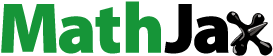
ABSTRACT
Conventional hot mix plants operate to support large paving projects, making production more economic with high volume output. When repairs and maintenance are needed, it can be challenging to maintain small quantities of hot bituminous mixtures at a sufficient temperature, especially in the case of winter maintenance. Consequently, the repair materials cannot be compacted to the desired level on some occasions. This research aimed to develop a polymer modified asphalt binder with reduced temperature sensitivity for hand-laid and low-volume applications. The results showed that the highest penetration index has been achieved by modifying bitumen with 20% rubber and 2% wax. The FTIR and XRD analysis indicated that the bitumen, rubber and wax that react chemically to build 3D networks have an interlocked structure in the bitumen matrix resulting in reduced temperature sensitivity of the polymer modified asphalt binder. Furthermore, indirect tensile stiffness, permanent deformation, creep test and fatigue life test, water sensitivity and freeze-thaw cycle demonstrated an improvement in the asphalt mixture properties in terms of mechanical and durability perspectives. Overall, based on this investigation, modifying asphalt binder with 20% rubber and 2% wax resulted in stronger and durable asphalt mixture in comparison to traditional hot mix asphalt.
1. Introduction
Producing high-quality asphalt materials for low-volume hand-laid applications for emergency pothole patching and utility cut repairs has always presented a challenge. The widely used material in pavement repair is hot-mix asphalt (HMA). The main drawback of HMA is that it is produced in batches of two to three tons, despite the average patch requiring only a small amount of asphalt (Saeed and Hammons Citation2009). When keeping the HMA at a high temperature for a long duration during the process of mixing, transportation and storage, the bitumen ages, and its viscosity and softening point are increased, leading to fretting and/or cracking (Read et al. Citation2003). In addition, the temperature of HMA drops during transportation, especially for remote maintenance during winter (Carruth and Santiago Citation2015). This reduction in temperature not only reduces the compressibility of the mixture (resulting in high air void content) but also weakens the joint between the old surface and the new patching materials that trigger moisture ingress.
In recent decades, some polymeric materials have been developed to improve asphalt patching repair materials (Yuan et al. Citation2012), but it was relatively expensive to tailor them for specific special applications. Modifying bitumen with additive can improve bitumen characteristics such as the adhesion to aggregate and workability and thus improving the properties of the final asphalt mixture (Suganpriya et al. Citation2016). Additives are used to delay the deterioration and increase the service life of the pavements.
The temperature sensitivity of asphalt binder plays a significant role in understanding asphalt pavement failures, especially on the asphalt aggregate adhesion (Wu et al. Citation2008), as well as it is related to asphalt mixtures ability to resist permanent deformation (Wang et al. Citation2009). The temperature sensitivity indicates how quickly asphalt properties change over time in terms of indices such as penetration index. All asphalt binders share the similar basic thermoplastic property of being softer when heated and stiffer when cooled (Kishchynskyi et al. Citation2016). Normal asphalt binder has PI ranging between (−2 and +2). Asphalt binder has low sensitivity to temperature when PI values increased more than +2, while the asphalt binder is extremely sensitive to high temperature when PI values less than −2 (Abedali Abed and Al-Haddad Citation2019).
Using polymer in modifying asphalt has been considered as a great option to produce mixtures that can improve both rutting and cracking; despite this, from an economic perspective, it is relatively expensive. However, the use of recycled polymer such as crumb rubber offers an inexpensive alternative (Mashaan et al. Citation2014). Rubberised binders have improved asphalt pavement’s quality, increased rutting and cracking resistance and therefore reduced maintenance costs (Porto et al. Citation2019). Critical evaluation of several previous research showed that low content of rubber (around 4%) has almost no significant effect on the mechanical properties and the performance of the asphalt mixtures, more than 20% was also found to be inappropriate (Porto et al. Citation2019). Moreover, in terms of visco-flow characteristics and viscosity, it is suggested to use 15–25% of crumb rubber at 180°C of treatment temperature and a treatment duration of 90 min (Li et al. Citation2018). However, rubberised binders may be attributed to the weak compatibility between rubber and bitumen, which is demonstrated by its propensity to separate during transportation to the paving site or during high-temperature static storage (Polacco et al. Citation2015, Behnood Citation2019). Hence, transportation and temperature can be considered very important factors because they impact the performance of the pavement. Therefore, adding wax to rubberised binders may tackle this problem to improve the temperature sensitivity of the binder (Hainin et al. Citation2015, Wang et al. Citation2016).
Wax (Sasobit®) is an additive to bitumen that improves its workability and durability. Sasobit® additive could decrease the mixing and compaction temperatures of rubberised asphalt mixtures and extend the long-term performance of the pavement (Xiao et al. Citation2009, Jamshidi et al. Citation2013). At temperatures under 100°C, Sasobit® seemingly emulates the structure of crystalline lattice in the binder resulting in improved rutting resistance at service temperatures leading to improved stability (Wasiuddin et al. Citation2012). Furthermore, Sasobit® plays as a flow-improver decreasing the binder viscosity and thus enabling lower mixing and compaction temperatures (Hainin et al. Citation2015). Recent studies have demonstrated that adding 1–3% of Sasobit® to the asphalt binder lowers the binder viscosity making it much simpler to be mixed and handled at lower temperatures compared to unmodified asphalt binder (Ghuzlan and Al Assi Citation2017). They also recommended that the optimum dose of Sasobit® to modify the asphalt binder is 2%, while a higher percentage has negatively affected the performance of asphalt mixtures (Ghuzlan and Al Assi Citation2017).
It can be concluded that rubber and wax have a strong influence on the properties of the binder, as well as the mixture. These materials are expected to improve the temperature sensitivity of asphalt binder as well as the workability and compactability of asphalt mixtures to be used in pavement repair. This research aimed to develop a polymer-modified asphalt binder exhibiting reduced temperature sensitivity for hand-laid pavement repair in low-volume applications.
2. Materials and experimental methods
Based on the previous studies, the neat bitumen samples were modified with rubber and wax by adding the desired dosages of additives to the binder (neat bitumen). shows the initial physical properties of the candidate binder and additives. The grade of the bitumen used in this investigation was 100/150 according to BS EN 12591 (European Committee for Standardization Citation2009).
Table 1. Initial physical properties of candidate binder and additives.
In order to investigate the individual influence of rubber and wax on binder (neat bitumen), mixes containing different workable ranges of these modifiers in the binary blend were tested, as shown in . The expectations from these mixes were that they would improve the workability of the patching materials by reducing the temperature sensitivity, enhancing PI.
Table 2. Experimental scheme to examine the influence of additives on asphalt binder.
illustrates the flowchart to show the methods, materials and tests performed to assess the physical properties of the polymer-modified asphalt binder (PMAB) (developed binder), also the mechanical and durability properties of polymer-modified asphalt mixture using the developed binder. First, the bitumen was heated in an oven at 180°C for 1 h. Then, the modifiers (rubber and wax) were blended with bitumen at 180°C and 2000 rpm, for 90 min. The second laboratory programme covered the determination of stiffness modulus, permanent deformation using wheel track test (WTT) and fatigue performance using four-point bending test (4PB). The final stage of the laboratory programme covered the durability tests for asphalt mixtures, such as water sensitivity and freeze and thaw test.
