ABSTRACT
Empirical evidence suggests a phonological loop deficit associated with Down syndrome. A trisomy 21 may be associated with a narrowing of visual attention to fewer than four objects at a time too. In a study with computer tachistoscopy, the hypothesis was confirmed in all 194 persons with trisomy 21. The subitising limit of persons with trisomy 21 thus has a value that lies between the value of neurotypical persons and persons with simultanagnosia. A fitting term would therefore be ‘simultandysgnosia’. Consideration of this dysgnosia could prove to be a key to learning disabilities in mathematics in particular and learning disabilities in general. Diagnostic and learning methods should consider this limitation of the attention span not only in verbal but also in visual tasks.
Introduction
Due to their abstraction, mathematical operations can relieve the working memory immensely. But when people learn these operations, they bring their working memory to its limits. This applies to both verbal and visual working memory.
Campos, Almeida, Ferreira, Martinez, and Ramalho (Citation2013, p. 421) state: ‘[…] we conclude that working memory (WM) assumes an important role in different math curricular achievements.’ They found by using arithmetic story problems as the dependent variable, that working memory components accounted for more than 50 percent of the variance (Campos et al., Citation2013, pp. 429–430). As children improve their working memory through exercise, they can better understand the counting process mentally and gradually renounce on fingers as a memory aid (Geary, Citation2006).
Baddeley and Jarrold (Citation2007) investigated the influence of trisomy 21 on working memory. Baddeley's concept of a multicomponent working memory offers a model that is the most popular way of conceptualising the attentional interface between perception and memory. He suggests a phonological loop deficit associated with Down syndrome. A plausible explanation for this is provided by empirical evidence identifying limited verbal short-term memory and working memory for longer words and larger phrases in people with trisomy 21 (Vicari, Marotta, & Carlesimo, Citation2004).
As a strength, Bellugi and George (Citation2001, p. 25) emphasise in persons with trisomy 21 language comprehension. They observed that individuals with trisomy 21 are more prone to short and tight three to five-word sentences and have less sensitivity to grammatical details. These findings were confirmed by studies by Abbeduto, Warren, and Conners (Citation2007). Witecy and Penke (Citation2016, Citation2017) asked whether linguistic impairment in children with trisomy 21 was more of a developmental delay or a speech disorder. More precisely: Can the observed language problems of people with trisomy 21 be attributed solely to their limited cognitive abilities or is there, in addition to the cognitive impairment, an additional disturbance of speech development?
The results speak for two different groups: firstly speech-impaired and secondly speech-unaffected children with trisomy 21. Only in the first group do linguistic development and understanding remain behind cognitive development. However, the study of Witecy and Penke (Citation2016, Citation2017) could not reproduce a better understanding of speech with lower speech capacity.
Baddeley’s conclusion is: ‘However, whether such a deficit is particular to the condition, or rather a general corollary of the learning difficulties associated with Down syndrome, remains an open question’ (Baddeley & Jarrold, Citation2007, p. 928).
Conversely, we believe that the limited capacity of working memory is an important symptom of a type of learning difficulty that is regularly associated with trisomy 21 not only in speech development. This symptom is particularly evident in learning difficulties in mathematics. We assume that the findings about working memory associated with Down syndrome have a deeper cause that universally influences all forms of working memory – not only the phonological loop.
For example, we investigated, how many elementary movements persons with trisomy 21 can simultaneously and successively imitate. In all of the 713 test subjects with trisomy 21 there was a limit to the number of correctly imitated elementary movements. Whereas neurotypical subjects reached their limit by imitating four elementary movements, participants with trisomy 21 could imitate only two elementary movements (Zimpel & Röhm, Citation2018).
Learners with trisomy 21 who participated in various mathematics projects at the University of Hamburg displayed unique characteristics during subitising. It was observed over and over that learners counted amounts of three to four objects individually. It was obvious that they could not judge the amount at a glance. With more training, they learn to gauge the quantities successfully. From time to time, however, they still misjudge numbers of four or five elements (Zimpel, Citation2013, pp. 36–37).
The peculiarity in subitising of students with trisomy 21 fits well with Montoya’s et al. (Citation2014, p. 156) findings that increased gene expression by sections of chromosome 21 exist in the hippocampus. The database is the Brain Atlas of the Allen Institute for Brain Sciences (http://human.brain-map.org). Focus of gene expression (of chromosome 21) are the pyramidal cells of Ammon’s horn (cornu Ammonis). The neurons in this section are affecting learning in connection with subitising. These neurons include the Schaffer collaterals involved in long-term potentiation (Squire & Kandel, Citation1999, p. 122.).
The Schaffer collaterals are responsible for immediate memory (ultra-short-term memory) that connects the brain with the here and now, the present moment: ‘It is the information that is currently the focus of attention and occupies the stream of thoughts. The capacity of immediate memory is quite limited […]’ (Squire & Kandel, Citation1999, p. 92.).
