Abstract
We investigated the spatial distributions of total phosphorus (P) and its fractions in the sediments from Hongfeng Reservoir, the largest man-made water body in southwest China, to reveal P sources to the reservoir and its pollution history. We sectioned sediment cores and measured P species concentrations, which increased progressively from relatively low concentrations at a depth of 20–30 cm to higher concentrations in upper sediment layers, reflecting an increase in P input that accompanied anthropogenic influences. P bound to metal oxides (NaOH-SRP) was the largest component of P in most core profiles, accounting for 34.6–67.2% of total P. The Yangchang River is the primary external P source to the reservoir and should be the focus of remediation efforts in the future. A batch P release experiment conducted using sediment cores showed that sediment P release was mainly caused by the transformation and/or desorption of NaOH–P and Residual–P under high temperature and low oxygen conditions. Large P release from sediments could affect the water quality of the reservoir in the future. Detailed knowledge of in situ microscale changes at the sediment–water interface is required to understand the migration of P fractions in the sediments.
Phosphorus (P) is a major factor contributing to lake eutrophication (James et al. Citation2000, Conley et al. Citation2009). P sources include rainfall, runoff, soil leaching, industrial and municipal waste water discharge, and internal release from sediments (Carpenter et al. Citation1998). Although lake sediments act as a long-term sink of P, nutrient release from sediments (internal loading) can be a significant term in the annual P budget of many lakes, especially after a reduction of external loading (Søndergaard et al. Citation2003, Haggard et al. Citation2005). High rates of P release from sediments into the overlying water may prevent improvement of water quality and delay lake recovery for a considerable period after external loading is reduced (Carpenter Citation2005, Genkai-Kato and Carpenter Citation2005, Nausch et al. Citation2009).
Previous studies showed that the major physicochemical conditions that affect sediment P release at the sediment–water interface (SWI) are temperature, dissolved oxygen (DO) concentration, and pH (Jin et al. Citation2006, Jiang et al. Citation2008, Steinberg Citation2011). High temperatures and anaerobic conditions promote release of P from sediments. Higher temperatures (e.g., >20 C) enhance the growth of bacteria and autotrophic algae and lead to greater release of P from sediment (Jiang et al. Citation2008). The effects of DO on P release are influenced by redox and pH (Wang et al. Citation2008). Anoxia at the SWI cause reduction of iron (Fe(III))-host minerals, which results in Fe-bound P release, commonly regarded as a major P-release mechanism (Steinberg Citation2011). Release of Fe–P occurs frequently under alkaline conditions, while acid pH promotes release of calcium (Ca)-bound P. Less P release occurs under neutral conditions (Jin et al. Citation2006). These studies demonstrated that P release from sediments to the water column is achieved by transformation of P fractions in sediments, which is controlled by the physicochemical conditions at the SWI and mineralization of organic P in the sediments.
Sediment P release depends mainly on the total P concentration and the relative abundances of P fractions (Gächter and Müller Citation2003); thus, total P concentration and its fractions have been widely investigated to understand P dynamics and estimate the amount of mobile P (Nürnberg Citation1988, Zheng et al. Citation2004, Gao et al. Citation2005, Rydin et al. Citation2011). Sequential chemical extraction techniques have been used to characterize P fractions in sediments (Psenner et al. Citation1988, Ruttenberg Citation1992, Hupfer et al. Citation1995). These P fractions include NH4Cl–P, BD–P, NaOH-SRP, NaOH-NRP, HCl–P, and Residual–P. The NH4C1–P fraction represents the loosely adsorbed P, which may include porewater P, P released from CaCO3 associated P, or P leached from decaying bacterial cells in deposited phytodetrital aggregates. BD–P represents redox-sensitive P forms primarily bound to Fe-hydroxides and manganese (Mn) compounds. NaOH-SRP represents P bound to metal oxides, primarily of Fe and aluminium (Al), and is exchangeable with OH− and inorganic P compounds soluble in bases. NaOH-NRP> primarily represents polyphosphate (poly-P) in NaOH and/or ethylenediaminetetraacetic acid (EDTA) extracts of the sediments. HCl–P represents P forms sensitive to low pH and is assumed to consist mainly of apatite P and P bound to carbonates. Residual–P represents refractory organic P. The distributions of P fractions in sediment cores have been used to estimate potential release of P from sediments (Mort et al. Citation2010, Rydin et al. Citation2011).
A sound understanding of both external P sources and the mechanism of internal P release from sediments is necessary to formulate lake restoration plans. Most external P is ultimately transferred to the sediments; thus, the total P concentration and its fractions in sediments provide information for tracking sources of external P. Concurrent reservoir hydrology and historical monitoring data are needed to support the conclusions based on total P and its fractionations.