Figure 1. Flowchart showing the methods, materials and tests performed to evaluate the physical, mechanical and durability properties of the developed binder.
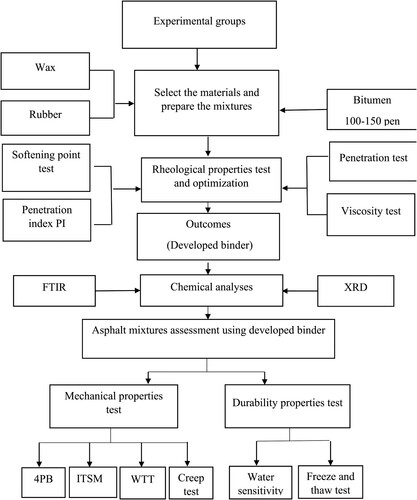
2.1. Assessment of modified binder
The penetration test was conducted according to BS EN 1426 (European Committee for Standardization Citation2015a). The penetration test is a widely used method to assess the consistency of a bituminous binder at a specific temperature. The standard test for softening point of bituminous materials is the Ring and Ball method that has been conducted according to BS EN 1427 (European Committee for Standardization Citation2015b). The softening point indicates the temperature at which bitumen starts to display fluidity (Nassar and Ibrahim Citation2012). As it is known, the softening point has a direct relationship with asphalt deformation; the higher softening point, the more deformation resistance (Al-Hadidy and Yi-qiu Citation2009). The Penetration index (PI) is the measure of temperature sensitivity of binder and stiffness of asphalt. Low-temperature sensitivity is a reflection of increased resistance against thermal cracking and permanent deformation (Ghasemi and Marandi Citation2013, Sitinamaluwa and Mampearachchi Citation2014). Thus, asphalt mixture containing binder with high PI has greater resistance to low-temperature cracking and permanent deformation (Ghasemi and Marandi Citation2013, Taherkhani and Afroozi Citation2016, Yaacob et al. Citation2016). The calculation of the PI is based on values of penetration and softening point according to BS EN 12591 (European Committee for Standardization Citation2015c). PI was calculated using the equation provided below:
where SP is the softening temperature in degrees Celsius and Log P is the common logarithm of penetration at 25°C.
Viscosity can be defined as the resistance to the flow of a fluid. Considering the standard test temperature used by Li et al., the test was performed at a mixing temperature of 180°C for PMAB (Li et al. Citation2018). A Brookfield DV-II+ Pro Viscometer rotational viscometer was used according to BS EN 13302 (European Committee for Standardization Citation2018a) at 180°C.
The physical and chemical properties of asphalt binder can be described using Fourier transform infrared spectrometer (FTIR) (Weigel and Stephan Citation2017). FTIR spectroscopy is the most frequently used tool to observe the changes in the chemical structure of bitumen after ageing and measure the oxidation products. FTIR gives precise information regarding oxygenation rate, aliphaticity and aromaticity (Lushinga et al. Citation2019). The FTIR spectroscopy was conducted using the instrument: Perkin-Elmer Spectrum BX series FTIR, equipped with a Miracle ATR accessory. In this investigation, the FTIR was employed on the neat bitumen and PMAB. X-ray Diffraction is a non-destructive and multipurpose analysis technique used to distinguish crystalline phases in the materials and to investigate the structural properties of these phases (Al-Hdabi Citation2014). The XRD test was conducted using the instrument Rigaku mini-flex diffractometer (mini-flex goniometer), CuK X-ray radiation (30 kV voltage and 15 mA current at scanning speed of 2.0°/min in continuous scan mode) and scanning range (2θ) of 5–60°. XRD is a commonly used tool to determine the crystallite parameters of modified binder; it offers quantitative intensity curves according to the peak intensity and position of the structural parameters in the binder sample (Alhumaidan et al. Citation2015).
2.2. Aggregates used for specimen
The coarse and fine aggregate used in this investigation to manufacture asphalt mixtures was of crushed granite from Carnsew Quarry at Mabe, Penryn, UK. A sieve analysis according to the standard BS EN 933-1 (European Committee for Standardization Citation2012) was performed on the aggregate. The aggregate structure permitted a curve to be established following EN 13108-1 (European Committee for Standardization Citation2016a). and show the particle size distribution of the aggregate where a dense aggregate gradation for asphalt concrete binder course AC10 was used to prepare asphalt mixture (RuW) using PMAB (developed binder). Additionally, the results have been compared with control traditional hot mix asphalt (HMA) of AC10 using a conventional 100/150 penetration grade neat binder. The binder content of 5.2% by weight of aggregate was used.
Table 3. Aggregate gradation for AC10 surface course.
2.3. Assessment of asphalt mixture
2.3.1. Indirect tensile stiffness modulus test (ITSM)
Stiffness Modulus is the uniaxial stress divided by the corresponding strain. It has been referred to as an indicator of satisfactory structural design due to its relation to the capacity of individual pavement layers enabling the traffic loads to spread to the layer underneath. ITSM was performed in accordance with BS EN 12697-26 (European Committee for Standardization Citation2018b) using Cooper Research Technology HYD-25 testing apparatus, seen in .
In this test, the samples were exposed to five transient load pulses along its vertical axis; the subsequent indirect deformation crosses the horizontal axis obtained using Linear Variable Differential Transducers (LVDTs).
2.3.2. Wheel track test (permanent deformation)
Wheel tracking tests are used to evaluate the permanent deformation of the asphalt mixtures at 45°C and 60°C. This has been set as according to the European Committee for Standardisation for hot weather (European Committee for Standardization Citation2007). Also, these two temperatures have been selected following the British Standard PD 6691:2016 (European Committee for Standardization Citation2016b), 45°C shows moderate to heavily stressed sites demanding high rutting resistance, whereas 60°C shows very heavily stressed sites demanding very high rutting resistance. The loose asphalt mixtures were blended and compressed in a steel mould under a roller compactor, making a solid block with measurements of 405 mm (length) × 300 mm (width) × 50 mm (thickness). These blocks were left to sit in the mould for a 24-hour period at room temperature before conducting the test, as shown in .
2.3.3. Four-point bending (fatigue test)
Several factors can result in fatigue cracking of asphalt pavements, including traffic loading and freeze and thaw cycle, which causes high stress on the asphalt pavement (Read et al. Citation2003, Dulaimi et al. Citation2020). A repeated loading pressure fatigue creates cracking in two phases: the crack initiation phase through micro cracks and the crack propagation phase through which the micro cracks increase under other applications of tensile strains (Read et al. Citation2003). In this research, a four-point bending test (4PB) () was used to evaluate the resistance of fatigue crack for the asphalt mixtures containing modified binder in accordance with BS EN 12697-24 (European Committee for Standardization Citation2018b).
