due to the high cost of binders formulated exclusively by epoxy. To resolve this issue, asphalt binders have been diluted with epoxy. Within this framework, previous studies gave special emphasis on assessing the impact of epoxy on modified materials and the way it affects the overall mechanical performance at binder (Kang et al. Citation2010, Citation2015, Xiao et al. Citation2011, Gong et al. Citation2019, Apostolidis et al. Citation2019a) and mixture level (Luo et al. Citation2015, Wu et al. Citation2017, Luo et al. Citation2018, Apostolidis et al. Citation2020a). Since the use of epoxy binders is promoted mainly to produce materials of enhanced durability, the impact of epoxy on the oxidative aging performance of asphalt was evaluated as well (Herrington and Alabaster Citation2008, Apostolidis et al. Citation2019a, Citation2020c). However, fundamental understanding of oxidation mechanism of epoxy and epoxy diluted asphalt binders, collectively called epoxy asphalt binders, is still needed because of the significantly different nature of epoxy binders comparing the conventional asphalt binders.
Specifically, oxidation of asphalt binders is result of various concurrent chemical reactions proceeding over time. Stiffening and embrittlement of asphalt are the two primary effects of oxidation that determine the material degradation process, named oxidative aging (Petersen et al. Citation1993, Petersen, Harnsberger & Robertson Citation1996, Petersen & Harnsberger Citation1998, Petersen Citation2009). Quantitative information on the rate of chemical compositional changes of epoxy asphalt materials as function of time is important to predict the oxidative aging through their service life. Until now, predictions on the long-term performance of epoxy asphalt materials were with single-point data sets on time at 130°C (Apostolidis et al. Citation2019a, Citation2020c), much higher than the actual pavement temperatures. Useful lab data with pragmatic implications are missing to simulate the exact oxidation mechanism of such polymeric materials. Thus, kinetics parameters are important to assist on reliable predictions of the long-term performance of pavements as function of time and temperature.
The principle scope of this research was to assess the compositional changes of epoxy asphalt materials due to oxidative aging. The extent of the oxidation reaction in asphalt binders is tracked normally by the infrared peaks that represent carbonyls and sulfoxides. In this study, Fourier Transform Infrared (FTIR) spectroscopy was used to examine the compositional changes of epoxy asphalt binders with and without filler particles at different temperatures to determine ultimately the oxidation kinetics parameters. A simple theoretical model to describe the oxidation also used to estimate the kinetics parameters of oxidative aging epoxy asphalt. Finally, Dynamic Shear Rheometer (DSR) studies have been performed to assess the composition dependence on the rheological properties of epoxy asphalt materials due to oxidative aging.
Materials and methods
Materials and preparation
A 70–100 pengrade asphalt binder was selected for this research. The epoxy binder, supplied by ChemCo Systems (California, USA) is formulated from two liquid parts; (i) the Part A (epoxy resin formed from epichlorohydrin and bisphenol-A) and (ii) Part B (blend of petroleum asphalt, heavy naphthenic distillate and fatty acid extract). The basic properties of individual parts of epoxy binder are shown in . According to the supplier, Part A and B were oven-heated separately for 1 h, at 85°C and 110°C, respectively. Afterwards, Part A and B were mixed together for approximately 10–20 s and with a weight ratio of 20:80, and the epoxy binder was produced.
Table 1. Properties of epoxy binder (type BIX).
Asphalt binder is always in conjunction with mineral particles (i.e. filler and aggregates) of different sizes in asphalt pavements. Filler particles together with a binder form the mastic which is the binding system between aggregates. Fillers may act as catalyst or inhibitor through the asphalt oxidation process, a possibility which needs further investigation. Thus, mastic samples were prepared as well by mixing fillers with the newly formulated binders. In other words, to assess the influence of filler on oxidation kinetics of studied materials, the neat asphalt and epoxy asphalt binder were aged as well.
The filler particles used in this study were pure limestone particles (main minerals; dolomite and calcite) (density: 2.77 g/cm3; BET specific surface area: 13.25 m2/g) passed through the 0.075-mm sieve. The weight ratio of filler and binder was 56:44. Four mastic samples of different weight ratio of epoxy and asphalt binder were prepared (see ). Before mixing filler and binders, filler particles were pre-heated in the oven for approximately 60 min at 120°C. Mixing fillers and binders performed manually for 5 min to ensure production of homogeneous mixes without migrated particles at the bottom of can. All samples were placed in a refrigerator at −10°C to minimise further curing.
Table 2. Name and composition of studied materials.