Hongfeng Reservoir (106.24°N, 26.30°E) is one of the largest man-made water bodies on the Yunnan-Guizhou Plateau in southwest China (). It supplies drinking water to the provincial capital, Guiyang City, with a population greater than 3 million people. Hongfeng Reservoir was constructed in the 1960s and has a surface area of 57.2 km2, a mean water depth of 10.5 m (Zmax = 45 m), and holds 6.01 × 108 m3 of water. Some small islands are distributed in the reservoir, especially in the south lake area. Over the last few decades the catchment has undergone rapid industrialization, and P pollution has become a major problem for water quality in the reservoir (Chen et al. Citation2010). Annual external P loading is at least 50 tonnes/yr. A chemical fertilizer plant lies upstream of Yangchang River (Xia and Li Citation2011). A large amount of phospho-gypsum residue generated during the manufacture of phosphamidon, an insecticide, has accumulated in ponds only 800 m from the Yangchang River. Because there are no wastewater treatment facilities, phospho-gypsum leachate is discharged directly into the Yangchang River through surface runoff and eventually makes its way into the reservoir. The total P concentration in these surface runoff waters exceeds 0.5 mg/L. Since 2007, the local government has transported the waste tailings out of the basin, reducing the P contribution to the reservoir. Cage fish culturing began in Hongfeng Reservoir in 1987 and was stopped in 1999. Originally confined to the shore line, it expanded until the large-scale fixed and floating cages ultimately covered 0.3–0.5% of the reservoir. Residual fish food (0.12 million tonnes/yr), and fish excrement brought considerable quantities of P to the sediments. Hongfeng Reservoir is monomictic and strongly stratified in summer. Soluble P, mobilized after the onset of anoxia, accumulates rapidly in the hypolimnion, reaching concentrations >0.20 mg/L in summer. Isothermy occurs in the reservoir by early autumn nearly every year, leading to water-column circulation, sediment P release, anoxia in surface waters, and fish deaths (Wan et al. Citation2010).
We investigated the spatial distribution of total P and its fractions in sediments as well as the mechanism of P release from sediments. Our objectives were to (1) identify sources of external P loading and the pollution history of the reservoir, and (2) study the process of P release from sediments. Our results can be used to guide future plans for P-control in the reservoir.
Materials and methods
Sampling and analytical procedures
To investigate the spatial distribution of P and its fractions in sediments of Hongfeng Reservoir, we collected 107 sediment cores in September 2008 and 4 sediment cores in July 2010 (). A plastic static gravity corer with a 6 cm internal diameter was used to extract the cores. Cores were used for study only if they had an undisturbed sediment surface and overlying water. Lengths of cores collected in 2008 ranged from 4 to 78 cm, with an average value of 31 cm. The 2008 cores were sectioned into 5 cm segments in the upper 10 cm, and into 10 cm segments from 10 cm depth to the core base. Sediment cores collected in 2010 were taken at the Taohuanyuan River (water depth ∼10 m), the Yangchang River (water depth ∼12 m), the fish-farming region (water depth ∼8 m), and near the outlet (water depth ∼40 m), and sectioned into 2 cm segments. All samples were immediately transferred to the laboratory in coolers (<4 C) and freeze-dried. Dry samples were ground and sieved for chemical analysis with a standard 100-mesh sieve.
P release from sediments
Additional sediment cores were collected, one from the outlet area in November 2014 and one from the south central lake region in April 2015, to study P release from sediments. Cores were sampled using a polymethyl methacrylate glass tube covered with tinfoil and kept in the dark while being transferred to the laboratory. Experiments were conducted in a transparent rectangular Perspex box (20 × 20 × 50 cm, width × length × height) filled with filtered lake water. Each core was put in the water and placed, along with the Perspex box, into an illumination incubator (SPX-70BIII, Taisite Instrument Co., Tianjing, China). The incubation temperature was set at 20 C, equivalent to the temperature of bottom water in the reservoir during summer. The batch experiment lasted for 14 days. Because the hypolimnion in the outlet area of Hongfeng Reservoir is anaerobic, the experiment was conducted under low-oxygen conditions (DO < 1.0 mg/L, Eh ∼ −50 mV, pH ∼7.6), produced by purging the overlying water with nitrogen (99%) for 2 h twice every day.
To determine how the chemical fractions of P in sediments change with the P release process, dissolved reactive P (DRP) and P fractionations in surface sediments (0–5 cm) were analyzed before and after the incubation experiment. Sediments were sectioned into 1 cm intervals. Porewater subsamples (n = 5) were collected using centrifugation (3500 r/min, 20 min), after which sediment subsamples (n = 5) were immediately freeze-dried. Dry samples were ground and sieved for analysis of P fractions with a standard 100 mesh sieve (Ruban et al. Citation2001).