Schneider and Deubel (Citation2000) found four units as a limit for the simultaneous recording of neurotypical persons. A historical analysis of the development of the notation of numerals (e.g. Roman, Chinese and Indian numerical notations) as well as ontogenetic and intercultural comparative studies suggest an optimal bundling of signs in three to four units (Zimpel, Citation2008, pp. 33–44). Dehaene (Citation1997, p. 71) and Dehaene and Cohen (Citation1994) also call this limit the ‘subitising limit’. Behind this limit, the approach to numbers begins by bundling into figures or with more or less skilful estimate.
Baddeley’s (Citation2007) findings that a phonological loop deficit is associated with Down syndrome could be a hint to special pre-attentional mechanisms independent from sensory modalities. Trick and Pylyshyn (Citation1994) suspect such a pre-attentional processing mechanism with strict capacity limitation to a maximum of 4 items in neurotypical persons. This subitisation limit is also known in investigations with auditory (Repp, Citation2007) and haptic (Plaisier, Bergmann Tiest, & Kappers, Citation2009) stimuli.
If the subitising limit decreases to no more than one object at a time that is called ‘simultanagnosia’. For example, that is the case with the Bálint’s syndrome. This neurological disorder is characterised by the inability of an individual to perceive more than one single object at a time.
A metaphor for simultanagnosia is the constriction of the visual ‘window’ of attention ‘[…] with little or no understanding of the fragmented world they perceive. Their perception is often “captured” by local elements of a scene, in that they report local details of a scene or object at the expense of the global whole […]’ (Dalrymple, Birmingham, Bischof, Barton, & Kingstone, Citation2011, p. 265).
There are different categories of simultanagnosia. An additional category could be associated with trisomy 21. We have conducted studies with Navon (Citation1977, Citation1981) figures. The results: people with trisomy 21 tend at one moment to neglect details in favour of the overall form and to highlight a detail in the next moment in order to abstract from the overall form (Zimpel, Citation2013, pp. 36–37, Citation2016, pp. 96–99).
The difficulty of switching between two aspects recalls the symptoms that Lurija (Citation1970, pp. 181–185; Citation1992, p. 122) described in patients with simultanagnosia: Either they could direct their attention to the pen, then the line they wanted to write slipped out of the field of attention, or they focused on the line and lost sight of the tip of the pen. If they wanted to draw a circle, they could concentrate only on either the centre or the line to be drawn with the pen, and so on.
Hoffmann (Citation2003, pp. 111–112) describes the difficulty of switching between two aspects, using the example of a woman with simultanagnosia: ‘When she was shown a jug of water poured into a glass, she first noticed the handle and said “suitcase”. She was asked to look again and recognised the glass. As she remembered the handle, she looked down until she saw the pitcher (now with its handle).’
According to Huberle and Karnath (Citation2006, p. 234), it is known that in this syndrome, affected persons cannot perceive more than one object at a time. However, bundling into larger units is still possible because short words or even short sentences can be read without error in this syndrome. The same effect can be caused by a series of lines or points arranged in a geometric shape.
Clements (Citation1999, pp. 401–402) calls this bundling of numbers into figures like numbers on dice, domino, etc. ‘conceptual subitising’ and the recognising of a number without use of bundling processes ‘perceptual subitising’. According to this distinction, we observed in persons with trisomy 21 (like in persons with simultanagnosia) that in the identification of small numbers, conceptual subitising is easier for them than perceptual subitising. This observation might explain some of the learning problems of people with trisomy 21 in mathematics, since numbers are based on an abstraction of the arrangement of the elements of a set.
Huberle and Karnath (Citation2006, pp. 905–911) came with Navon figures to the experimental conclusion that even in a simultanagnosia the partial-whole consideration does not prove to be an either-or-question. Sometimes patients with simultaneous diagnosis neglect details in favour of the overall shape in one moment and they highlight a detail in the next moment whereas neglecting the overall shape.
Bellugi and George (Citation2001, p. 62) came to the experimental result that people with trisomy 21 would more likely perceive the overall shape in Navon figures and tend to overlook details. Experimentally, there are also opposite findings (Zimpel, Citation2013). We expect people with trisomy 21 to have special difficulties in capturing interference images. This refers to images in which several dice point images are arranged so that their overall image again results in a dice point image. Studies with computer tachistoscopy have already demonstrated this particular difficulty for 19 participants with a trisomy 21 (Zimpeltachistoscopy in 19 participants with trisomy, Citation2013).
A consistent explanation of these results could be: A trisomy 21 is regularly accompanied by a special category of simultanagnosia. This category associated with trisomy 21 means a restriction of subitising to less than four objects at the same time. In a strong sense, it is therefore not an ‘agnosia’ but a ‘dysgnosia’. To get a deeper understanding of the learning difficulties of people with trisomy 21, the following hypothesis emerged: A trisomy 21 is regularly accompanied by a simultandysgnosia.