The Superpave age conditioning protocols are not appropriate for evaluating the epoxy asphalt (EA) binders due to their thermosetting nature (Youtcheff et al. Citation2006). Therefore, all samples (3.2-mm sample thickness) were subjected to oven-conditioning (atmospheric pressure) over 0, 2, 5, 8, 24, 120, 240 and 480 h lengths of time at 80°C, 90°C and 100°C. Due to the fact that the oxidation rate of asphalt binder is affected by the material chemistry, film thickness and temperature, samples were obtained from the top surface of conditioned materials to avoid measuring samples of different aging levels at different depths. After each isothermal time period, the chemical composition changes were measured as function of time through FTIR spectroscopy. The epoxy compositional dependence of viscoelastic properties of mastics was evaluated as well by performing rheological measurements in a DSR.
FTIR spectroscopy
Typically, the asphalt oxidation, and subsequently stiffening, is distinguished in two phases, a non-linear fast-rate reaction and a linear constant-rate reaction phase (see ) (Van Oort Citation1956, Liu et al. Citation1996, Jin et al. Citation2011, Herrington Citation2012, Glaser et al. Citation2013, Liu and Glover Citation2015, Herrington et al. Citation2017, Jing Citation2019). Previous works focused on constant-rate kinetics of aging asphalt binder as well due to the fact that the duration of the fast rate period is considered to be relatively short of small impact on the long-term asphalt performance (Liu et al. Citation1996, Domke et al. Citation1999). The oxidation reactions as aliphatic sulphide to sulfoxide and benzylic carbon to carbonyl compounds have been indicated as the main reaction pathway of oxidative aging in asphalt that dominates the material stiffening (Petersen et al. Citation1993, Petersen and Harnsberger Citation1998).
In this study, the evolution of chemical compounds due to aging was tracked by FTIR spectroscopy equipped with an Attenuated Total Reflectance (ATR) fixture. IR spectra with wavenumber from 4000 to 600 cm−1 were recorded and collected for all the samples as in (Apostolidis et al. Citation2019a). A certain amount of material was placed directly on the ATR crystal pedestal and pressed with a constant force to ensure proper contact to the surface. A minimum of three sub-samples were investigated for each sample and 20 scans per sub-sample were performed with a fixed resolution of 4 cm−1. Typical spectra of functionalities of neat asphalt and EA binder recorded after oven-conditioning at 100°C for 480 h and shown in (a–c) demonstrate the spectra of filler and mastics, respectively.
Figure 2. IR spectra of (a) asphalt and EA binder, (b) filler and (c) asphalt binder with filler, or asphalt mastic, and EA mastic (after oven-conditioning at 100°C for 480 h).
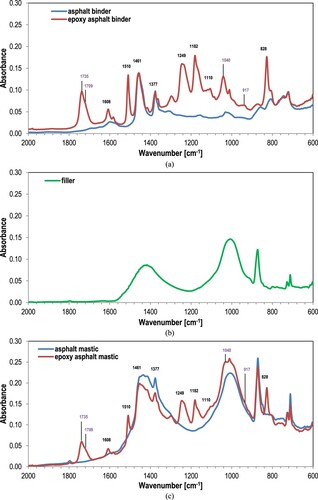
Once Part A is mixed with Part B, the curing reaction of epoxy occurs in presence of acid-based reaction catalysts/initiators giving ether and ester compounds, and producing the epoxy binder (Herrington and Alabaster Citation2008, Wei and Zhang Citation2012, Apostolidis et al. Citation2019a). Specifically, oxirane groups (at 917 cm−1) react with the carbonyl acid group (at 1709 cm−1) resulting into ester (at 1735cm−1) and ether (at 1040 cm−1). Hence, loss of carboxylic acid (at 1709 cm−1) and oxirane (at 917 cm−1) groups, and the corresponding increase of ester (at 1735 cm−1) and ether (at 1040 cm−1) groups happens. However, due to the fact that the curing of EA systems is beyond the scope of this research, the extent of oxidative aging of studied materials was evaluated by calculating the carbonyl (CO) and sulfoxide (SO) aging indices by using the area method. The CO and SO aging indices are defined as the integrated peak area from 1753 to 1660 cm−1 and from 1047 to 995 cm−1, respectively.
Dynamic shear rheometer
The mechanistic way of quantifying the oxidative aging deterioration in mastics (binder with filler) is by frequency sweep measurements using a DSR. In this research, the effect of aging on the performance of the frequency-dependent properties (i.e. complex modulus and phase angle) of oven-conditioned samples was determined in the DSR. DSR frequency sweep measurements (0.1–10 Hz, 0–50°C at temperature steps of 10°C) performed to quantify the oven-aging effect on mastic. The parallel plate testing geometry (plates of 8-mm diameter with a 2-m sample gap) was used and the aged samples (at 100°C) were reshaped to fit between the parallel plates. The master curves were produced with the time-temperature superposition model to shift all temperatures to 30°C.