This device is capable of clamping specimens in a bending frame to provide horizontal translation and rotational freedom at all supports. As cyclic bending is started, the two inner clamps are loaded in the vertical direction, and the perpendicular position of the outer clamps is fixed. A constant movement was generated with a constant strain between the inner loading points. The fatigue life can be defined as the number of required cycles to achieve a decrease of a 50% in the original stiffness of the mixture. According to (Dulaimi et al. Citation2020), the strain level (150 microstrains) in a pavement structure depends on variables including the type of mixture, the road load, the layer thickness and subgrade. Tests were executed on prismatic samples measuring 400 × 50 × 50 mm at a frequency of 10 Hz and a temperature of 20°C under a sinusoidal waveform in a pre-set strain mode.
2.3.4. Creep test (creep and relaxation test)
The creep test illustrates a way for detecting the creep parameters of asphalt mixtures through uniaxial static creep and relaxation. The sample is exposed to a static pressure showing the strain in 3600-s loading followed by 3600-s unloading conducted at a stress level of 100 kPa according to the European Committee for Standardization BS EN 12697-25 (European Committee for Standardization Citation2018b). The accumulative strain (permanent deformation) of the test sample is obtained as a function of the loading time. The samples were kept at the test temperature of 20°C and 40°C (±1°C) from four to seven hours. The creep and relaxation curves were drawn based on the average value of the three tested samples. The creep sample was cylindrical with 150 mm in diameter, which was put between two plane-parallel loading plates with the upper plate has a diameter of 100 mm ().
2.4. Asphalt mixtures durability assessment
2.4.1. Water sensitivity test
In this study, a water sensitivity test was carried out according to BS EN 12697-12 (European Committee for Standardization Citation2017). This test reveals any loss of the adhesive bond between the bitumen and aggregate in the cylindrical samples in the presence of water. In this test, the compressed samples were split into two groups. The first group was for dry testing, and the second was for saturated. The dry samples were tested without moisture conditioning as they were left in the mould (after compaction) for a day at room temperature. The samples in the second group were saturated as part of the moisture pre-condition protocol. For this, every sample was extruded and dipped in a water bath at room temperature for four days; after that, the samples were removed from the water bath and placed in the vacuum container (). To reach the needed degree of saturation, vacuum pressure and duration (6.7 kPa for 10 min) was applied to the samples. Following the completion of the vacuum process, the specimens were left in the vacuum container for another 30 min and then transferred from the container to a flat surface in a water bath at 40°C for three days, before being tested.
Figure 7. Experimental arrangement for water sensitivity test showing the vacuum container and the samples in the water bath at 40°C.
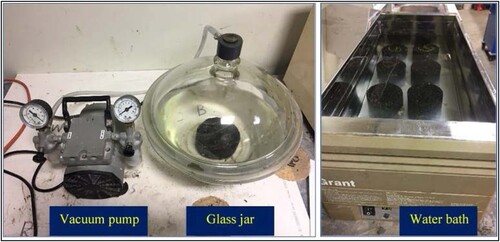
The dry and wet specimens were tested at 20°C according to BS EN 12697-12 (European Committee for Standardization Citation2018b). For each mixture type, five sets of each sample were tested. Water sensitivity of asphalt mixtures is measured using the indirect tensile stiffness modulus ratio (SMR) or indirect tensile strength ratio (ITSR) (Dulaimi et al. Citation2017, Shanbara et al. Citation2018). In this research, water sensitivity was obtained using SMR as seen in Equation (1) (Al-Hdabi et al. Citation2014, Dulaimi et al. Citation2017)
2. 4.2. Freeze and thaw test
Asphalt pavements deteriorate over time because of the environment and daily heavy traffic loading on roads. A harsh environment such as cold temperatures can also cause pavements to crack, but pavement deterioration is most notably caused by freeze and thaw cycles (Biswas et al. Citation2018, Cong et al. Citation2020). To apply freeze and thaw cycles, there is no specific approach recommended (Feng et al. Citation2010, Si et al. Citation2015, Fakhri et al. Citation2020); however, some procedures have been suggested in the literature for freeze and thaw cycles on asphalt mixtures. As can be seen, a range of temperatures was applied in the freeze and thaw cycles assessment. Therefore, based on recent studies (Fiber et al. Citation2018, Cong et al. Citation2020), the freeze and thaw process was carried out by placing the samples into an automatic chamber () with freeze and thaw cycles (maximum of 10 times).
The automated freeze and thaw procedure is illustrated in . Samples were vacuum saturated at 97.3 kPa for 15 min and placed into the automatic chamber for the main procedure (Fiber et al. Citation2018, Cong et al. Citation2020). After cycling, the specimens were cooled in a water container at a temperature of 25°C for 2 h before the ITSM test to calculate the SMR (Cong et al. Citation2020).
Table 4. Freeze and thaw temperature regime followed.
3. Results and discussion
3.1. Assessment of the binder
3.1.1. Influence of rubber on binder
The influence of rubber content on binder PI and dynamic viscosity is illustrated in and , respectively. As rubber content increases in the mix, the penetration point decreases and softening point increases. ((A,B)). This observation was in accordance with Mashaan et al. (Citation2011) studies. The PI was considerably increased by the addition of rubber, up to positive values of about 1.50 when adding 20%, as shown in (C), which consequently improved the temperature sensitivity of the rubberised binder. Indeed, adding rubber enhanced the performance properties of asphalt pavement in terms of resistance against deformation during construction and road services (Mashaan et al. Citation2014). Moreover, a binder with a higher PI has a higher resistance to low-temperature cracking and permanent deformation, as reported by many studies (Ghasemi and Marandi Citation2013, Taherkhani and Afroozi Citation2016, Yaacob et al. Citation2016). To maximise the PI of rubberised binder, the optimum dose was found to be 20% in this research, which was also in accordance with the findings of Porto et al. (Citation2019).
Figure 9. Influence of rubber on bitumen (A) penetration test (B) softening point test C) penetration index PI.
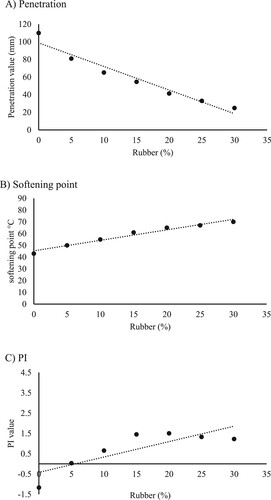
The positive correlation between rubber content and viscosity is evident in . Significantly, sharp increases in viscosity were recorded above 15% rubber content. This could happen because the rubber absorbs the light component of the bitumen, resulting in variation of the binder component that increases binder viscosity in the PMAB system. The increase in viscosity leads to an increase in binder film thickness around the aggregate due to the gel structure assumed by the PMB, improving the adhesion and cohesion of the asphalt mixture (Presti Citation2013).