Why is a verification of this hypothesis of particular importance? Having different attentional characteristics inevitably leads to learning difficulties when people are forced to learn at the same pace. The majority of recommended support measures for trisomy 21 learners found in special needs education are based on visual support (Bird & Buckley, Citation2000; Oelwein, Citation2007; Wilken, Citation2014). The reason for this is presumably that mainly visual cognitive strengths are suspected for humans with trisomy 21. If one asks in pedagogical literature for cognitive strengths, one finds again and again statements, like these: ‘Most children with Down syndrome, which I met, are visual learners’ (Oelwein, Citation2007, p. 51). Additionally, it is frequently pointed out in the literature that persons with a trisomy 21 show better results in visual tasks compared to persons with Williams-Beuren syndrome (Vicari, Bellucci, & Carlesimo, Citation2006). As a strength, Bellugi and George (Citation2001, p. 25) emphasise in persons with trisomy 21 (in addition to language comprehension) their spatial orientation and their supposedly typical view of the big picture.
If the hypothesis of a simultandysgnosia in trisomy 21 were confirmed, diagnostic and learning methods should consider this limitation of the attention span not only in verbal but also in visual tasks. This is particularly important for mathematics lessons, because linguistic-algorithmic and figurative-geometric contents are in close interaction.
Preliminary Investigations
The hypothesis that a trisomy 21 is associated with a narrowing of visual attention to fewer than four objects at the same time (simultandysgnosia) has already been confirmed by a small study with computer tachistoscopy in 19 participants with trisomy 21 (Zimpel, Citation2013). In this article we are reporting the results of a ten times larger study with 194 participants with trisomy 21.
In the preliminary investigations, we found that all persons with trisomy 21 who participated in the investigation were very familiar with dice point images. In the case of a simultandysgnosia, it would be expected that there would be no problems with dice point images, since this is a form of ‘conceptual subitising’.
As a result of many experiments on students without trisomy 21, the calibration of the four-unit measurement method was performed based on the experiments of Schneider and Deubel (Citation2000). Limiting the presentation time of the arrangements on the computer screen to precisely 250 milliseconds proved sufficiently accurate. The participants themselves can determine the duration of the break between each presentation in this procedure.
Variations of the experimental conditions showed that random arrangements of the squares could cause spontaneous shape impressions. For example, three points were spontaneously seen as a triangle, four points as a kite quad, or five points as the letter ‘M’, thus reducing the total number of visible units. Students showed experimentally that the estimates became more accurate when they knew the maximum number of squares displayed. For example, in some arrangements the maximum is twenty. Some students indicated that they then actively searched for four times five squares in large quantities and estimated how many squares were missing from 20.
The problem of bundling elements into figures has been known since the experiments with the tachistoscope by Wundt (Citation1901, pp. 254–255; Citation1913, pp. 255–260): Wundt’s participants simultaneously recorded up to six simple dashes, numbers or letters. For senseless syllables, on the other hand, they were able to capture six to ten letters simultaneously. Proverbs, such as ‘better late than never’ or ‘there’s no place like home’, raised the volume of attention to four to five short words, that is, twenty to thirty letters. In an effort to eliminate conceptual subitising, Wundt came to an attention span for the tactile and visual sense of four to six units. Also from the studies on the historical development of figures from tally marks (Zimpel, Citation2008, pp. 33–44) is known: Five units create the need for bundling. Even five dashes (IIIII) are easier to detect in two bundles (II III).
To get a deeper understanding of the learning difficulties of people with trisomy 21, the following hypothesis emerged: A trisomy 21 is regularly accompanied by a smaller attention span.
Methods
We used computer tachistoscopy to investigate,
Whether the persons of the experimental group recognise the numbers on conventional dice point images and higher numbers, which are arranged in a similar pattern, just as fast (in 250 milliseconds) as the persons of the comparison group,
Whether the subjects of the experimental group have significantly more difficulty in simultaneously recording the overall shape and details than the subjects of the comparison group,
Whether errors in the number of small squares in randomly arranged square clouds, which are displayed only 250 milliseconds, occur in the subjects of the experimental group even at significantly smaller amounts than in the persons of the comparison group and,
Whether errors in the indication of the number of dashes in a row occur in the persons of the experimental group even at significantly smaller dash amounts than in the persons of the comparison group.
Participants
Both groups – experimental group (with trisomy 21) and comparison group (neurotypical persons) – involved participants of school age and adulthood with a developed number concept and the ability to count disordered quantities with more than 20 elements.
Requirement for participating in the experiment was not the IQ, but the ability to count disordered quantities with more than 20 elements and a developed number concept. The number concept was checked before with Piaget experiments on seriation and class inclusion: sorting disorderly quantities by size, assigning digits to disordered sets, comparing quantities with different distances between the single elements etc.
Our main criterion for a developed number concept was the understanding of the invariance principle for numbers, the logical concept of quantity conservation, that occurs between 5 and 8 years of age (Piaget & Szeminska, Citation1965, p. 10).
Even though the participants in the experimental group usually develop a number concept only at an older age, a 6-year-old girl with trisomy 21 already met the necessary requirements at the time of the examination. Although her IQ score was two standard deviations below norm, she was very interested in numbers and benefited from a dedicated support of her parents.