Results and discussion
As mentioned earlier, one of the principle objectives of this study was to determine the oxidation kinetics parameters of epoxy asphalt and compare them with those of unmodified materials to quantify the overall aging sensitivity. Another objective was to assess the changes of modulus and phase angle of epoxy asphalt materials. Nevertheless, to restrain the scope of this research and to provide evidence about the improvement of oxidation resistance with the epoxy incorporation in asphalt, one base binder was used. Therefore, the current results are not generalised in all asphalt binders.
Compositional changes
The content of carbonyl and sulfoxide compounds was calculated with area method as a function of time at constant temperatures (isothermal oven-conditioning). The evolution of carbonyl and sulfoxide in asphalt and EA binder over time at different temperatures is demonstrated in . The total amount of carbonyl and sulfoxide compounds in these binders did not change dramatically at 80°C, however remarkable effects of temperature were noticed by increasing the applied temperature to 100°C. This trend is shown also when filler particles were added in binders, as shown in . These compounds increase as aging proceeds in all binders with and without filler.
Figure 3. Change of (a) sulfoxide and (b) carbonyl compounds of asphalt (top) and EA binder (bottom) over oven-conditioning at 80°C, 90°C and 100°C.
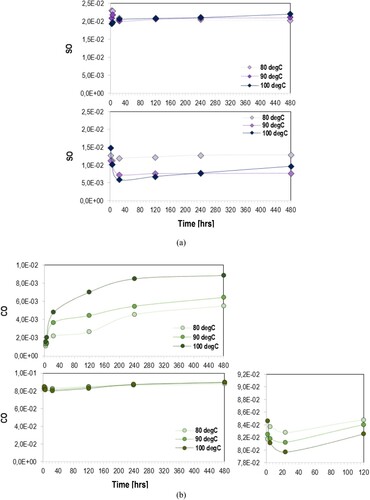
Figure 4. Change of (a) sulfoxide and (b) carbonyl compounds of asphalt (top) and EA mastic (bottom) over oven-conditioning at 80°C, 90°C and 100°C.
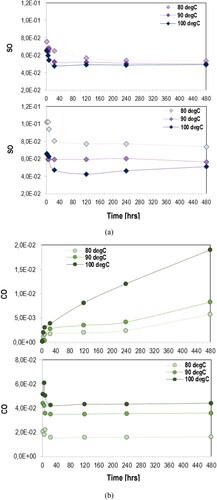
Both and depict a decreasing trend of carbonyl and sulfoxide compounds of EA materials at the first 24 h of oven-conditioning at constant temperature, due to the epoxy curing (Apostolidis et al. Citation2019a). At 80°C and 90°C, sulfoxides remain almost unchanged through the aging of asphalt binder and mastic as well. However, the sulphur reacting species of studied materials have shown an obvious increasing trend at 100°C (see (a) and 4(a)), a phenomenon which coincides with the increase attribute of sulfoxides in epoxy asphalt at 130°C, evaluated elsewhere (Apostolidis et al. Citation2019a, Citation2020c). Sulfoxide compounds continuously decrease at 80°C and 90°C and through the early conditioning time lengths at 100°C. Through the later conditioning time lengths, the rate of sulfoxide of asphalt and epoxy mastics shows an increasing tendency at 100°C.
Another significant observation is that the actual carbonyl compounds measured in the infrared spectrum has been significantly higher in epoxy binder (EA100) than of the neat asphalt binder (EA0), as demonstrated in (b). Similarly, high carbonyl values were measured in the epoxy binders with filler (see (b)). As mentioned, the epoxy resins are composed of monomers (i.e. building blocks) with an epoxide group at either end. Here, the bisphenol A diglycidyl ether (DGEBA) was used as epoxy monomer, which is included in Part A. The Part B includes free carboxylic acids able to react with the epoxide groups of epoxy monomers formulating new groups of ester and ether during esterification and etherification reactions. As indicated from the reduction of sulfoxide and carbonyl groups in and , the curing period was terminated after 24 h of oven-conditioning. According to earlier studies (Herrington and Alabaster Citation2008, Wei and Zhang Citation2012, Apostolidis et al. Citation2019a), the newly formed three-dimensional epoxy network is mainly composed of carbonyls. This was the primary reason why both EA100 and EAF100 demonstrated remarkably higher carbonyl compounds than of EA0 and EAF0. Nevertheless, carbonyls in asphalt increased more rapidly than in epoxy systems during aging.
