Abstract
River ecosystems are closely linked to flow velocity, depth, water temperature, and river bed substrates, and the alteration of hydrological regime due to dam operation has significant impacts on the river ecosystem. It is critical for river managers to seek the optimal solution to balance the environmental discharge requirement with hydropower production. This study investigated links between hydropower plant generation and stream habitat conditions in the You-shui River, China, and developed an optimization model to obtain the most beneficial solution for both generating capacity and environmental discharge. The typical annual flow rates were determined, and Chinese sucker (Procypris rabaudi) was chosen as the target fish with 4 life stages to represent the habitat suitability situation under the environmental discharge. The optimal model was designed to be a function of the maximum value of the sum of the power generating function and the overall suitability index (OSI) function. The traditional environmental flow scheme and optional plan with 3 other schemes were also calculated for comparison. The results of this model indicate that traditional environmental discharge methods cannot satisfy the requirement of juvenile, adult, and spawning stages of the target fish. The solution of the optional plan can meet the habitat demand but will significantly reduce the hydropower generation. The analysis indicates that the optimal model could well balance the hydropower production and the ecosystem protection in the You-shui River and is therefore recommended as a suitable dam management method.
Optimization of dam operation is essential to increase ecosystem protection in the downstream of the river and has been previously investigated (Symphorian et al. Citation2003, Richter and Thomas Citation2007, Olden and Naiman Citation2010). The traditional management of dam operation is predominantly guided by hydropower generation (Escudero et al. Citation1996, Barros et al. Citation2003, Vicuña et al. Citation2008). This solution may stop or severely alter the downstream flow and sediment transport pattern, which will affect the relative abundance and relative condition of aquatic species (Ligon et al. Citation1995, Koel and Sparks Citation2002, Choi et al. Citation2005, Gordon and Meentemeyer Citation2006, Agostinho et al. Citation2008, Yao et al. Citation2014a). River managers are turning from power energy-oriented operations to ecologically based river ecosystem restoration activities to improve degraded streams and river ecological environment (Schmidt et al. Citation1998, Hart et al. Citation2002, Palmer et al. Citation2005, Renöfält et al. Citation2010, Chen et al. Citation2013, Yao and Rutchmann Citation2014). Moreover, preservation of aquatic ecosystems is among the highest priorities and one of the greatest challenges for river management authorities in some streams (Mackenzie Citation1996, Koel and Sparks Citation2002). For example, an increasing number of dams are being removed to meet concerns about the environment and ecosystems, especially to improve water quality and restore fisheries, which could result in outcomes beneficial to ecosystems (Kanehl et al. Citation1997, Bednarek Citation2001, Johnson and Graber Citation2002, Doyle et al. Citation2005, Miao et al. Citation2014). It is therefore important and significant to maintain a certain ecological discharge and suitable hydraulic conditions of velocity, depth, water temperature, and substrates for downstream fish species.
To mitigate the effects of power-oriented activities and retain the ecosystem, especially the downstream river ecosystem, an environmental discharge program has been proposed that releases a certain proportion of the total flow discharge to meet the stream flow requirement of the fish species (Acreman and Dunbar Citation1999, Mallekh et al. Citation1999, Tharme Citation2003). This program has received much attention and has been applied by many river management authorities (Hughes Citation2001, Arthington et al. Citation2003, Shafroth et al. Citation2010). In those rivers in which it has been applied, most of the plans have proposed a constant flow discharge as a fixed value percentage in the range of 2 to 5% of the total discharge (Zheng Citation2010); however, many researchers argued that the current environmental discharge should be increased to 10%, or even to 20%, of the total discharge (Wang et al. Citation2010). Researchers also suggested that target fish species preference and different seasons are factors that should be considered in the environmental discharge program (Poff et al. Citation1997, Yao et al. Citation2014a, Citation2014b). Many experts are against this proposal because increasing the environmental discharge may cause significant loss of hydropower generation, and taking the flow seasonality into account would increase the difficulty of river management (Suen and Eheart Citation2006, Renöfält et al. Citation2010, Chen et al. Citation2013). Thus, a balance and optimization between protection of aquatic ecosystems and economic benefits should be made.