The recruitment of the participants took place via self-help organisations and educational institutions. There were 194 participants with trisomy 21 in the experiments, including 98 female and 96 male. As a comparison group, a further 280 people without trisomy 21 were added, especially parents (32 percent), siblings (15 percent) and students (20 percent). Of these participants, 152 were female and 128 male
The IQ was determined for the individuals included in the comparison group with Coloured/Standard Progressive Matrices (CPM, SPM). The IQ lies between average to above average. The average age in the comparison group with neurotypical individuals was 17.5 years (minimum 6 years and maximum 82 years). This might seem very broad, but the 82-year-old man fulfilled all the criteria for participation and was very motivated.
The average age in the experimental group with trisomy 21 was 19.5 years (minimum 6 years and maximum 53 years). The average value of the CPM-score (Coloured Progressive Matrices) of the participants with trisomy 21 (test group) is 32 (minimum: 30, maximum: 35). The IQ values of the participants in the experimental group are significantly below the normal range (IQ between 50 and 75). Thus, the diagnosed learning difficulties are in the lower range of intellectual disability, mild intellectual disability (ICD-10 F70) and in the upper range of moderate intellectual disability (ICD-10 F71). Development effects cannot be definitively clarified based on the available data, because all participants of the test class were educated according to special guidelines at special schools or in integration classes.
Experimental Procedure
The presentation of different quantities lasted only a quarter of a second. This is an established empirical method for estimating the subitising limit. The short-term performance is ideally designed to prevent, or at least significantly complicate, complex bundling techniques. We used this method of computer tachistoscopy to present differently grouped numbers, concretely: for the presentation of dice point images, interference pictures, clouds of squares and strips of dashes.
Participants could choose to name the number verbally, type the number into the computer, or point to the number on a number table. The duration of all four experiments was between 15 to 25 minutes. There is only one attempt, since the computer programme is without a return button. The time for the decision after the image display was not limited.
Procedure for Experiment 1: Dice Point Images
With dice point images from one to six, the simultandysgnosia should fall away as an obstacle to the recognition of the numbers. As a control, we added to the arrangements with dice point images of one to six additional analogue-style arrangements of seven and eight points as shown in .
Procedure for Experiment 2: Interference Pictures
The interference images selected, like Navon figures, contain an opposition between details and the whole as shown in .
Figure 2. 16 arrangements, each presented exactly 250 milliseconds on the computer screen, in the second experiment ‘interference pictures‘.
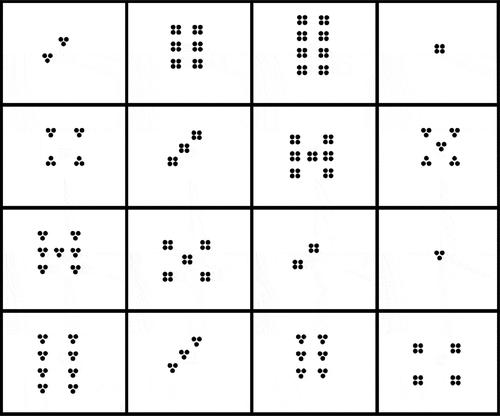
All participants were asked to indicate both factors (for example: ‘four times four’ or ‘three and five’ or ‘two – three’, etc.). Mathematically incorrect phrasing and the order of multiplicand and multiplier didn’t matter (see ). For this, it is not necessary to understand the multiplication language. Nevertheless the participants were allowed to call the total number (the product). The task requires switching back and forth between the overall figure and its parts.
Procedure for Experiment 3: Clouds of Squares
The aim of the third experiment was to make bundling and shape effects more difficult. In particular, we found disordered sets of small squares (clouds of squares) as shown in to be suitable. The arrangement of the small squares was random but remained the same for all participants to ensure comparability.
Procedure for Experiment 4: Strips of Dashes
To avoid mental bundling, we changed the arrangements. Instead of the clouds of squares, they now contained strips of dashes, more precisely: different numbers of lines in a row at regular intervals (see and ).
Figure 4. 28 arrangements displayed exactly 250 milliseconds on the computer screen in experiment 4 ‘strips of dashes’.
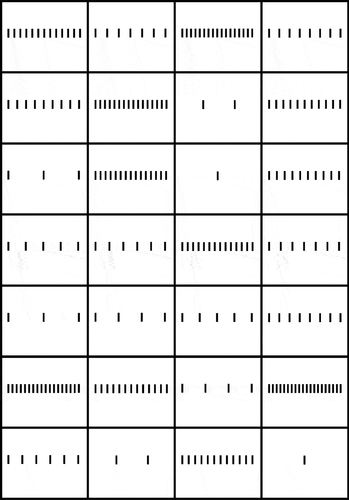
This should complicate the estimation of the numbers. The suggestive shape of the different strips, which differed only in the density of hatching, was similar in all arrangements.
Statistical Analysis
Assumptions about the distribution (e.g. normal distribution) of the frequency of correct number identifications or estimates cannot be made. Four tests are available for this case: the Mann-Whitney U test, the Kolmogorov-Smirnov test, the Wald Wolfowitz test and the Moses test. The first three methods can be used to test the hypothesis that the two samples are from the same population. Since we have no assumptions about the frequency distribution of correctly identified quantities and do not know the frequency of correct estimates, all four tests are helpful.