Analysis of P fractions in sediments
Sediment P fractionation was performed using the sequential extraction scheme of Psenner et al. (Citation1988) and Hupfer et al. (Citation1995). Determination of the P fractions was as follows: (1) NH4C1–P, extracted with 1 M NH4C1 solution for 0.5 h; (2) BD–P, extracted with 0.11 M bicarbonate/dithionite solution for 1 h; (3) NaOH–P, total P extracted with 1 M NaOH solution for 16 h; composed of NaOH-SRP and NaOH-NRP; (4) HC1–P, extracted with 0.5 M HCl solution for 16 h; and (5) Residual P, digested with 30% (v/v) H2SO4 and 8% K2S2O4 at 121 C for 30 min, extracted with 1 M HCl solution for 16 h. Concentrations of P in the extraction solution were determined using the method of Murphy and Riley (Citation1962).
Mapping and calculations
We used the inverse distance weighted (IDW) method to interpret the data and map the spatial distribution of total P in the sediments, employing ArcGIS software (v9.3). IDW is commonly applied to interpolate hydrological and soil properties (Ahmed and De Marsily Citation1987, Ashraf and Afshari Citation2013).
Concentrations of P fractions in the sediments collected from sites near the Yangchang River and reservoir outlet were correlated by regression analysis using SPSS software. The Pearson correlation coefficient was used to determine the level of those correlations.
Results
Total P concentration in the sediments
Total P concentrations varied with sediment depth in the Hongfeng Reservoir (). Total P concentrations in surface sediments (0–5 cm) ranged from 766 to 4306 mg/kg, with a mean value of 1815 mg/kg. Of the samples in this layer, 93% had >1000 mg/kg of P and 31% had >2000 mg/kg of P. Total P concentrations decreased with increasing sediment depth. In the 5–10 cm layers, the total P concentrations varied from 443 to 5160 mg/kg, with a mean value of 1696 mg/kg. For the 10–20 cm and the 20–30 cm layers, the average total P concentrations were 1566 and 1162 mg/kg, respectively.
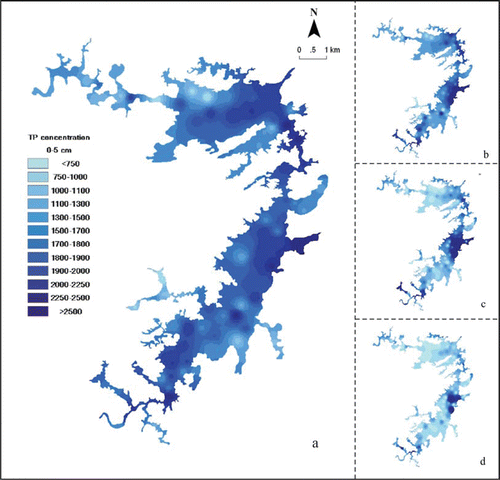
Total P concentrations in the sediment profiles were different at the 4 sites (). At Taohuanyuan River, the average total P concentration was 938 mg/kg, which was relatively constant with sediment depth. At Yangchang River, the total P concentrations decreased rapidly with depth in the 0–20 cm sediment layers (from 1579 to 978 mg/kg), then varied slightly in the 20–40 cm layers (from 889 to 792 mg/kg). The change in total P concentrations in the core profile from the outlet site was similar to that from the Yangchang River. In the fish farming region, total P concentrations increased with sediment depth from the surface (1491 mg/kg) to 10 cm (2300 mg/kg), changed slightly between 10 and 20 cm, then decreased dramatically (from 1921 to 320 mg/kg) in deeper layers.
P fractions in the sediments
The P fractions consisted primarily of inorganic P species, including NH4Cl–P, BD–P, NaOH–srP, and HCl–P, but included organic P as well (). Generally, concentrations of the various P fractions at the 4 sites followed the order of NaOH-SRP > Residual–P > NaOH-NRP > HCl–P > BD–P > NH4Cl–P in the entire profile.
The NH4Cl–P concentrations in the sediments ranged from 7 to 54 mg/kg at the 4 sites, accounting for <2% of the total P (). At the Taohuanyuan River, NH4Cl–P concentrations were typically constant with sediment depth and averaged 7 mg/kg for the entire sediment core. At the other sites, NH4Cl–P concentrations decreased rapidly in the 0–20 cm layers but changed only slightly in the 20–40 cm layers. The highest NH4Cl–P concentration (54 mg/kg) was measured in sediments from the fish-farming region.
The BD–P concentration was lowest at the Taohuanyuan River, with a mean value of 16 mg/kg for the 0–30 cm section, equivalent to 1.7% of total P (). The BD–P concentration changed only slightly throughout the sediment profile. At the Yangchang River and outlet sites, BD–P was relatively high and declined rapidly from 120 mg/kg at 0–2 cm to 40 mg/kg at 18–20 cm depth but changed only slightly in deeper strata. In the fish-farming region, the BD–P concentration was relatively high, and the concentration increased with sediment depth in the 0–10 cm layers from 86 to 155 mg/kg and then declined rapidly in the 10–20 cm layers from 155 to 39 mg/kg.