With the advancement of numerical simulation, the environmental discharge should be defined according to the target fish species’ suitability because the environmental discharge is variable for different life stages of fish (Poff et al. Citation2010, Yao et al. Citation2014a, 2015b, 2014c). Following previous research on environmental discharge, a new river eco-friendly dam optimization system has been developed that could increase stream ecosystem benefits and services while protecting or balancing economic benefits, especially for diversion-type hydropower plants. The main objectives of this study were (1) to assess the target fish habitat suitability conditions and hydropower production under the traditional environmental discharge; (2) to evaluate the habitat condition and energy output under the optional environment flow scheme; and (3) to develop an optimal model that could benefit both target fish habitat and hydropower production.
Study site
The study area for this model is You-shui River, 3.5 km long and ∼70 m wide, located downstream of Guanyin-Ping Dam, Hubei Province, China (109°94′E; 29°93′N; ). You-shui River is an important geological area with 5 types of surficial deposits in the study area: sand (0.3–2.0 mm), gravel (2.0–64.0 mm), cobble (64.0–250.0 mm), boulder (250.0–4000.0 mm), and bedrock (solid rock). The deposits in the river occur due to stream flow and sediment transport, large floods, and glacial activity in the Rocky Mountains in China. The cross-sections of the river are narrow, with steep slopes on both river banks creating a “V” shape near the Guanyin-Ping Dam and a “U” shape in the downstream of the river (). The river bed material composition was divided into 3 layers—the active layer, substrate layer, and rocky layer Fig. 2)—according to erosion, deposition, and geological structure. The typical annual flow is steady at ∼50 m3/s from January to May, increases beginning in May–Jun, then peaks at ∼175 m3/s in August before returning to previous levels in November (Fig. 2). The temperature near the riverbank and the backwater nursery areas is 16 C, and the main channel temperature is about 10–14 C.
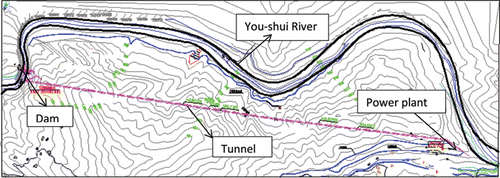
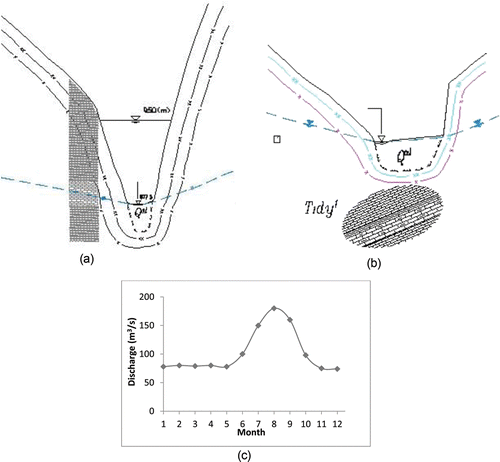
The You-shui River also plays an important role in creating and maintaining diverse habitat conditions for many fish species such as Chinese sucker (Procypris rabaudi), Black carp (Mylopharyngodon piceus), and Silver carp (Hypophthalmichthys molitrix). After the Guanyin-Ping Dam construction, however, the flow regimes of the river have been greatly altered, with a large proportion of water flowing into the diversion tunnel (Fig. 1). These flow alterations have resulted in physical and ecological changes to the You-shui River, including changes of river flow rate, water depth, riverbed substrates, and fish habitat suitability conditions. To fulfill the ecological requirements and create suitable physical habitats for fish species in the You-shui River, the target fish, chosen by its predominance in the river and endangered status, should be reviewed, and a suitable hydraulic dam operation should be planned. In this study, the Chinese sucker (Procypris rabaudi) was chosen as the target fish because it represents a major fish population in the You-shui River and is on the list of National Fish Protection. The life pattern of Chinese sucker (Procypris rabaudi) can be divided into 4 life stages: larvae (Jun–Jul), juvenile (Aug–Sep), adult (Octo–Feb) and spawning (Mar–May), each with distinct preference curves for water flow, depth, and temperature, and substrate composition (.
Methods
The optimal model system consisted of 2 models: (A) the hydropower calculation model and (B) the habitat suitability model. The habitat model has 3 parts: (1) the hydrodynamic model, (2) the heat transfer model, and (3) the sediment transport model. The finite volume method (FVM), which can be represented as a flow chart (), was used to obtain the numerical solution by applying the appropriate boundary conditions.