Gel penetration chromatography analysis shows that a high percentage of crumb rubber (15–20%) in bitumen contributes to reducing the component of the binder’s large molecular sizes value (Lee et al. Citation2011). This is due to the ejection of lighter constituents that were at lower proportions, being absorbed by the rubber from the bitumen (Lee et al. Citation2011). Furthermore, significant improvements in terms of ageing and creep stiffness, as well as lower energy requirement, when the dosage of rubber was in the range of 15–20% (Wang et al. Citation2012). Overall, the higher the amount of crumb rubber, the higher the viscosity of PMB thus improving the properties of the binder at high temperatures. Nevertheless, an excessive amount of crumb rubber may result in the weakness of the asphalt mixture with inferior end properties at low winter temperatures. Considering all the factors above and the results for PI and viscosity, the optimum rubber amount was observed to be 20% in modified bitumen, a level that is expected to improve the workability and durability of the proposed asphalt mixture.
3.1.2. Influence of wax on binder
The influence of wax concentration on binder PI and dynamic viscosity is illustrated in and , respectively. Varying the wax concentration was shown to influence the penetration ((A)) and softening point ((B)) of the binder and the PI in a similar way to the rubber effect. The explanation for this might be that when the wax cools and crystallises it forms a uniform network structure in the binder (Hainin et al. Citation2015). Furthermore, at temperatures below 100°C, wax reportedly forms a crystalline lattice structure in the binder that is the basis for the increased resistance to rutting at service temperatures leading to more stability (Hurley and Prowell Citation2005, Wasiuddin et al. Citation2012). (C) illustrates that optimum PI was identified when the wax concentration was around 2%. Previous researchers have recommended the addition rate of wax to be in the range of 1–3% by mass of the binder (Zhao and Guo Citation2012). Others have reported that using more than 4% wax has a harmful impact, causing cracking of asphalt mix at low temperatures (Hainin et al. Citation2015). Additionally, Ghuzlan and Al Assi (Citation2017) pointed out that the optimum percentage of wax to be added to the asphalt binder is 2%, and higher percentages negatively affect the performance of asphalt. Thus, with the addition of 2% wax, the modified binder is expected to be less susceptible to temperature changes. Hainin et al. (Citation2015) found that the network of the crystalline structure formed in the wax-modified binder reduces temperature sensitivity and increases the elasticity of the binder.
Figure 11. Influence of wax on bitumen (A) penetration test (B) softening point test (C) penetration index PI.
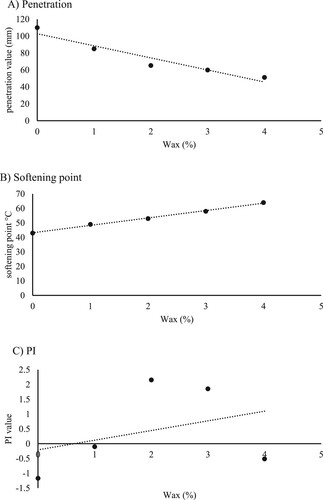
As can be seen in , increasing the wax content of bitumen reduced its viscosity. This observation might partly be explained by assuming that some parts of the wax dissolved in bitumen due to that most waxes have low molecular content and/or oil-based molecules (Wang et al. Citation2016). Furthermore, wax forms a homogeneous solution with bitumen and produces a significant reduction in its viscosity (Qin et al. Citation2014). The melting point of wax is around 100°C, and it is completely dissolved in bitumen at temperatures higher than 115°C (Kridan et al. Citation2011, Wasiuddin et al. Citation2012, Arshad et al. Citation2013). Hainin et al. (Citation2015) showed that wax affects the viscoelastic properties of binder differently depending on the temperature. It can decrease the viscosity of binder at high service temperatures (around 135°C), whereas it has the opposite effect at low temperatures (around 80°C) and increases the viscosity of virgin bitumen (Hainin et al. Citation2015). Thus, adding wax to bitumen will improve the viscosity during mixing (reduced viscosity) and decrease the construction temperatures.
3.1.3. Influence of wax on binder modified with optimum rubber content
Based on the PI results, the optimum rubber and wax contents were observed to be 20% and 2%, respectively. Indeed, wax decreases the risk of compaction failures, particularly when using very hard and highly viscous bitumen such as a rubber–bitumen system (Hainin et al. Citation2015). Normally, a higher viscosity value results in higher mixing and compaction temperatures and may increase the energy consumption (Xiao et al. Citation2014) and result in loss of modifying properties such as temperature sensitivity (Pyshyev et al. Citation2016). Therefore, with the aim of further enhancement in terms of PI and viscosity, a ternary blended binder through incorporating wax in a binder containing the optimum level of rubber (20%) was investigated. The results in (A) illustrate that adding wax to the rubberised binder increased the penetration slightly. This means that the hardness of rubber asphalt was slightly changed towards a softer grade. However, increasing the wax content increased the softening point, as illustrated in (B). The chemical composition of wax is described as fine crystalline materials in long-chain hydrocarbons (Hainin et al. Citation2015). This structure means that wax can adsorb saturated components (mostly wax-based or oil-based molecules) with a similar structure in a rubber–bitumen system at high temperatures (Wang et al. Citation2016). When the temperature decreases, wax and saturated components crystallise together and form a stable crystal structure, which can improve the softening point of rubberised asphalt (Wang et al. Citation2016). Significantly, the results displayed in (C) show that adding 2% wax to bitumen modified with 20% rubber considerably increased the PI value compared with the PI of solo and binary blends; the significant increase was around 168%, as shown in . However, reduction in viscosity associated with increasing wax content in a ternary blend (), was in accordance with the findings in the case of a binary blend in . This can be explained by the ability of the wax to adsorb saturated components in the rubber–bitumen binder (Wang et al. Citation2016). In addition, the aforementioned effect of the wax follows two different trends based on temperature, decreasing the viscosity at high temperatures while increasing the viscosity at low temperatures (Hainin et al. Citation2015) ().
Figure 13. Influence of wax on binder modified with 20% rubber (A) penetration test (B) softening point test (C) penetration index PI.
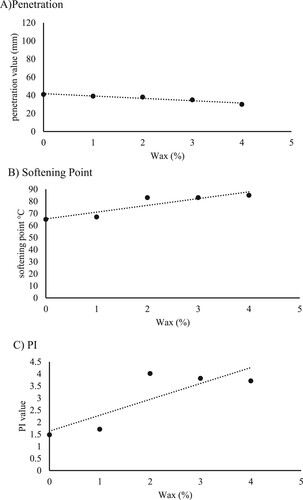
The PI value indicates the temperature sensitivity; thus, the higher PI value provided by the ternary blended binder containing 20% rubber and 2% wax showed low sensitivity to temperature, which is expected to overcome the problem related to the transportation of patching or repair materials. Asphalt mixtures containing bitumen with higher PI have a higher resistance to low-temperature cracking and permanent deformation (Ghasemi and Marandi Citation2013, Taherkhani and Afroozi Citation2016, Yaacob et al. Citation2016). In addition, wax allows for the production of rubber-modified asphalt mixes at standard temperatures. This means high-temperature melting can be avoided, thus reducing emissions and preventing binder ageing, and representing a major contribution to environmental protection and occupational health and safety. An additional benefit of the wax is that it enhances the workability and compacting properties of asphalt mixtures.