The NaOH-SRP concentration represented the greatest proportion in most of the core profiles, accounting for 34.6–67.2% of total P (). Mean NaOH-SRP concentrations in the cores from the 4 sites were 835 mg/kg in the fish-farming region, 713 mg/kg in the Yangchang River, 579 mg/kg in the outlet site, and 381 mg/kg in the Taohuanyuan River. NaOH-srP concentrations varied slightly throughout the sediment profile from 335 to 380 mg/kg. At the outlet and Yangchang River, NaOH-SRP increased with depth in the 0–5 cm layers and then declined rapidly from 1259 mg/kg at 5 cm depth to 455 mg/kg at 20 cm depth. In the fish-farming region, the NaOH-SRP concentrations first increased with depth in the 0–10 cm layers from 763 to 1464 mg/kg, then declined rapidly to 331 mg/kg at 25 cm depth.
NaOH-NRP concentration was highest at the outlet site, with a mean value of 168 mg/kg for the 0–30 cm section, or 5.3% of the total P (). The concentration declined with sediment depth, from 209 mg/kg in the surface 2 cm to 89 mg/kg in the bottom layer. Concentration profiles of NaOH-NRP in the fish-farming region remained stable in the 0–20 cm layers with a mean of 190 mg/kg, and declined slowly to 95 mg/kg at 40 cm depth. At Taohuanyuan River and Yangchang River, the NaOH-NRP concentrations were relatively constant with depth and displayed average values of 111 and 108 mg/kg, respectively.
HCl–P comprised 5.5–24.6% of the total P in the 0–30 cm section, with the highest concentration measured at the outlet site (). At outlet and Yangchang River, HCl–P concentration increased with sediment depth in the 0–5 cm layers from 190 to 210 mg/kg, changed only slightly in the 5–15 cm layers, and declined rapidly between 15 and 20 cm depth from 213 to 97 mg/kg. The HCl–P concentrations at Taohuanyuan River changed little over the entire profile. At the fish-farming region, the concentration profile of HCl–P was similar with those of NH4Cl–P and BD–P.
Residual–P concentrations were high, >300 mg/kg in the top 10 cm of the 4 core profiles, and the proportion of the total P represented by Residual–P ranged between 12.9% and 39.2% (). The highest average Residual–P concentration for the 0–30 cm section (358 mg/kg) was measured at the Taohuanyuan River site. At most sites, Residual–P concentrations varied only slightly with depth in the sediment, whereas in the fish-farming region, Residual–P concentrations decreased rapidly with depth in the 20–30 cm layers from 290 to 68 mg/kg.
Correlation between P fraction concentrations from different sampling sites
Vertical P fractions in the sediments from the outlet area exhibit significant positive correlations with the P fractions in sediments from the Yangchang River (P < 0.01, ). In all fractions, Residual–P in sediments from these 2 samplings sites has the lowest correlation coefficient (r = 0.708). Except the Residual–P, all other sediment P fractions had a correlation coefficient >0.900. BD–P in sediments from the 2 sites has the highest correlation coefficient (r = 0.954).
Temporal variation of total P concentrations in the inflow waters
Monthly monitoring data of total P concentrations in the water of inflow rivers to Hongfeng Reservoir were recorded from January 2009 to December 2013 (). Before July 2011, the P concentration in the Yangchang River averaged ∼0.50 mg/L, about 10 times greater than P concentration in the Taohuayuan River water. Although the P concentrations in the Yangchang River decreased markedly after July 2011, it was still higher than that in the Taohuayuan River, indicating that the Yangchang River has been the most important P source for the Hongfeng Reservoir over the last few decades and has controlled P concentration in waters and sediments. Assuming an average annual inflow rate of 12.67 m3/s (Wang et al. Citation2012) and water P concentration of ∼0.05 mg/L from July 2011 to present, we estimate that the Yangchang River generally carries ∼15 tonnes/yr of P into the reservoir, accounting for ∼75% of the total P amount (∼20 tonnes) in the water of Hongfeng Reservoir.
DRP and P fraction changes in the experiment
P fraction concentrations in the surface sediments (0–5 cm) changed significantly at the end of the batch experiment (). At the beginning, total P concentration in surface sediments averaged 1789 ± 175 mg/kg at the outlet and 1907 ± 215 mg/kg in the south central lake region. After the 14 d incubation period, the mean concentration decreased to 1338 ± 154 mg/kg at the outlet and 1592 ± 217 mg/kg in the south central lake region. At the outlet site, NaOH–P and Residual–P concentrations in sediments declined on average by 385 and 59 mg/kg, respectively. P fraction concentrations in sediments of the south central lake region exhibited similar variation characteristics, with reductions of NaOH–P and Residual–P concentrations by an average of 113 and 168 mg/kg, respectively. These declines account for most of the reduction in total P concentrations. Mean concentrations of NH4Cl–P, BD–P, and HCl–P did not change substantially during the experiment. Throughout the experiment, DRP concentrations in the overlying water increased from 0.050 to 0.112 mg/L at the outlet and from 0.023 to 0.134 mg/L in the south central lake region; while the mean concentration of DRP in porewaters increased from 0.585 to 0.998 mg/L at the outlet and from 0.544 to 0.690 mg/L in the south central lake region.