The Mann-Whitney U test is a nonparametric test of the null hypothesis that it is equally likely that a randomly selected value from one sample will be less than or greater than a randomly selected value from a second sample. The Kolmogorov-Smirnov statistic quantifies a distance between the empirical distribution functions of two samples. The Wald Wolfowitz test detects differences between distributions that differ solely in their location. The Moses test compares the span of the two samples and checks for two independent samples from a continuous field if extreme values are equally likely in both populations or extreme values are more likely to occur in the population from which the sample with the larger range was drawn.
Since the number of correct mentions of the number is based on an identical unit (correct or incorrect number), the scaling level is also given for the Kolmogorov-Smirnov test. By contrast, the remaining examination procedures only require ordinal scale level.
Differences between the mean frequencies of correct estimates of the single participant in the experimental and in the comparison group have a roughly normal distribution, because there are equal probabilities to estimate the number higher or lower. We used the independent samples t-test, because two separate sets of independent and identically distributed (computed with F-test) samples were obtained.
Results
Experiment 1: Dice Point Images
The differences between the number of right estimates of the items in the experimental and in the comparison group verify our hypothesis: More than 85 percent of the participants, both those of the comparison group and those with trisomy 21 in the experimental group, easily recognised the classic dice point images (one to six points). Uncertainties only occurred in a few participants of the experimental group, but the differences are not statistically significant (, ).
Figure 6. Relative frequencies of participants with three correct indications of the number of points in experiment 1 ‘dice point images’. Abscissa: number of points. Ordinate: percentage of participants with three correct indications of the number of points. (The differences are statistically highly significant, with an error probability of p < 0.001, Mann-Whitney test, Moses test, Kolmogorov-Smirnov test in two samples and Wald Wolfowitz test.).
Table 1. The differences between the number of correct estimates of participants in the experimental and in the comparison group in experiment 1 (dice point images)
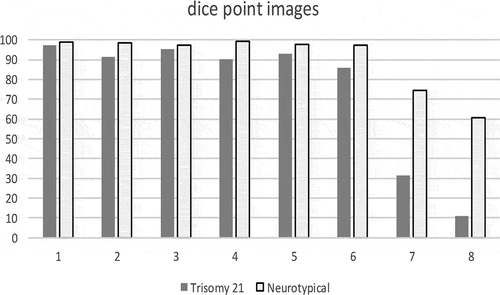
Only in the seven- and eight-point images in the style of the dice point images occurred significant failure frequencies. As expected from the simultandysgnosia hypothesis, highly significant differences between the experimental and comparison groups were found again ().
This confirms the hypothesis: The participants of the experimental group recognise the numbers on conventional dice images just as fast (in 250 milliseconds) as the participants of the comparison group. However, more problems than those of the comparison group occur significantly at higher numbers ordered according to a similar pattern.
Experiment 2: Interference Pictures
The differences between the number of right estimates of the items in the experimental and in the comparison group verify our hypothesis: The statistically highly significant differences between experimental and comparison group (, ) suggest that individuals with trisomy 21 who have a developed number concept actually have difficulty relating parts to the whole and the other way round.
Figure 7. Relative frequencies of participants with correct identification of numbers of points in experiment 2 ‘interference pictures’. Abscissa: number of points. Ordinate: percentage of participants with correct indications of the number of points. (The differences are statistically highly significant, with an error probability of p < 0.001, Mann-Whitney test, Moses test, Kolmogorov-Smirnov test in two samples and Wald Wolfowitz test.).
Table 2. The differences between the number of right estimates of participants in the experimental and in the comparison group in experiment 2 (interference pictures)
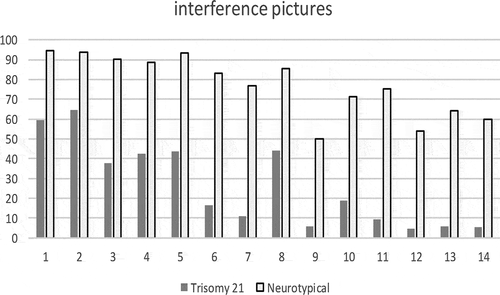
At this point, it can be seen that the hypothesis has been confirmed: Participants in the experimental group have significantly greater difficulty in simultaneously identifying the overall shape and details of the interference images than participants in the comparison group.
Experiment 3: Clouds of Squares
The differences between the number of right estimates of the items in the experimental and in the comparison group verify our hypothesis: In this third experiment, differences between the experimental and the comparison groups are apparent as expected in the case of simultandysgnosia in persons with trisomy 21 (): The frequency of correct estimates of the numbers decreases significantly earlier in the experimental group (participants with trisomy 21) with increasing number of squares shown than in the comparison group.