3.1.4. FTIR analysis
According to , the PMAB and the neat bitumen both have similar chemical groups, including the asymmetrical stretch (peaks 600–1700 cm−1) and the symmetric (peaks 2850–2950 cm−1). However, PMAB, as was expected, has additional peaks 1540 and 1576 cm−1 which corresponded to CH2 and C=O, respectively, compared with the peaks of the neat bitumen. This indicated chemical reactions between the compounds in PMAB (Nivitha et al. Citation2016, Weigel and Stephan Citation2017).
Based on PMB peak at 1580 cm−1 which is aromatics (Nivitha et al. Citation2016) and corresponding to C=C. This result concluded that adding wax and rubber to neat bitumen increases the aromatic content significantly. Lushinga et al. (Citation2019) and Zhang et al. (Citation2019) have concluded that the higher content of aromatic components increases the resistance to crack at low temperatures. Therefore, the high aromatic content improved low-temperature cracking performance and that indicated the binder has low-temperature sensitivity (Ghasemi and Marandi Citation2013, Taherkhani and Afroozi Citation2016, Yaacob et al. Citation2016). Furthermore, binders with high aromatic content improve asphalt mixture performance in terms of water resistance and fatigue life. Additionally, the spectra of PMB showed a couple of strong sharp peaks in the range between 1500 and 1600 cm−1. The peaks intensity is sharp, and thus, it can be directly assigned to the N–H stretch. These peaks show the Nitrogen presence as primary amines in crumb rubber (Nivitha et al. Citation2016). However, using crumb rubber as polymer modifier binder, the spectra indicate the presence of N–H stretch in the region 3280 and 3320 cm−1 as less intense (Nivitha et al. Citation2016). Hence, the results suggested that this peak has vanished; this can be explained by the fact that adding wax to polymer-modified with rubber showed a chemical reaction between wax and rubber (Butt et al. Citation2010). The wavenumber range of 1030 cm−1 characterises the functional class of sulfoxide (S=O); as expected the sulfoxide is from the rubber. The sulfoxide content in the rubber is usually in a range of 1–2% regardless of whether it is from a car or truck tire (Presti Citation2013), as adding wax to rubber modified binder did not show any increase in the sulfoxide (Butt et al. Citation2010). Additionally, the wavenumber ranges 2800–3000 cm−1 which is symmetric in neat bitumen, and PMAB represents the functional class of aliphatic C–H stretch (Nivitha et al. Citation2016). According to Khordehbinan and Kaymanesh (Citation2020), the better asphalt binder has the most flexural peaks in the wavenumber range of 400–1500 cm−1 (Khordehbinan and Kaymanesh Citation2020). Here, among compounds for neat bitumen and PMAB, the PMAB has the most flexural peaks in the wavenumber range of 1355–1588 cm−1. Hence, PMAB has a better performance than the neat bitumen.
3.1.5. X-ray diffraction (XRD) analysis
shows the X-ray diffraction for PMAB in comparison with the neat bitumen. As stated previously, the XRD method is used to chemically analyse bitumen specimens using centroid peak, peak area and peak intensity.
The response of XRD to various compounds follows an identical pattern; and thus, the centroid peak and peak area are different for the neat bitumen and PMAB, the peak intensity values are different. The wide band of 2-theta between 10 and 29 shows that the structure is amorphous and crystallised (Geçkil Citation2019).
According to , the PMAB has lower peak intensity compared to the neat bitumen. Hence, adding rubber and wax to neat bitumen declined the peak intensity considerably. This indicates that the bitumen, rubber and wax react chemically. Furthermore, the peak intensity shows the relative strength of diffraction. Lower peak intensity results in less diffraction and improves low-temperature cracking and temperature sensitivity (Khordehbinan and Kaymanesh Citation2020). The addition of 2% of wax to binder modified with 20% rubber builds 3D networks that have an interlaced form in the bitumen matrix (Khordehbinan and Kaymanesh Citation2020) as the wax has crystallises it forms (Hainin et al. Citation2015), resulting in improved temperature sensitivity (Hainin et al. Citation2015). Based on the XRD analysis, when peak intensity value decreased, the temperature sensitivity decreased as well (Geçkil Citation2019). Overall, these results indicate that the PMAB has a better performance than the neat bitumen, which is confirmed by the results of the PI and FTIR tests.
3.2. Mechanical properties assessment of mixtures using PMAB
3.2.1. ITSM test
An ITSM test was accomplished at 20°C to assess the stiffness modulus of the asphalt mixtures. As stated by previous researchers (Mashaan et al. Citation2014, Modarres and Hamedi Citation2014), polymer-modified asphalt mixtures have obtained higher stiffness than the traditional mixtures. The results of ITSM tests, as demonstrated in , shows that the RuW has a higher stiffness value (increased by 51%) than the HMA. Of note, the higher the stiffness, the better resistance to permanent deformation (Mashaan et al. Citation2014). Hence, rubber and wax have improved the mixture’s stiffness, as showed in the PI results. Moreover, the FTIR analysis confirmed that modifying bitumen with rubber and wax increased its resistance against thermal cracking and permanent deformation. Thus, the enhanced ITSM value may attribute to the increase in the adhesion of the asphalt binder with the aggregates. Furthermore, the viscosity of asphalt binder can improve the binder adhesion and cohesion, as stated by Kim et al. (Citation2015) and Presti (Citation2013), this improvement is due to the increased binder film thickness around the aggregate (as confirmed previously in the viscosity test). PMAB offers enhanced cohesion between the binder and the aggregate resulting in good adhesion between the previous surface and new layer of the patching materials once pothole or utility reinstatement repairs.
3.2.2. Wheel track test (depth rutting)
The wheel track test was used to evaluate the rutting resistance of asphalt mixtures. shows that RuW has the best rutting behaviour compared to the HMA, the rutting of RuW reduced by 23% and 57% at 45°C and 60°C, respectively.
As expected, the binder modified with rubber and wax improved the rutting resistance of asphalt mixtures (as confirmed in the PI, FTIR and XRD results). The modification of the binder caused an increase in the stiffness of the asphalt mixture; therefore, rut depth in the asphalt mixture was significantly reduced. In addition, the results of the wheel track test agree with the results of the ITSM test in that there were higher stiffness modulus values displayed by RuW, compared to that of HMA. The wheel track test outcomes suggest that modifying the neat bitumen with 20% rubber and 2% wax decreases rutting depth and the thermal sensitivity of asphalt mixtures. Indeed, using asphalt binder with high PI improved resistance to permanent deformation of asphalt mixtures (Ghasemi and Marandi Citation2013, Taherkhani and Afroozi Citation2016, Yaacob et al. Citation2016). Ultimately, modifying the neat bitumen with 20% rubber and 2% wax could provide a great alternative to the expensive materials stated by Zhu et al. (Citation2014) and Sun et al. (Citation2018), Styrene Butadiene Styrene.