Discussion
Pollution history of Hongfeng Reservoir
In the Hongfeng Reservoir sediments, the total P concentrations increased progressively from relatively low concentrations at a depth of 20–30 cm to higher values in upper layers (), most likely reflecting an increase in P input with increasing anthropogenic effects. Rapid development of industry and agriculture, along with population growth and urbanization in the catchment, resulted in large P inputs to the reservoir. As P loading increased, so did P accumulation and concentration in the sediments (Hupfer et al. Citation1995, Gao et al. Citation2005). Most regions of the Hongfeng Reservoir show no evidence of P pollution during the time period representing the depths of 20–30 cm (). At that time, the average concentration of total P in the sediments was only 1162 mg/kg, similar to P concentrations in local soils (Jiang et al. Citation2011).
Higher concentrations of total P, however, were already observed in the fish-farming and Yangchang River areas, possibly because deposition rates in these 2 regions were greater than the average value for the entire reservoir due to high density of fish food and feces from the fish-farming region and input of P-rich waste residues in the Yangchang River area. Rapid sedimentation at these sites suggests that deposition of sediment in the 20–30 cm layer occurred later (more recently) than in other regions of the lake. A large external P load was delivered to the reservoir during the time when the 5–20 cm sediment layer was deposited (, ). Sediments accumulated in those decades have average total P concentrations of 1566–1696 mg/kg. Highest total P concentrations occurred in uppermost 10 cm and have an average of 1815 mg/kg, suggesting that external P loading increased over time.
The effect of control measures for external pollutants is reflected in the concentration changes of pollutants in recent sediments; concentrations of total P and its fractions in the sediments from the outlet, Yangchang River, and the fish-farming areas decreased dramatically in the 0–10 cm layers (). Sediment P concentrations tended to increase toward the surface, but concentration gradients were notably larger in the top 20 cm layers across the entire reservoir, indicating that P inputs have varied substantially through time.
Sources of P in the sediments
Profile distribution of P fractions in the sediments from the north basin of the reservoir indicates no significant P loading from the Taohuanyuan River site, the only water inlet for the north basin. The increased total P concentration measured in the outlet region has been primarily controlled by inputs to the south basin. Yangchang River is the primary inflow to the south basin and likely dominated external P loading to the reservoir. Significant positive correlations with the P fractions in sediments from the Yangchang River and outlet site () suggest that they had common origins for incoming P and transformation mechanisms of the P species (Ting and Appan Citation1996, Jiang et al. Citation2011).
Similar patterns of P concentration with sediment depth across sites could be the result of multiple factors, including similarities among the sampling sites. In this study, water depth at the Yangchang River site was approximately 12 m, whereas the water depth at the outlet area was approximately 40 m. The different water depths at these 2 sites suggest they have different sediment depositional environments. Secondly, P in waters at the Yangchang River site was reactive because its primary P source was principally inorganic P (dissolved or particulate) from phospho-gypsum leachate. Such P can be transported over long distances before being used biologically and sedimented. In the outlet region, water velocity is reduced by the dam, thereby promoting deposition of the particulate phosphorus. All the above processes cause synchronous changes in P concentration and fractions in sediments of the Yangchang Rriver and in the downstream deposits. For example, P loading at the Yangchang River site was reduced in the 2000s, and sediment P in the outlet area also decreased. Moreover, monthly monitoring data show that phosphate concentration in Yangchang River water was higher than that in Taohuayuan River (Xia and Li Citation2011; ), revealing that the Yangchang River was the primary source of external P-loading.
Concentrations of most P fractions in the fish-farming region were highest in the 4 sampling sites of the reservoir. NaOH-SRP in the 0–10 cm layers ranged from 763 to 1464 mg/kg, with a mean value of 1064 mg/kg. One feature of P pollution caused by fish farming is the rapid P enrichment in the sediments over a short period, with most P present in the form of NaOH-SRP. With the cessation of fish culture activity, sediment P deposition was reduced. Downstream sites such as the outlet area were rarely influenced by the high P concentration, indicating that the P pollution caused by cage fish culture had mostly a local impact, probably a consequence of the following factors: (1) sediment P in the fish-farming zone here has low mobility because most P in the sediments was from undecomposed artificial fish food, given its short period of time in the sediments; and (2) hydrodynamic transfer of P in this region was reduced as a result of the physical barrier created by extensive islands in the vicinity.