For quantitative reasons, the incremental values of carbonyl compounds, demonstrated in and , were calculated to estimate the kinetics parameters of oxidation reaction of carbon species in studied materials. According to , the extent of carbonyl incremental values (ΔCOi = (COi–CO0)/CO0) of EA binder with (EAF100) and without filler (EA100) increased, in similar fashion with the carbonyl compounds (i.e. oxidised carbon species) in asphalt binder. To evaluate the universal oxidation mechanism of herein studied materials, the kinetic (Arrhenius) parameters can be determined to minimise the expensive long-term oxidation tests over different temperatures in the laboratory.
Figure 5. Incremental values of carbonyl compounds asphalt (top) and EA binder (bottom), (a) without and (b) with filler particles, over oven-conditioning at 80°C, 90°C and 100°C.
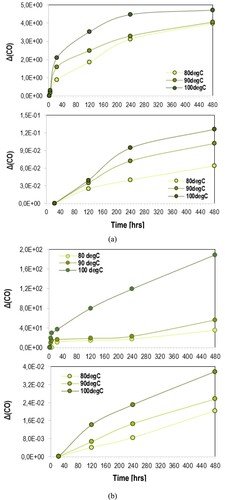
For the oxidation of studied binders with and without filler, incremental values of carbonyl compounds have been used and the methodology for estimating the oxidation kinetics parameters is discussed in the following section.
Kinetic parameters of carbonyl compounds formation
The chemical reaction process of carbonyls formation in asphalt binder is expressed as
(1)
(1) where x is the reacted carbon or carbonyl compounds at different aging times, k is the reaction rate coefficient and n is the reaction order number. In Equation (1), it has been assumed that chemical reaction of carbonyls formation was independent of oxygen concentration and the changes in the measured carbonyl peak area in the spectra were entirely due to oxidation. Thus, the first-order rate expression of reaction kinetics is as
(2)
(2) and by calculating its integral
(3)
(3) where t is the aging time and x0 is the initial carbonyl content.
Because the conditioning temperature affects the oxidation reactions and the transformation rate of carbon to carbonyl compounds, these effects can be incorporated into the reaction rate coefficients by using the Arrhenius approach as
(4)
(4) where Ea is the activation energy, R is the universal gas constant, T is the absolute temperature and A is the reaction factor.
Based on the data generated by the incremental values of carbonyls in both asphalt and epoxy materials, plots the relationship between –ln(k) and 1/T. lists the Ea and A values obtained from Equation (4). From Equations (4) and (3), the final form of the oxidation kinetic equation becomes as
(5)
(5) The values of kinetics (Arrhenius) parameters (i.e. activation energy and reaction rate) were fit by utilising the linearisation of the natural log of the rate constant plotted against 1/RT and the curve-fitting results are demonstrated in . Based on the relationships given above, Arrhenius parameters were calculated and provided in . In , the reaction rates of all materials illustrate a reasonable fit to the Arrhenius temperature dependency in isothermal conditions. The Arrhenius plot of asphalt and epoxy binder and of respective mastics are shown in (a,b), respectively.
Table 3. Kinetic parameters of studied materials.
The temperature sensitivity of materials is represented by the values of activation energy. As indicated in previous studies (Branthaver et al. Citation1993, Petersen et al. Citation1996, Petersen and Harnsberger Citation1998), the activation energy of carbonyls formation in asphalt binders is generally higher than of sulfoxides. So, the formation of carbonyl compounds is less temperature sensitive than of sulfoxides. Hence, the low values of activation energy of epoxy mastics correspond to more temperature sensitive systems comparing the unmodified materials. Increase of activation energy of asphalt and epoxy binders with filler was noticed in . Thus, decrease of the overall oxidation sensitivity is indicated with the addition of filler at any given temperature, a phenomenon observed elsewhere as well (Petersen et al. Citation1987, Moraes and Bahia Citation2015).
Data in show that the increase of epoxy proportion in asphalt binder, with and without filler, leads to the decrease of activation energy and reaction rate of carbonyls formation, and thus to temperature sensitive and oxygen-resistant systems, respectively. Based on this work performed only with running isothermal oven-aging tests at multiple temperatures, lower reaction orders and activation energies are exhibited on modified mastics of higher epoxy proportions in asphalt.