3.2.3. Creep test
It can be clearly seen from that the RuW mixture has more creep resistance than the conventional HMA at the temperatures 20°C and 40°C, likely due to high values of stiffness which strengthens bonds between aggregate particles and asphalt binder. Also, the RuW mixture absorbs or distracts some amount of energy applied by static load, recording high recovery (the relaxation) compared to the HMA. This might be due to the role the rubber plays in the modified binder in creating a three-dimensional polymer network (Wang et al. Citation2020), which prevents or interrupts the formation of microcracks under loading, also wax at temperatures below 100°C forms a crystalline lattice structure in the binder which acts as the basis for the increased resistance (Hurley and Prowell Citation2005, Wasiuddin et al. Citation2012). Furthermore, the increase in the testing temperature caused by the stiffening effect of the RuW mixture is higher compared to the HMA. In addition, it is considered that PMAB has improved RuW mixture when compared to elasticity behaviour of RuW at 20°C and 40°C; it can be noticed that the mixture has less temperature sensitivity (as confirmed by PI results) (Ghasemi and Marandi Citation2013, Taherkhani and Afroozi Citation2016, Yaacob et al. Citation2016). Therefore, as expected, modifying the bitumen with rubber and wax increases the asphalt mixtures’ elasticity and reduces the permanent deformation (Ghasemi and Marandi Citation2013, Sitinamaluwa and Mampearachchi Citation2014).
3.2.4. Four-point bending test (fatigue cracking resistance)
shows that the highest fatigue life is seen in the RuW mixture compared with the HMA mixture, and thus resulting in improved behaviour against fatigue. This result was based on controlled strain criteria (150 microstrains). There was a 39% rise in the fatigue life of the RuW mixture compared to that of the HMA mixture, this is due to the significant improvement in the cohesive features of the asphalt mixture.
This improvement of the fatigue life is because of increased adhesive force between the aggregates and PMAB. The chemical-based additive improves the fatigue resistance of the base bitumen owing to the 3-D polymer network of the modified binder (Wang et al. Citation2020), delaying or preventing the micro-cracks formation under fatigue loading. Moreover, aggregate particles have a stronger bond with the binder as the thicker modified binder surrounds the aggregates (Presti Citation2013, Kim et al. Citation2015); thus the performance of the RuW mixture is improved when compared to the HMA mixture. The stiffness of the RuW may offer extra stable cohesion that creates an improved, reinforcing factor (Ghasemi and Marandi Citation2013, Sitinamaluwa and Mampearachchi Citation2014), resulting in enhanced cohesive characteristics of the asphalt matrix. This test has only investigated the effect of loading rate on asphalt mixture with 150 microstrains and 50% reduction in the initial stiffness level at 20°C corresponding fatigue life. Both fatigue life and strength of asphalt mixtures are affected by several test conditions, including test temperature, rate of the initial stiffness, microstrains in addition to other factors. At some point, these conditions must be considered while evaluating the fatigue life of the asphalt mixtures.
3.3. Durability performance of mixtures prepared with PMAB
3.3.1. Water sensitivity test
The stiffness modulus ratio (SMR) of RuW and HMA mixtures are demonstrated in . The RuW has a slightly higher SMR compared to the HMA mixture, resulting in improved adhesion characteristics of PMAB with aggregate due to the positive effect of high viscosity of PMAB (as confirmed by the viscosity test). The modification of bitumen with rubber and wax has improved RuW water sensitivity by around 6% compared to HMA. Improved cohesion and adhesion are mostly responsible for this enhanced performance (Cui et al. Citation2014), against the water action in the RuW mixture. As modified binder has a resilient bond with aggregate, generating improved resistance to water action (Cui et al. Citation2014, Omar et al. Citation2020).
3.3.2. Freeze and thaw performance
Stiffness of RuW and HMA mixtures along with SMR ratio present almost similar drop-trend with the rise of freeze and thaw cycles as demonstrated in .
Figure 23. Comparative results of the ITSM and SMR of the RuW and the HMA mixtures under various freeze and thaw cycles.
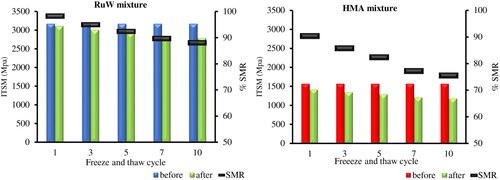
This is due to the viscosity of asphalt binder deteriorating when the number of freeze–thaw cycles increased (Mohi et al. Citation2020), thus, stiffness of asphalt mixtures reduced (Mohi et al. Citation2020, Vega-zamanillo et al. Citation2020). However, the RuW mixture has recorded a higher SMR ratio in comparison with the HMA mixture during all freeze and thaw cycles. This is due to the modified asphalt binder having higher viscosity (as resulted in viscosity test previously) than the bitumen; therefore, the cohesion of the modified binder and its adhesion with aggregate is stronger than that of neat bitumen and HMA mixture, respectively. Therefore, the RuW mixture resulted in better performance during freeze and thaw cycles. This is an evident influence of modified asphalt binder with rubber and wax to improve the binder viscosity and temperature sensitivity.
4. Conclusions
The work presented in this paper shows the results of developing a binder with minimal temperature sensitivity and enhanced viscoelastic properties for hand-laid application in low volumes for emergency repair. A series of experiments were performed to assess the temperature sensitivity of the modified binder by measuring the PI, FTIR and XRD of the modified binder and mixture. The results of asphalt binder and mixture tests showed that:
In terms of asphalt binder evaluation, modifying the binder with rubber and wax decreases the penetration and increases the softening point. This suggests that the PMAB is more resistant to flow and stiffer compared to the neat bitumen. The effect of the rubber content was significant; when mixed with the neat bitumen there were considerably higher levels of viscosity. Conversely, adding wax reduced the viscosity value. Adding 2% wax to bitumen modified with 20% rubber was observed to have a significant impact on the penetration and softening point of the binder, resulting in greater PI value improving the rheology of the binder at ambient and melting temperatures. Thus, the resultant binder can be used for patching materials, which is expected to overcome the problem related to transportation. Based on the FTIR and XRD analysis, it is indicated that the neat bitumen, rubber and wax react chemically to build 3D networks that have an interlocked structure in the bitumen matrix resulting in reduced temperature sensitivity of the PMAB.
In terms of asphalt mixture evaluation, the stiffness modulus of the RuW mixture increased by 51% compared to the conventional HMA mixture. Moreover, the experimental outcomes of the wheel track test, creep test and fatigue life test revealed an enhancement in the asphalt mixture due to the development of better adhesion between the aggregates. This is due to the increased viscosity of the modified binder (PMAB), which led to increasing the film surrounding the aggregates. This may enhance a good adhesion between old surface and patching materials (new layer) once pothole or utility reinstatement repairs.
From a durability perspective, the RuW mixture indicated a better resistance to water sensitivity along with freeze and thaw cycle.
Ultimately, based on the results of this research, modifying the asphalt binder with 20% rubber and 2% wax resulted in a stronger, more durable asphalt mixture in comparison to the HMA mixture.
Disclosure statement
No potential conflict of interest was reported by the author(s).
Additional information
Funding
References
- Abed, Y.H., and Abedali Al-Haddad, A.H.A, 2019. Temperature susceptibility of modified asphalt binders. IOP Conference Series: Materials Science and Engineering, 671, 012121.