Figure 8. Relative frequencies of participants with correctly identified numbers in experiment 3 ‘clouds of squares’. Abscissa: number of elements of the disordered sets. Ordinate: percentage of participants with correct indications of the number of elements of the disordered quantity. (The differences are statistically highly significant, with an error probability of p < 0.001, Mann-Whitney test, Moses test, Kolmogorov-Smirnov test in two samples and Wald Wolfowitz test.).
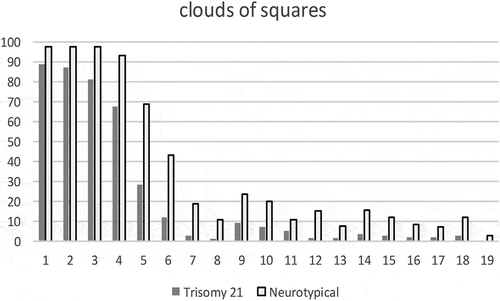
For up to six units, nearly half of the comparison group estimated the number correctly (see , ). These are, however, slightly more accurate estimates than would be expected for an attention scope of three to four units.
Table 3. The differences between the number of right estimates of participants in the experimental and in the comparison group in experiment 3 (clouds of squares)
The hypothesis has nevertheless been confirmed: First errors in the number of small squares displayed only 250 milliseconds occur in the participants of the experimental group even at smaller amounts than in the people of the comparison group.
Experiment 4: Strips of Dashes
The differences between the number of right estimates of the items in the experimental and in the comparison group verify our hypothesis: For all participants involved in the study, estimating the numbers in the fourth experiment proved to be somewhat more difficult than in the third. Both the individuals with trisomy 21 and those in the comparison group found less opportunity for mental bundling of the elements within the 250-millisecond presentation time. The suggestive shape of the different strips, which differed only in the density of hatching, was similar in all arrangements.
With this experiment, we were able to reproduce the theoretically expected subitising limit of about four units with sufficient accuracy. The frequencies of correct estimates of numbers in the experimental group (participants with trisomy 21) in this experiment decreased slightly after two units and very clearly after three units (see , ).
Figure 9. Relative frequencies of participants with correct indications of the number of dashes in experiment 4 ‘strips of dashes’. Abscissa: number of dashes. Ordinate: percentage of people with correct indications of the number of dashes within the row. (The differences are statistically highly significant, with an error probability of p < 0.001, Mann-Whitney test, Moses test, Kolmogorov-Smirnov test in two samples and Wald Wolfowitz test.).
Table 4. The differences between the number of right estimates of participants in the experimental and in the comparison group in experiment 4 (strips of dashes)
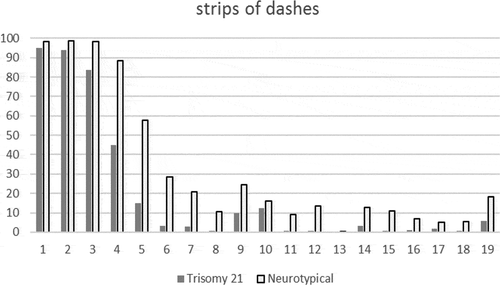
Potential Limitation of the Research
For the evaluation of the results, mainly the correct identifications of the amount were evaluated. This includes the number of correct answers based on randomly correct estimates too. The probability for estimating the right number decreases with increasing amount of elements. However, the small numbers are characterising the difference. To minimise this effect the sample size is important. Nevertheless, it is not so easy to find people with both trisomy 21 and a developed number concept.
In our studies, forty percent of the participants without trisomy 21 already had a fully developed concept of number in the age range of four to five years. The participants with trisomy 21 only reached a similar value in the age range of 14 to 17 years. While the entire group without trisomy 21 has fully developed concept of number from age of 14 years, the group with trisomy 21 reached a peak of 45 percent in the age group of 18+ years (Rieckmann, Citation2016, p. 160).
The experimental group consists of participants with trisomy 21, who are accompanied by very dedicated parents. Therefore, it is not a representative random sample. Many of the participants in the trial group stood out because they had trained their working memory very well, were very motivated and could handle test situations. Some enjoyed demonstrating their well-trained working memory. For example, a teenager with trisomy 21 asked for multiplication tasks over and over again to convince us of his ability to do mental arithmetic. This might be an indication that the use of working memory is a learned ability, but the subitising limit would seem to be an inherent, immutable characteristic.
In the comparison group, however, we assume a representative random sample, because a relationship with people with trisomy 21 should not have an effect on the subitising limit. Prerequisite for participation was that there was no Down syndrome – and no other syndrome in the spectrum of neurodiversity, such as autism, epilepsy, dyscalculia, etc.
Discussion
The good performance of people with trisomy 21 in the ‘dice point images’ experiment suggests, that conceptual subitising is easier for them than perceptual subitising. It is similar to persons with simultanagnosia (Huberle & Karnath, Citation2006, p. 234).
The experiment ‘interference pictures’ illustrates the difficulties of persons with trisomy 21 to change between overall perception and detailed perception (like persons with simultanagnosia).
That is very important for learning. In reading it is difficult for people with trisomy 21 to regard the whole word and the letters at the same time. In mathematics they have basic problems such as to recognise simultaneously more than two digits. With the training of their working memory they can improve their abilities a lot, but they have to work much harder than neurotypical people because of their smaller subitising limit. Teachers should respect that.