Rheological changes
The mechanical performance of epoxy asphalt binders is time- and temperature-dependent similar as asphalt binders. In this research, the epoxy binder (i.e. BIX type binder) were diluted in an asphalt binder formulating two-phase systems that stabilised, or toughened, with the addition of filler particles. Dynamic tests were conducted in DSR to assess the viscoelastic behaviour of aged epoxy-modified mastics, with filler particles, and at different epoxy modification levels, oven-aging time periods at 100°C.
The studied mastics were tested in frequency sweep at different temperatures (0°C, 10°C, 20°C, 30°C, 40°C and 50°C) and their complex modulus and phase angle master curves were compared using 30°C as the reference temperature. To assess the effect of aging on material viscoelasticity, it is common practice to use the complex modulus, as the total resistance of testing samples to deformation under different frequencies, and the phase angle, which indicates the viscous performance of samples. The construction of master curves enables the evaluation of rheological changes of studied mastics over time and also on estimating the material properties at conditions beyond the measuring equipment capabilities.
shows the master curves of complex modulus and phase angle of oven-conditioned samples (i.e. EAF0, EAF20, EAF50 and EAF100) after 480 h at 100°C. These materials are already fully cured. EAF20 and EAF50 have shown higher values of modulus over the analysing frequency range than the unmodified mastic, or EAF0, indicating the generation of stiffer materials by substituting asphalt binder with epoxy. The EA binder with filler, or EAF100, is definitely a material of high modulus, higher than of EAF0 and EAF20, and slightly lower than of EAF50, indicating that the latter have shown the stiffer material among all studied mastics (i.e. all studied modification levels).
Figure 7 Master curves of (a) complex modulus and (b) phase angle of studied materials after oven-conditioning for 480 h at 100°C.
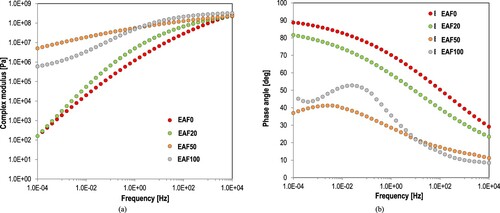
Another observation from the is that EAF100 mastic has shown a low frequency dependency of modulus over the studied frequency range indicating that this material is more elastic than of unmodified one. With the proportional substitution of asphalt binder with epoxy, epoxy mastics have been produced of similar frequency dependency as of EAF100, and particularly EAF50 has demonstrated this elastic behaviour. EAF20 is shown similar shape of complex modulus and also of phase angle as of EAF0, leading to the conclusion that the role of asphalt binder in mastic is more dominant in EAF20. The S-shape of phase angle of both EAF50 and EAF100 indicates that presence of a strongly crosslinked polymeric network and the rubbery characteristics of these materials at the whole frequency range.
demonstrates the master curves of complex modulus (part (i) of ) and phase angle (part (ii) of ) of EAF20, EAF50 and EAF100 after different time periods of oven-aging at 100°C. The influence of aging on the viscoelasticity of epoxy mastics is apparent in . The complex modulus increases rapidly with the oven-conditioning time. In case EAF50 and EAF100, the phase angle at low frequencies is dropped dramatically from 2 to 5 h of oven-conditioning at 100°C and generating the S-shape phase angle which indicates the strongly crosslinked polymeric network in asphalt. Over oven-aging, the phase angle over the whole frequency range tends to decrease and to shift along lower frequencies. The elastic crosslinked network which offers the rubbery characteristics to the system becomes less effective and this region disappears. The decrease of phase angle together with the increase of modulus, which becomes considerably frequency independent at all temperatures (frequencies), show the development of a rubbery system of high modulus and elasticity at a very broad temperature range. With increasing oven-age time periods, the phase angle decreases and thus epoxy mastics with 50% and 100% of EA binder in them becomes more elastic as shown in . EAF20 demonstrates a stiffening process similar of an unmodified material without indicating any epoxy polymeric network in it as EBF50 (see (b)). As noticed, the role of epoxy in EAF20 is not dominant and thus this material still ages and degrades with the typical way of asphalt materials.
Figure 8. Master curves of (i) complex modulus and (ii) phase angle of (a) EAF20, (b) EAF50, and (c) EAF100 during oven-conditioning for different times.