- Al-Hadidy, A.I., and Yi-qiu, T, 2009. Effect of polyethylene on life of flexible pavements. Construction and Building Materials, 23 (3), 1456–1464.
- Al-Hdabi, A., 2014. High strength cold rolled asphalt surface course mixtures. (May), 267.
- Al-Hdabi, A., Al Nageim, H., and Seton, L., 2014. Superior cold rolled asphalt mixtures using supplementary cementations materials. Construction and Building Materials, 64, 95–102.
- Alhumaidan, F.S., et al., 2015. Changes in asphaltene structure during thermal cracking of residual oils: XRD study. Fuel, 150, 558–564.
- Arshad, A.K., et al., 2013. The effects of sasobit® modifier on binder at high and intermediate temperatures. International Journal of Engineering and Advanced Technology (IJEAT), 2 (3), 2249–8958.
- Behnood, A, 2019. Application of rejuvenators to improve the rheological and mechanical properties of asphalt binders and mixtures: A review. Journal of Cleaner Production, 231, 171–182.
- Biswas, S., Hashemian, L., and Bayat, A, 2018. Investigation of pothole severity and maintenance methods in Canada through questionnaire survey. Journal of Cold Regions Engineering, 32 (2), 04018002.
- Butt, A.A., et al., 2010. The effect of wax modification on the performance of mastic asphalt. International Journal of Pavement Research and Technology, 3 (2), 86–95.
- Carruth, W.D., and Santiago, M.M, 2015. Hot in-place asphalt recycling for small repairs on airfields in remote settings. International Journal of Pavement Research and Technology, 8 (6), 395–402.
- Cong, L., et al., 2020. Experimental investigation on performance deterioration of asphalt mixture under freeze–thaw cycles. International Journal of Transportation Science and Technology, 9 (3), 218–228.
- Cui, S., et al., 2014. Durability of asphalt mixtures: effect of aggregate type and adhesion promoters. International Journal of Adhesion and Adhesives, 54, 100–111.
- Dulaimi, A., et al., 2017. High performance cold asphalt concrete mixture for binder course using alkali-activated binary blended cementitious filler. Construction and Building Materials, 141, 160–170.
- Dulaimi, A., et al., 2020. An evaluation of the performance of hot mix asphalt containing calcium carbide residue as a filler. Construction and Building Materials, 261, 119918.
- European Committee for Standardization, 2007. Bituminous Mixtures –Test Methods for Hot Mix Asphalt – Wheel Tracking Test Methods for Hot Mix Asphalt. London, UK, 3 (1).
- European Committee for Standardization, 2009. Bitumen and bituminous binders - specifications for paving grade bitumens. London: British Standards Institution.
- European Committee for Standardization, 2012. BSI Standards Publication Tests for geometrical properties of aggregates Part 1 : Determination of particle size distribution — Sieving method. British Standards Institution, London, UK, BS EN 933–.
- European Committee for Standardization, 2015a. Bitumen and bituminous binders – determination of bitumen and bituminous binders — Determination of needle penetration. London: British Standards Institution.
- European Committee for Standardization, 2015b. Bitumen and bituminous binders – determination of the softening point. Ring and Ball method. London: British Standards Institution.
- European Committee for Standardization, 2015c. Bitumen and bituminous binders – specifications for paving grade bitumens -annex A (normative) calculation of the penetration index. London: British Standards Institution.
- European Committee for Standardization, 2016a. BSI Standards Publication Bituminous mixtures — Material specifications Part 1 : Asphalt Concrete. British Standards Institution, London, UK, BS EN 1310.
- European Committee for Standardization, 2016b. PD 6691 : 2016 BSI Standards Publication PUBLISHED DOCUMENT Guidance on the use of BS EN 13108 Bituminous mixtures – Material specifications. London, UK.
- European Committee for Standardization, 2017. Part 12, Bituminous Mixtures-Test Methods for Hot Mix Asphalt-Determination of the Water Sensitivity of Bituminous Specimens. British Standards Institution, London, UK.
- European Committee for Standardization, 2018a. Bitumen and bituminous binders – determination of dynamic viscosity of bituminous binder using a rotating spindle apparatus. London: British Standards Institution.
- European Committee for Standardization, 2018b. BS EN 12697-26 : 2018 BSI Standards Publication Bituminous mixtures - Test methods. British Standards Institution, London, UK.
- Fakhri, M., Ali, S., and Aliha, M.R.M, 2020. Impact of freeze–thaw cycles on low temperature mixed mode I/II cracking properties of water saturated hot mix asphalt: An experimental study. Construction and Building Materials, 261, 119939.
- Feng, D., et al., 2010. Impact of salt and freeze–thaw cycles on performance of asphalt mixtures in coastal frozen region of China. Cold Regions Science and Technology, 62 (1), 34–41.
- Fiber, B., et al., 2018. Comparative study on the damage characteristics of asphalt mixtures reinforced with an eco-friendly Basalt Fiber under freeze-thaw cycles. Materials, 11 (2488), 1–17.
- Geçkil, T, 2019. Physical, chemical, microstructural and rheological properties of reactive terpolymer-modified bitumen. Materials, 16 (6), 13–17.
- Ghasemi, M., and Marandi, S.M, 2013. Laboratory studies of the effect of recycled glass powder additive on the properties of polymer modified asphalt binders. International Journal of Engineering, Transactions A: Basics, 26 (10), 1183–1190.
- Ghuzlan, K.A., and Al Assi, M.O., 2017. Sasobit-Modified asphalt binder rheology. Journal of Materials in Civil Engineering, 29 (9), 04017142.
- Hainin, M.R., et al., 2015. An overall review: modified asphalt binder containing sasobit in warm-mix asphalt technology. Jurnal Teknologi, 73 (4), 1–6.
- Hurley, G.C., and Prowell, B.D., 2005. Evaluation of sasobit for use in warm mix asphalt. NCAT report. Auburn.
- Jamshidi, A., Hamzah, M.O., and You, Z, 2013. Performance of warm Mix asphalt containing sasobit®: state-of-the-art. Construction and Building Materials, 38, 530–553.
- Khordehbinan, M., and Kaymanesh, M.R, 2020. Chemical analysis and middle-low temperature functional of waste polybutadiene rubber polymer modified bitumen. Petroleum Science and Technology, 38 (1), 8–17.
- Kim, H.H., et al., 2015. Viscosity-temperature characteristics of warm mix asphalt binder with sasobit®. Construction and Building Materials, 95 (4), 1–6.
- Kishchynskyi, S., Nagaychuk, V., and Bezuglyi, A, 2016. Improving Quality and Durability of Bitumen and Asphalt Concrete by Modification Using Recycled Polyethylene Based Polymer Composition, 143, 119–127.
- Kridan, F.A.M., Arshad, A.K., and Rahman, M.Y.A., 2011. The effect of warm mix asphalt additive (sasobit®) on determination of optimum bitumen content. International Journal of Research & Reviews in Applied Sciences, 6 (4), 400–406.
- Lee, S.-J., et al., 2011. Aging analysis of rubberized asphalt binders and mixes using gel permeation chromatography. Construction and Building Materials, 25 (3), 1485–1490.