The experiments ‘clouds of squares’ and ‘strips of dashes’ confirm the hypothesis that the scope of attention of persons with trisomy 21 is limited to 2 to 3 units.
People with simultandysgnosia have a full field of vision. It is not their perception that is limited, but their attention. Since subitising by neurotypical people is according to Trick and Pylyshyn (Citation1994) a pre-attentional processing mechanism with strict capacity limitation on four chunks, we could speculate that the narrowed attentional scope causes learning difficulties in people with trisomy 21. That is why diagnostic and learning methods should consider this limit of the attention span.
Final Remarks
Bird and Buckley (Citation2000, p. 49) recommend the following: ‘Children with Down’s syndrome can be helped by incorporating additional options of motor and visual processing of information. Language, which is supported by symbolic movements such as signs, gestures or the finger alphabet and visual methods, such as images, symbols, words and formulas (multisensory methods), helps the children to remember information.’
As motivating as visual and multisensory offerings may be, they can also distract learners from the essential, especially when the subitising limit is lower. That is very important for abstract concepts in mathematics. If someone recognises triangles, that does not mean that the person has understood that a triangle represents the shortest connection between three geometrical points. Successfully adding minutes does not mean that the person has understood that one minute is longer than 25 seconds, etc.
We agree with Yang, Conners, and Merrill (Citation2014, p. 1473): ‘Although further research is needed for firm conclusions on some visuo-spatial abilities, there was no evidence that visuo-spatial ability is a strength in DS relative to general cognitive ability. Rather, the review suggests an uneven profile of visuo-spatial abilities in DS in which some abilities are commensurate with general cognitive ability level, and others are below.’
In various individuals with trisomy 21, the assumption that their cognitive strengths are found primarily in visual-spatial thinking may prove counterproductive and may even exacerbate learning disabilities.
This raises many new questions that we are currently investigating in further research projects: Are intelligence tests barrier-free for people with trisomy 21? Do learning methods in small steps consider the limited attention span of people with trisomy 21? Do ‘ten frames’ (bundling two times five elements) and ten-structures in arithmetic have the same effect for people with trisomy 21 as they do for neurotypical people?
With trisomy 21, learning arithmetic is particularly challenging. This could be due to the decimal system, which does not meet the specific attention attributes of people with trisomy 21. Since it’s hardly possible to replace the prevailing numerical system with another, it is up to parents and teachers to select appropriate learning materials to avoid aversions.
We are developing new materials together with people with trisomy 21. Their suitability in individual cases, can only be discovered by experiment. It is fundamentally recommended that as many different teaching materials are made available to students to choose from freely. Traditional lessons can be avoided by taking interests, talents and specific attention attributes of students with trisomy 21 into account.
Disclosure statement
No potential conflict of interest was reported by the authors.
References
- Abbeduto, L., Warren, S. F., & Conners, F. A. (2007). Language development in Down syndrome: From the prelinguistic period to the acquisition of literacy. Developmental Disabilities Research Reviews, 13(3), 247–261.
- Baddeley, A., & Jarrold, C. (2007). Working memory and Down syndrome. Journal of Intellectual Disability Research, 51(12), 925–993.
- Bellugi, U., & George, M. (2001). Journey from cognition to brain to gene. Perspectives from Williams Syndrome. Cambridge: MIT Press.
- Bird, G., & Buckley, S. (2000). Handbuch für Lehrer von Kindern mit Down-Syndrom [Manual for teachers of children with Down syndrome]. Eltersdorf: g&s.
- Campos, I. S., Almeida, L. S., Ferreira, A. I., Martinez, L. F., & Ramalho, G. (2013). Cognitive processes and math performance: A study with children at third grade of basic education. European Journal of Psychology of Education, 28(2), 421–436.
- Clements, D. H. (1999). Subitizing: What is it? Why teach it? Teaching Children Mathematics, 5, 400–405.
- Dalrymple, K., Birmingham, E., Bischof, W. F., Barton, J. J. S., & Kingstone, A. (2011). Experiencing simultanagnosia through windowed viewing of complex social scenes. Brain Research, 1367, 265–277.
- Dehaene, S. (1997). The number sense: How the mind creates mathematics. New York: Oxford University Press.
- Dehaene, S., & Cohen, L. (1994). Dissociable mechanisms of subitizing and counting: Neuropsychological evidence from simultanagnosic patients. Journal of Experimental Psychology: Human Perception and Performance, 20(5), 958–975.
- Geary, D. C. (2006). Development of mathematical understanding. In D. Kuhn, R. S. Siegler, W. Damon, & R. M. Lerner (Eds.), Handbook of child psychology: Volume 2: Cognition, perception, and language (pp. 777–810). Hoboken: Wiley.
- Hoffmann, D. (2003). Visuelle Intelligenz. Wie die Welt im Kopf entsteht [Visual intelligence. How the world is created in the mind]. München: dtv.