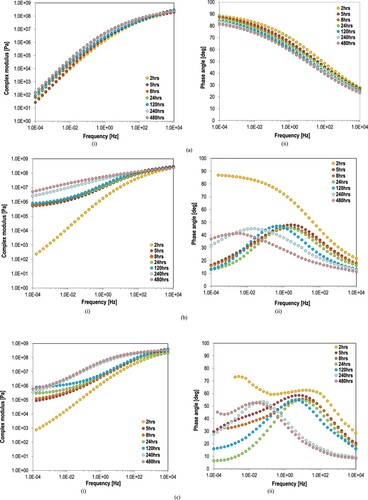
Finally, the compositional dependence of modulus and phase angle of oxidative aging epoxy asphalt mastics were studied in an effort to elucidate the relationship of epoxy on the oxidation extent and stiffening of asphalt. Modulus and phase angle values at 10 Hz of mastics (i.e. EAF0, EAF20, EAF50 and EAF100) after different oven-aging time periods at 100°C are provided in relationship to the epoxy proportion in asphalt in . From the data provided on modulus evolution on mastics, the modulus increase and phase angle decrease at 10 Hz appears sufficiently related with the amount of epoxy in asphalt (EAF0: 0 wt% and EAF100: 100 wt%). This phenomenon is more apparent at high epoxy proportions in asphalt. The modulus increase and phase angle decrease with the increase of oven-conditioning time lengths is more obvious in case of EAF50 and EAF100. This behaviour may lead to the conclusion that, apart from the aging degradation of their asphalt part, the epoxy asphalt mastics are still hardening after 480 h at 100°C due to the epoxy polymerisation.
Conclusions
To provide insight into the compositional changes due to oxidative aging of epoxy asphalt, the FTIR spectroscopy was used and the oxidation products were tracked at different temperatures over time. DSR studies have been performed as well to assess the composition dependence on the rheological properties of aging mastics. The key findings of this study are as follows;
When epoxy asphalt is oxidised isothermally, the carbonyl and sulfoxide compounds undergo a period of rapid decrease, which indicates the epoxy curing in asphalt binder. A time period of constant increase rate of these compounds was observed after curing, manifesting the oxidative aging. The incremental values of carbonyl compounds of epoxy asphalt materials were calculated through oxidative aging to estimate the oxidation kinetics parameters.
A simple oxidation model was utilised to describe the oxidation of epoxy asphalt materials (binders with and without filler), and to estimate the kinetics (Arrhenius) parameters. The oxidation of carbon species of epoxy asphalt materials was compositional dependent. Lower values of activation energy accompany in lower values of reaction rate were exhibited on mastics of high epoxy proportions in asphalt binder, with and without filler. The low values of activation energy of epoxy asphalt materials correspond to more temperature sensitive systems comparing the unmodified materials. Reduction of oxidation sensitivity (i.e. increase of activation energy and decrease of reaction rate of carbonyls formation) was demonstrated as well with the filler particles in both pure epoxy and asphalt binders. In the future, the kinetics parameters can be utilised to predict the material oxidative aging in pavements as a function of time and climatic conditions of interest.
The different mastics have been subjected to DSR testing as well to provide sufficient data to evaluate the stiffening effect due to oxidation. Increase of modulus over a wide range of frequencies, decrease of frequency dependency of modulus and shifting of phase angle to lower values were some important observations noticed over oven-aging of epoxy asphalt mastics. The S-shape of phase angle of both highly modified mastics indicates the presence of a strongly crosslinked polymer network and the rubbery characteristics of these materials at the whole frequency range. Finally, mastics with higher proportions of epoxy in asphalt have shown higher modulus and elasticity over aging times.
To summarise, the oxidative aging of epoxy asphalt mastics occurs slowly leading to the conclusion that the incorporation of epoxy compounds in asphalt binders could result in oxygen-resistant materials. Also, asphalt and epoxy asphalt binders are becoming of high modulus over aging time. Nevertheless, these materials are expected to be more brittle after aging, and thus more prone to fatigue- and thermal-induced cracking. Thus, the low temperature performance of oxidised epoxy binders and mastic will be studied in the future to assess their glass transition behaviour and ultimately their sensitivity to low temperature fracture.
Acknowledgements
The authors thank ChemCo Systems and Dura Vermeer for the cooperation.
Disclosure statement
No potential conflict of interest was reported by the author(s).