- Li, P., et al., 2018. Analysis of viscosity and composition properties for crumb rubber modified asphalt. Construction and Building Materials, 169, 638–647.
- Lushinga, N., Cao, L., and Dong, Z, 2019. Effect of silicone oil on dispersion and low-temperature fracture performance of crumb rubber asphalt. Advances in Materials Science and Engineering, 2019, 1–12.
- Mashaan, N.S., et al., 2011. Effect of crumb rubber concentration on the physical and rheological properties of rubberised bitumen binders. International Journal of the Physical Sciences, 6 (4), 684–690.
- Mashaan, N.S., et al., 2014. A review on using crumb rubber in reinforcement of asphalt pavement. The Scientific World Journal, 2014 (214612), 21.
- Modarres, A., and Hamedi, H, 2014. Effect of waste plastic bottles on the stiffness and fatigue properties of modified asphalt mixes. Materials and Design, 61, 8–15.
- Mohi, I., et al., 2020. Effect of freeze-thaw cycles on the properties of asphalt pavements in cold regions: a review. Transportation Research Procedia, 48, 3634–3641.
- Nassar, I.M.K.I., and Ibrahim, K, 2012. Evaluation of the effect of waste polystyrene on performance of asphalt binder. ARPN Journal of Science and Technology, 2 (2225–7217), 927–935.
- Nivitha, M.R., Prasad, E., and Krishnan, J.M, 2016. Ageing in modified bitumen using FTIR spectroscopy. International Journal of Pavement Engineering, 17 (7), 565–577.
- Omar, H.A., et al., 2020. Effects of moisture damage on asphalt mixtures. Journal of Traffic and Transportation Engineering (English Edition), 7 (5), 600–628.
- Polacco, G., et al., 2015. A review of the fundamentals of polymer-modified asphalts: asphalt/polymer interactions and principles of compatibility. Advances in Colloid and Interface Science, 224, 72–112.
- Porto, M., et al., 2019. Bitumen and bitumen modification: A review on latest advances. Applied Sciences (Switzerland), 9 (4).
- Presti, D. Lo, 2013. Recycled tyre rubber modified bitumens for road asphalt mixtures: A literature review. Construction and Building Materials, 49, 863–881.
- Pyshyev, S., et al., 2016. Polymer modified bitumen: review. Chemistry and Chemical Technology, 10 (4), 631–636.
- Qin, Q., et al., 2014. Morphology, thermal analysis and rheology of Sasobit modified warm mix asphalt binders. Fuel, 115, 416–425.
- Read, J., Whiteoak, D., and Hunter, R, 2003. The shell bitumen handbook. 5th ed. London: Thomas Telford Publishing.
- Saeed, A., and Hammons, M.I., 2009. Airfield repairs in austere locations using pelletized asphalt technology. In: Transportation Research Board 89th Annual Meeting. Washington, DC: National academies of sciences engineering and medicine, 10–3163.
- Shanbara, H.K., Ruddock, F., and Atherton, W, 2018. A laboratory study of high-performance cold mix asphalt mixtures reinforced with natural and synthetic fibres. Construction and Building Materials, 172, 166–175.
- Si, W., et al., 2015. Impact of freeze-thaw cycles on compressive characteristics of asphalt mixture in cold regions. Journal of Wuhan University of Technology-Mater. Sci. Ed., 30 (4), 703–709.
- Sitinamaluwa, H.S., and Mampearachchi, W.K, 2014. Development of a polymer-modified bitumen specification based on empirical tests – case study for Sri Lanka. Road Materials and Pavement Design, 15 (3), 712–720.
- Suganpriya, V., Omprakash, S., and Chandralega, V, 2016. Study of behaviour of bitumen modified with crumb rubber. International Journal of Engineering Research, 5 (5), 192–197.
- Sun, M., et al., 2018. Performance of polyurethane modified asphalt and its mixtures. Construction and Building Materials, 191, 386–397.
- Taherkhani, H., and Afroozi, S, 2016. The properties of nanosilica-modified asphalt cement. Petroleum Science and Technology, 34 (15), 1381–1386.
- Vega-Zamanillo, Á., Juli-Gándara, L., Calzada-Pérez, M.Á, and Teijón-López-Zuazo, E., 2020. Impact of temperature changes and freeze—thaw cycles on the behaviour of asphalt concrete submerged in water with sodium chloride.. Applied Sciences, 10 (4), 1241.
- Wang, H., et al., 2012. Laboratory evaluation on high temperature viscosity and low temperature stiffness of asphalt binder with high percent scrap tire rubber. Construction and Building Materials, 26 (1), 583–590.
- Wang, H., et al., 2020. Fatigue performance of long-term aged crumb rubber modified bitumen containing warm-mix additives. Construction and Building Materials, 239, 117824.
- Wang, L., Wei, J., and Zhang, Y, 2009. Development of alternative parameters to evaluate the temperature susceptibility of asphalt binders. International Journal of Pavement Research and Technology, 2 (2), 75.
- Wang, T., et al., 2016. Effects of Sasobit and its adding process on the performance of rubber asphalt. Chemical Engineering Transactions, 51 (2015), 181–186.
- Wasiuddin, N., et al., 2012. Effects of temperature and shear rate on viscosity of Sasobit® -modified asphalt binders. International Journal of Pavement Research and Technology, 5 (6), 369–378.
- Weigel, S., and Stephan, D, 2017. The prediction of bitumen properties based on FTIR and multivariate analysis methods. Fuel, 208, 655–661.
- Wu, S.P., et al., 2008. Temperature sensitivity of asphalt-aggregate adhesion via dynamic mechanical analysis. Key Engineering Materials, 385–387, 473–476.
- Xiao, F., et al., 2014. Rheological property investigations for polymer and polyphosphoric acid modified asphalt binders at high temperatures. Construction and Building Materials, 64, 316–323.
- Xiao, F., Wenbin Zhao, P.E., and Amirkhanian, S.N, 2009. Fatigue behavior of rubberized asphalt concrete mixtures containing warm asphalt additives. Construction and Building Materials, 23 (10), 3144–3151.
- Yaacob, H., et al., 2016. Rheological properties of styrene butadiene rubber modified bitumen binder. Jurnal Teknologi (Sciences & Engineering), 78 (7–2), 121–126.
- Yuan, W., et al., 2012. DCPD resin catalyzed with Grubbs catalysts for reinforcing pothole patching materials. In: A.L. Gyekenyesi, ed. 8347, Nondestructive Characterization for Composite Materials, Aerospace. San Diego, California, United States: Engineering, Civil Infrastructure, and Homeland Security, 83471D.
- Zhang, R., et al., 2019. The impact of bio-oil as rejuvenator for aged asphalt binder. Construction and Building Materials, 196, 134–143.
- Zhao, G., and Guo, P, 2012. Workability of Sasobit warm mixture asphalt. Energy Procedia, 16, 1230–1236.
- Zhu, J., Birgisson, B., and Kringos, N, 2014. Polymer modification of bitumen: advances and challenges. European Polymer Journal, 54 (1), 18–38.