- Huberle, E., & Karnath, H.-O. (2006). Global shape recognition is modulated by the spatial distance of local elements – Evidence from simultanagnosia. Neuropsychologia, 44, 905–911.
- Lurija, A. R. (1970). Die höheren kortikalen Funktionen des Menschen und ihre Störungen bei örtlichen Hirnschädigungen [The higher cortical functions of humans and their disorders in local brain damage]. Berlin: DVW.
- Lurija, A. R. (1992). Das Gehirn in Aktion [The brain in action]. Reinbek: Rowohlt.
- Montoya, J. C., Fajardo, D., Peña, A., Sánchez, A., Domínguez, M. C., Satizábal, J. M., & García-Vallejo, F. (2014). Global differential expression of genes located in the Down Syndrome Critical Region in normal human brain. Colombia Médica, 45(4), 154–161.
- Navon, D. (1977). Forest before trees: The precedence of global features in visual perception. Cognitive Psychology, 9, 353–383.
- Navon, D. (1981). Do attention and decision follow perception: Comment on Miller. Journal of Experimental Psychology. Human Perception and Performance, 7, 1175–1182.
- Oelwein, P. L. (2007). Kinder mit Down-Syndrom lernen lesen [Children with Down syndrome are learning to read]. Eltersdorf: g&s.
- Piaget, J., & Szeminska, A. (1965). Die Entwicklung des Zahlbegriffes beim Kinde [The Child’s Conception of Number]. Stuttgart: Klett.
- Plaisier, M. A., Bergmann Tiest, W. M., & Kappers, A. M. L. (2009). One, two, three, many-subitizing in active touch. Acta Psychologica, 131(2), 163–170.
- Repp, B. H. (2007). Perceiving the numerosity of rapidly occurring auditory events in metrical and non-metrical contexts. Perception & Psychophysics, 69, 529–543.
- Rieckmann, T. (2016). Cognitive development and mathematics. In A. F. Zimpel (Ed.), Trisomy 21 – What we can learn from people with Down syndrome (pp. 158–174). Bristol, CN: Vandenhoeck & Ruprecht.
- Schneider, W. X., & Deubel, H. (2000). Characterizing chunks in visual short-term memory: Not more than one feature per dimension? Behavioral and Brain Sciences, 24(1), 144–145.
- Squire, L. R., & Kandel, E. R. (1999). Gedächtnis. Die Natur des Erinnerns [Memory. The nature of remembering]. Heidelberg: Spektrum Akademischer Verlag.
- Trick, L. M., & Pylyshyn, Z. W. (1994). Why are small and large numbers enumerated differently? A limited-capacity preattentive stage in vision. Psychological Review, 101(1), 80–102.
- Vicari, S., Bellucci, S., & Carlesimo, G. A. (2006). Evidence from two genetic syndromes for the independence of spatial and visual working memory. Developmental Medicine & Child Neurology, 48(2), 126–131.
- Vicari, S., Marotta, L., & Carlesimo, G. A. (2004). Verbal short-term memory in Down’s syndrome: An articulatory loop deficit? Journal of Intellectual Disability Research, 48, 80–92.
- Wilken, E. (2014). Sprachförderung bei Kindern mit Down-Syndrom [Language support in children with Down syndrome]. Stuttgart: Kohlhammer.
- Witecy, B., & Penke, M. (2016). Das Verhältnis von Sprache und Kognition bei deutschsprachigen Kindern und Jugendlichen mit Down-Syndrom. [The relationship between language and cognition in German-speaking children and adolescents with Down syndrome]. Sprache, Stimme, Gehör, 40(2), 93–99.
- Witecy, B., & Penke, M. (2017). Language comprehension in children, adolescents, and adults with Down syndrome. Research in Developmental Disabilities, 62, 184–196.
- Wundt, W. (1901). Grundriss der Psychologie [Outline of psychology]. Leipzig: Engelmann.
- Wundt, W. (1913). Grundriss der Psychologie [Outline of psychology]. Leipzig: Kröner.
- Yang, Y., Conners, F. A., & Merrill, E. C. (2014). Visuo-spatial ability in individuals with Down syndrome: Is it really a strength? Research in Developmental Disabilities, 35(7), 1473–1500.
- Zimpel, A. F. (2008). Der zählende Mensch. Was Emotionen mit Mathematik zu tun haben [The counting human. What do emotions have to do with math?]. Göttingen: Vandenhoeck & Ruprecht.
- Zimpel, A. F. (2013). Studien zur verbesserung des verständnisses von lernschwierigkeiten bei trisomie 21 – bericht über die ergebnisse einer voruntersuchung [studies to improve understanding of learning difficulties in trisomy 21 – report of the results of a preliminary test]. Zeitschrift für Neuropsychologie, 29(1), 35–47.
- Zimpel, A. F. (2016). Trisomy 21 – what we can learn from people with down syndrome. Bristol, CN: Vandenhoeck & Ruprecht.
- Zimpel, A. F, & Röhm, A. C. (2018). A study of imitation ability in people with trisomy 21. Zeitschrift Für Neuropsychologie, 29(4), 223–235.