Additional information
Funding
References
- Apostolidis, P., et al., 2018. Chemo-rheological study of hardening of epoxy modified bituminous binders with the finite element method. Transportation Research Record: Journal of the Transportation Research Board, 2672, 190–199. TRB, National Research Council, Washington, DC. doi: https://doi.org/10.1177/0361198118781377
- Apostolidis, P., et al., 2019a. Evaluation of epoxy modification in bitumen. Construction and Building Materials, 208, 361–368. doi: https://doi.org/10.1016/j.conbuildmat.2019.03.013
- Apostolidis, P., et al., 2019b. Kinetic viscoelasticity of crosslinking epoxy asphalt. Transportation Research Record: Journal of the Transportation Research Board, 2673, 551–560. TRB, National Research Council, Washington, DC. doi: https://doi.org/10.1177/0361198119835530
- Apostolidis, P., et al., 2020a. Use of epoxy asphalt as surfacing and tack coat material for roadway pavements. Construction and Building Materials, 250, 118936. doi: https://doi.org/10.1016/j.conbuildmat.2020.118936
- Apostolidis, P., et al., 2020b. Characterization of epoxy-asphalt binders by differential scanning calorimetry. Construction and Building Materials, 249, 118800. doi: https://doi.org/10.1016/j.conbuildmat.2020.118800
- Apostolidis, P., et al., 2020c. Evaluation of epoxy modification in asphalt mastic. Materials & Structures. https://doi.org/https://doi.org/10.1617/s11527-020-01494-9.
- Balala, B, 1969. Studies leading to choose of epoxy asphalt for pavement on steel orthotropic bridge deck of San Mateo-Hayward bridge. Highway Research Record, 287, 12–18.
- Bauer, R.S., 1979. Epoxy resin chemistry. Washington, DC: American Chemical Society.
- Branthaver, J.F., et al., 1993. Binder characterization and evaluation, volume 2: chemistry. Washington, DC: SHRP, National Research Council, Report No. SHRP-A-368.
- Burns, C.D., 1964. Laboratory and field study of epoxy-asphalt concrete. US Army Engineer Waterways Experiment Station, US Corps of Engineers, Technical Report 3-368.
- Chen, C., et al., 2020. Performance characteristics of epoxy asphalt paving material for thin orthotropic steel plate decks. International Journal of Pavement Engineering, 21 (3), 397–407. doi: https://doi.org/10.1080/10298436.2018.1481961
- Dinnen, J., Farrington, J., and Widyatmoko, I., 2020. Experience with the use of epoxy-modified bituminous binders in surface courses in England. Asphalt Professional, 82, 14–21.
- Domke, C.H., et al., 1999. Effect of oxidation pressure on asphalt hardening susceptibility. Transportation Research Record: Journal of the Transportation Research Board, 1661, 114–121. TRB, National Research Council, Washington, DC. doi: https://doi.org/10.3141/1661-16
- Gaul, R.W, 1996. Epoxy asphalt concrete – a polymer concrete with 25 years’ experience. American Concrete Institute Publication Symposium, 166 (13), 233–251.
- Glaser, R.R., et al., 2013. Low-temperature oxidation kinetics of asphalt binders. Transportation Research Record: Journal of the Transportation Research Board, 2370, 63–68. TRB, National Research Council, Washington, DC. doi: https://doi.org/10.3141/2370-08
- Gong, J., et al., 2019. Performance of epoxy asphalt binder containing warm-mix asphalt additive. International Journal of Pavement Engineering. https://doi.org/https://doi.org/10.1080/10298436.2019.159727.
- Herrington, P.R, 2012. Diffusion and reaction of oxygen in bitumen films. Fuel, 94, 86–92. doi: https://doi.org/10.1016/j.fuel.2011.12.021
- Herrington, P. and Alabaster, D., 2008. Epoxy modified open-graded porous asphalt. Road Materials and Pavement Design, 9 (3), 481–498. doi: https://doi.org/10.1080/14680629.2008.9690129
- Herrington, P., James, B., and Henning, T.F.P., 2017. Validation of a bitumen oxidation rate model. Transportation Research Record: Journal of the Transportation Research Board, 2632, 110–118. TRB, National Research Council, Washington, DC. doi: https://doi.org/10.3141/2632-12
- Huang, W., et al., 2003. Epoxy asphalt concrete paving on the deck of long-span steel bridges. Chinese Science Bulletin, 48 (21), 2391–2394. doi: https://doi.org/10.1360/02ww0123
- International Transport Forum, 2017. Long-life surfacings for roads: field test results. Paris, France: OECD.
- Jin, X., et al., 2011. Fast-rate–constant-rate oxidation kinetics model for asphalt binders. Industrial & Engineering Chemistry Research, 50, 13373–13379. doi: https://doi.org/10.1021/ie201275q
- Jing, R, 2019. Ageing of bituminous materials: experimental and numerical characterization. Thesis (PhD). TU Delft.
- Joseph, A.H., 1965. Behavior of epoxy-asphalt airfield pavement, 1963 inspections. US Army Engineer Waterways Experiment Station, US Corps of Engineers, Technical Report 4-704.
- Kang, Y., et al., 2010. Rubber-like thermosetting epoxy asphalt composites exhibiting atypical yielding behaviors. Journal of Applied Polymer Science, 116, 1678–1685.