Previous studies generally accepted that P loading in the fish-farming region would have a large impact on water-column P concentration across the whole reservoir because this region contained the highest sediment P concentration and was located in the middle of the entire reservoir (Chen et al. Citation2010, Jiang et al. Citation2011); consequently, P from the Yangchang River was not studied in detail. Our results suggest that the Yangchang River is key to the effective management of eutrophication in the Hongfeng Reservoir. Moreover, until now P pollution in the residue field of the chemical company has not been cleaned completely, and the residue will continue to release pollutants; therefore, the assumption that P pollution is currently under control seems to be incorrect. Further steps may be required to restore conditions in the Yangchang River, and additional investigation of how P is transferred from the residues to the lake water is needed and may serve as a basis for development of a P-treatment plan.
Release of P from the sediments
In the batch experiment, concentrations of DRP in the overlying water and in sediment porewaters increased as the sediments were cultured under summer conditions of high temperature (∼20 C) and low oxygen (DO < 1.0 mg/L; ). Mean concentrations of total P in the surface sediments decreased approximately 25.2% at the outlet and 16.5% in the south central lake region. These results indicate that P release from sediments under summer conditions can strongly affect water quality in Hongfeng Reservoir.
Previous studies suggested that recycling of P from sediments depends on changes in the P fractions in surface sediments, which may result from continuous transport of chemical species across the SWI (Jorcin and Nogueira Citation2005, Jin et al. Citation2006, Wang et al. Citation2006, Tang et al. Citation2013). At the outlet site, the largest component of P decrease in the sediments was for NaOH–P. A decline of 384.7 mg/kg on average accounted for 85.3% of the total P decrease. At the south-central lake region, 2 large components of P decrease in the sediments were for Residual–P and NaOH–P. A decline of 281.4 mg/kg on average accounted for 89.5% of the total P decrease; thus, the increase in DRP in porewaters and overlying waters in the Hongfeng Reservoir was mainly from the transformation or desorption of NaOH‒P and/or Residual–P under high temperature and low oxygen conditions.
NaOH–P in sediments is a potentially important P source for overlying water and is sensitive to DO and oxidation–reduction potential (ORP; Wilson et al. Citation2008). It has been widely accepted that anoxia produces low ORP levels that induce the reduction of Fe(III) to Fe(II), resulting in NaOH–P release and an increase in DRP concentration in porewater (Gomez et al. Citation1999). This classical P-release model has been used to explain seasonal changes in water column P in lakes (Steinberg Citation2011). Residual–P represents refractory organic P (Hupfer et al. Citation1995). Zhang et al. (Citation2009) characterized the organic P in sediments from Hongfeng Reservoir by solution 31P nuclear magnetic resonance (31P NMR) spectroscopy, showing that it was dominated by Monoester–P (14.3% of the NaOH-EDTA extracted P) and Diester–P (3.1%), with smaller amounts of phosphonate (0.1%). The abundance of the organic P pool mainly depended on the content of organic matter in sediments (Sun et al. Citation2013). Thus, the release of organic P in sediments was likely controlled by the degradation of organic matters, strengthened by the anoxia and high-temperature environmental conditions.
In our experiment, DO concentration was maintained at a low level (<1.0 mg/L) and the BD–P (redox-sensitive P) concentration did not change, indicating that the SWI was in a stable reducing environment. Apart from maintaining a constant temperature of 20 C, environmental conditions were consistent with those at the bottom of Hongfeng Reservoir. Experimental results showed NaOH–P and Residual–P were rapidly transformed and released into the porewaters, illustrating that the higher temperature promoted P release from the sediments under persistent low oxygen conditions, possibly in part a consequence of greater microbial activity (e.g., respiration and sulfur reduction; Gächter and Meyer 1993, Hupfer et al. Citation1995). More detailed knowledge of the in situ microscale changes of the SWI is required to understand the migration of P fractions in the sediments.
Future research
In this study, the objective of the preliminary batch experiment was to reveal the potential of P release from sediments in the Hongfeng Reservoir. More experiments should be conducted to advance scientific and accurate understanding of the release fluxes of P from sediments in this reservoir. First, at least 2–3 cores sampled at the beginning of the experiment should be used to provide a time-zero baseline. Second, multiple cores (3–5 cores) should be taken from each location to conduct the experiment, and only one sediment sample from each core should be analyzed to improve understanding of the variability within the sediment. Moreover, it would be useful to evaluate this experiment in different seasons (summer and winter) and under varied environmental conditions (aerobic and anaerobic).