- Kang, Y., et al., 2015. Rheological behaviors of epoxy asphalt binder in comparison of base asphalt binder and SBS modified asphalt binder. Construction and Building Materials, 76, 343–350. doi: https://doi.org/10.1016/j.conbuildmat.2014.12.020
- Liu, M., et al., 1996. The kinetics of carbonyl formation in asphalt. AIChE Journal, 42, 1069–1076. doi: https://doi.org/10.1002/aic.690420417
- Liu, G. and Glover, C.J., 2015. A study on the oxidation kinetics of warm mix asphalt. Chemical Engineering Journal, 280, 115–120. doi: https://doi.org/10.1016/j.cej.2015.05.074
- Lu, Q. and Bors, J., 2015. Alternate uses of epoxy asphalt on bridge decks and roadways. Construction and Building Materials, 78, 18–25. doi: https://doi.org/10.1016/j.conbuildmat.2014.12.125
- Luo, S., et al., 2015. Performance evaluation of epoxy modified open-graded porous asphalt concrete. Construction and Building Materials, 76, 97–102. doi: https://doi.org/10.1016/j.conbuildmat.2014.11.057
- Luo, S., et al., 2018. Laboratory evaluation of double-layered pavement structures for long-span steel bridge decks. Journal of Materials in Civil Engineering, 30 (6), 04018111. doi: https://doi.org/10.1061/(ASCE)MT.1943-5533.0002291
- Moraes, R. and Bahia, H.U., 2015. Effect of mineral fillers on the oxidative aging of asphalt binders. Transportation Research Record: Journal of the Transportation Research Board, 2506, 19–31. TRB, National Research Council, Washington, DC. doi: https://doi.org/10.3141/2506-03
- Pascault, J.-P. and Williams, R.J.J., 2010. Epoxy polymers: new materials and innovations. Weinheim: Wiley-VCH Verlag GmbH & Co. KGaA.
- Petersen, J.C., et al., 1993. Effects of physicochemical factors on asphalt oxidation kinetics. Transportation Research Record: Journal of the Transportation Research Board, 1391, 1–10. TRB, National Research Council, Washington, DC.
- Petersen, J.C., 2009. A review of the fundamentals of asphalt oxidation: chemical, physicochemical property, and durability relationship. Transportation Research Circular, Number E-C140, Transportation Research Board of National Academies, Washington, DC.
- Petersen, J.C. and Harnsberger, P.M., 1998. Asphalt aging: dual oxidation mechanism and its interrelationships with asphalt composition and oxidative age hardening. Transportation Research Record: Journal of the Transportation Research Board, 1638, 47–55. TRB, National Research Council, Washington, DC. doi: https://doi.org/10.3141/1638-06
- Petersen, J.C., Harnsberger, P.M., and Robertson, R.E., 1996. Factors affecting the kinetics and mechanisms of asphalt oxidation and the relative effects of oxidation products on age hardening. Preprints, Division of Fuel Chemistry. American Chemical Society, 41 (4), 1232–1244.
- Petersen, J.C., Plancher, H., and Harnsberger, P.M., 1987. Lime treatment of asphalt to reduce age hardening and improve flow properties. Journal of the Association of Asphalt Paving Technologists, 56, 632–653.
- Van Oort, W.P, 1956. Durability of asphalt – it's aging in the dark. Industrial and Engineering Chemistry, 48, 1196–1201. doi: https://doi.org/10.1021/ie50559a033
- Wei, J. and Zhang, Y., 2012. Study on the curing process of epoxy asphalt. Journal of Testing and Evaluation, 40 (7), 1–8. doi: https://doi.org/10.1520/JTE20120136
- Widyatmoko, I., et al., 2006. Curing characteristics and the performance of epoxy asphalts. Tenth International Conference on Asphalt Pavements.
- Wu, J.P., et al., 2017. Long-term durability of epoxy-modified open-graded porous asphalt wearing course. International Journal of Pavement Engineering, 20 (8), 920–927. doi: https://doi.org/10.1080/10298436.2017.1366764
- Xiao, Y., et al., 2011. Characteristics of two-component epoxy modified Bitumen. Materials and Structures, 44, 611–622. doi: https://doi.org/10.1617/s11527-010-9652-2
- Youtcheff, J., et al., 2006. The evaluation of epoxy asphalt and epoxy asphalt mixtures. Proceedings of the Canadian Technical Asphalt Association, 51, 351–368.
- Zegard, A., et al., 2019. Long-lasting surfacing pavements using epoxy asphalt: province of North Holland case study. Transportation Research Board, 98th Annual Meeting, Washington DC.