Conclusions
Total P concentrations in the sediments of Hongfeng Reservoir increased progressively from relatively low concentrations at a depth of 20–30 cm to higher concentrations in the upper layers, reflecting an increase in P inputs with increasing anthropogenic impact. Among the P fractions in the sediments, the NaOH-SRP concentration was highest in most core profiles, accounting for 34.6–67.2% of the total P. The total P concentration and its fractions in sediments in from Yangchang River and fish-farming areas decreased dramatically upcore, in the 0–10 cm layers, showing the effect of control measures implemented to control external P loading.
Our results show that the Yangchang River has been the largest source of P loading to the Hongfeng Reservoir over the last few decades (, ). It is widely accepted that P loading in the fish-farming region had a great impact on water-column P concentration in the whole reservoir; thus, P from the Yangchang River was largely ignored. Our results, however, suggest that the Yangchang River is key to the effective management of external pollutants in this reservoir.
Under the simulation conditions of 20 C and DO < 1.0 mg/L, concentrations of DRP in the overlying water and sediment porewaters increased while total P concentrations in the sediments declined by ∼20%. The decreases of NaOH–P and Residual–P accounted for >85% of the total P decrease, suggesting that the increasing DRP in the overlying and porewaters was largely from the transformation and/or desorption of NaOH-P and Residual–P under high temperature and low oxygen conditions. Large P release from sediments will likely have a strong impact on future water quality of the reservoir. Further research is being conducted to investigate possible treatment measures to limit sediment P release, including dredging, in situ chemical treatment with aluminum or iron salts, injection of nitrate, and artificial destratification to increase oxygen levels in the hypolimnion.
Funding
This research was funded by grants from the Natural Science Foundation of China (No.41173125 and No. 41403113).
References
- Ahmed S, De Marsily G. 1987. Comparison of geostatistical methods for estimating transmissivity using data on transmissivity and specific capacity. Water Resour Res. 23(9):1717–1737.
- Ashraf S, Afshari H. 2013. Assessment of groundwater chemical quality, using inverse distance weighted method. J Chem Health Risks. 3(4):55–62.
- Carpenter SR. 2005. Eutrophication of aquatic ecosystems: bistability and soil P. PNAS. 102:10002–10005.
- Carpenter SR, Caraco NF, Correll DL, Howarth RW, Sharpley AN, Smith VH. 1998. Nonpoint pollution of surface waters with phosphorus and nitrogen. Ecol Appl. 8:559–568.
- Chen JA, Zhang W, Zhang RY, Li J, Zeng Y, Wang JF. 2010. Time and spatial distribution characteristics of nitrogen and P in the sediment of Lake Hongfeng. Proceedings of 13th World Lake Conference. China Agricultural University press. p. 2226–2230.
- Conley DJ, Paerl HW, Howarth RW, Boesch DF, Seitzinger SP, Havens KE, Lancelot C, Likens GE. 2009. Controlling eutrophication: nitrogen and phosphorus. Science. 323:1014–1015.
- Gächter R, Meyer JS. 1993. The role of microorganisms in mobilization and fixation of P in sediments. Hydrobiologia. 253:103–121.
- Gächter R, Müller B. 2003. Why the P retention of lakes does not necessarily depend on the oxygen supply to their sediment surface. Limnol Oceanogr. 48:929–933.
- Gao L, Zhou JM, Yang H, Chen J. 2005. P fractions in sediment profiles and their potiential contributions to eutrophication in Dianchi Lake. Environ Geol. 48:835–844.
- Genkai-Kato M, Carpenter SR. 2005 Eutrophication due to P recycling in relation to lake morphometry, temperature, and macrophytes. Ecology 86:210–219.
- Gomez E, Durillon C, Rofes G, Picot B. 1999. Phosphate adsorption and release from sediments of brackish lagoons: pH, O2 and loading influence. Water Res. 33:2437–2447.
- Haggard BE, Moore PA Jr, DeLaune PB. 2005. P flux from bottom sediments in Lake Eucha, Oklahoma. J Environ Qual. 34:724–728.
- Hupfer M, Gächter R, Ruegger A. 1995. Poly-P in lake sediments. 31P-NMR spectroscopy as a tool for its identification. Limnol Oceanogr. 40:610–617.
- James WF, Barko JW, Eakin HL, Helsel DR. 2000. Distribution of sediment phosphorus pools and fluxes in relation to alum treatment. J Am Water Resour Assoc. 36:647–656.
- Jiang X, Jin XC, Yao Y, Li LH, Wu FC. 2008. Effects of biological activity, light, temperature and oxygen on phosphorus release processes at the sediment and water interface of Taihu Lake, China. Water Res. 42(8–9):2251–2259.
- Jiang CH, Hu JW, Huang XF, Li CX, Deng JJ, Zhang J, Liu F. 2011. Phosphorus speciation in sediments of Lake Hongfeng, China. Chin J Oceanol Limnol. 29:53–62.
- Jin XC, Wang SR, Pang Y, Wu FC. 2006. Phosphorus fractions and the effect of pH on the phosphorus release of the sediments from different trophic areas in Taihu Lake, China. Environ Pollut. 139:288–295.
- Jorcin A, Nogueira MG. 2005. Temporal and spatial patterns based on sediment and sediment–water interface characteristics along a cascade of reservoirs (Parana-panema River, south-east Brazil), Lakes Reserv Res Manage. 10:1–12.
- Mort HP, Slomp CP, Gustafsson BG, Andersen TJ. 2010. Phosphorus recycling and burial in Baltic Sea sediments with contrasting redox conditions. Geochim Cosmochim Ac. 74(4):1350–1362.
- Murphy J, Riley JP. 1962. A modified single-solution method for the determination of phosphate in natural waters. Anal Chim Acta. 27:31–36.
- Nausch M, Nausch G, Lass HU, Mohrholz V, Nagel K, Siegel H, Wasmund N. 2009. P input by upwelling in the eastern Gotland Basin (Baltic Sea) in summer and its effects on filamentous cyanobacteria. Estuar Coast Shelf Sci. 83:434–442.
- Nürnberg GK. 1988. Prediction of phosphorus release rates from total and reductant-soluble phosphorus in anoxic lake sediments. Can J Fish Aquat Sci. 45(3):453–462.
- Psenner R, Boström B, Dinka M, Pettersson K,Puckso R. 1988. Fractionation of suspended matter and sediment. Arch Hydrobiol Beih Ergebn Limnol. 30:98–103.
- Ruban V, Lopez-Sanchez JF, Pardo P, Rauret G, Muntau H, Quevauviller P. 2001. Harmonized protocol and certified reference material for the determination of extractable contents of phosphorus in freshwater sediments – a synthesis of recent works. Fresen J Anal Chem. 370(2-3):224–228.
- Ruttenberg KC. 1992. Development of a sequential extraction method for different forms of phosphorus in marine sediments. Limnol Oceanogr. 37(7):1460–1482.
- Rydin E, Karlsson MJM, Jonsson P. 2011. P release from coastal Baltic Sea sediments as estimated from sediment profiles. Estuar Coast Shelf Sci. 92:111–117.
- Søndergaard M, Jensen JP, Jeppesen E. 2003. Role of sediment and internal loading of P in shallow lakes. Hydrobiologia. 506:135–145.
- Steinberg CEW. 2011. Aerobic phosphorus release from shallow lake sediments. Sci Total Environ. 409(21):4640–4641.
- Sun Q, Yao Y, Xu D, Ding SM, Zhang CS. 2013. Distribution of organic phhosphorus forms in Lake sediments. Fresen Environ Bull. 22(4a):1163–1172.
- Tang W, Zhang H, Zhang WQ, Wang C, Shan BQ. 2013. Biological invasions induced phosphorus release from sediments in freshwater ecosystems. Colloid Surf A. 436:873–880.
- Ting DS, Appan A. 1996. General characteristics and fractions of P in aquatic sediments of two tropical reservoirs. Water Sci Technol. 34:53–59.
- Wan GJ, Wan EY, Chen JA, Wan X, Bai ZG, Wu FC, Wang SL, Wang JF. 2010. A study on the environmental bio-geochemical process for a deep-water artificial reservoir: as exemplified by Lake Hongfeng and Lake Baihua, Guizhou, China. Earth Environ. 38(3):262–270. Chinese.
- Wang SR, Jin XC, Zhao HC, Wu FC. 2006. P fractions and its release in the sediments from the shallow lakes in the middle and lower reaches of Yangtze River area in China. Colloid Surf A. 273:109–116.
- Wang SR, Jin XC, Bu QY, Jiao LX, Wu FC. 2008. Effects of dissolved oxygen supply level on phosphorus release from lake sediments. Colloid Surf A. 316(1–3):245–252.
- Wang JQ, Zheng BG, Liu Q, Li Y, Liang L, Zhang JB, Zheng Z. 2012. Water quality monitoring and compensation amount for ecological compensation section in the Hongfeng Lake watershed. Environ Chem. 31(6):917–918. Chinese.
- Wilson TA, Norton SA, Lake BA, Amirbahman A. 2008. Sediment geochemistryof Al, Fe, and P for two historically acidic, oligotrophic Maine lakes. Sci Total Environ. 404:269–275.
- Xia PH, Li QH. 2011. Water environment and ecological restoration of measures at the inflow rivers of Hongfeng and Baihua reservoirs. J Anhui Agricult Sci. 39(12):7344–7346. Chinese.
- Zhang RY, Wu FC, He ZQ, Zheng J, Song BA, Jin LH. 2009. Phosphorus composition in sediments from seven different trophic lakes, China: a phosphorus-31 NMR study. J Environ Qual. 38(1):353–359.
- Zheng LB, Ye Y, Zhou HY. 2004. P forms in sediments of the East China Sea and its environmental significance. J Geogr Sci. 14:113–120.