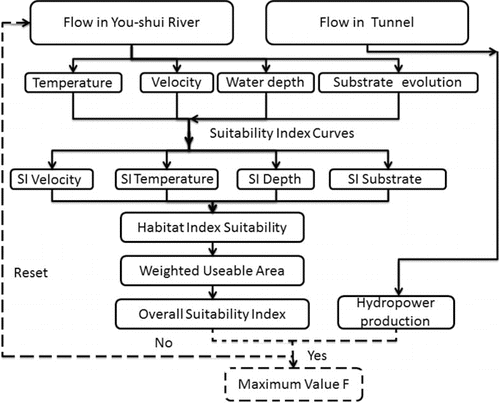
Optimization model development
The optimization model consisted of an objective function that takes into account both the dam operation and the ecological flow requirement. It was composed by weights of hydropower energy and habitat situation of fish species. The function was defined as:
(1)
where E is hydropower generation, OSI is the overall suitability index, and α and β are constants (α is 1/30,000; β is 1.05). F is the optimization output.
Hydropower calculation model
To calculate the hydropower generated by the dam (E), the following equation was used:
(2) where Q is discharge, H is water head for power generation (m), and η is hydropower efficiency in the range of 0.8–0.98 (0.81 was chosen in this study). H is defined as:
(3) where Hr is the relative water head (70 m), and Hf is the head loss, calculated by:
(4)
λ was calculated from:
(5)
(6)
where Re is the Reynolds number, v is kinematic viscosity (0.0131 cm2/s), and V is velocity in the tunnel.
Habitat suitability model
To calculate the OSI for the optimization model, the following equation was used:
(7a)
where weighted usable area (WUA) is given by:
(7b)
The habitat suitability index (HSI) and was calculated using:
(8)
where SIv, SId, SIt, and SIs were suitability index curves for velocity, water depth, temperature, and substrates, respectively. These fish preference curves are mainly from the literature review, professional judgement, and interpreted other fish species (Liu and He Citation1992, Liu et al. Citation2002, Yao et al. Citation2014a; Gui et al. unpubl. data, 2007). The habitat suitability model consisted of 3 parts, detailed in subsequent sections.
Hydrodynamic model
The depth-integrated 2-dimensional water equations obtained from the Navier-Stokes equations can be used to study the river with a reasonable amount of accuracy and efficiency. The hydrodynamic model was developed based on the Navier-Stokes equations. The momentum equations for depth-integrated 2-dimensional turbulent flows were:
Momentum equations:
(9)
(10)
Heat transfer model
The heat transfer model was based on:
(11)
where u and v are depth-integrated velocity components in the x and y directions, respectively; t is time; g is the gravitational acceleration; η is the water surface elevation; ρ is the density of water; h is the water depth; fcor is the Coriolis parameter; τxx, τxy, τyx, and τyy are depth-integrated Reynolds stresses; τbx and τby are shear stresses on the bed and flow interface; T is flow temperature; and ϵ is the heat transfer diffusion coefficient.
Free-surface elevation was calculated using the depth-integrated continuity equation:
(12)
The turbulence Reynolds stresses in Equationequations (9)(9) and Equation(10)
(10) were approximated according to the Bousinesq's assumption that they are related to the main rate of the strains of the depth-averaged flow field with a coefficient of eddy viscosity:
(13)
(14)
where cf is empirical friction coefficient, and vt is the eddy viscosity calculated from turbulence energy k and the dissipation rate ϵ:
(15)
The turbulence parameters k and ϵ in k-ϵ turbulence were defined by the following model transport equations:
(16)
(17) where
(18)
(19)
where Ph was the production of turbulent kinetic energy due to shear stresses with horizontal mean-velocity gradients; Pkv and Pϵv were production of k and ϵ, respectively; U* was bed shear velocity; and c1 = 1.44, c2 = 1.92, σk = 1.0, and σϵ = 1.3, σk = 0.7.
Sediment transport model
The sediment transport and riverbed evolution change, Zb, was calculated using the overall mass balance equation for bed load sediment (Coleman and Nikora 2009):
(20) where p is porosity of the bed material, and Qbs and Qbn are bed-load flux in the main-flow direction (s) and cross-flow direction, respectively (n).
To calculate the bed load transport rate, a large number of semi-empirical formulas were used, including the Meyer-Peter Müller, Einstein-Brown, England Hansen, and Van Rijn equations (Wu et al. Citation2000). The validity range of these sediment transport formulas and the graded sediments in the river determined the formula to be used. In this study, the appropriate bed load function was the Mayer-Peter Müller formula and can be used to calculate the bed load transport rate as follows:
(21a)
(21b)
where C is Chézy friction coefficient, C90 is grain related Chézy value, θ is Shields parameter, θcr is critical Shields value, , d50 = fractional grain size, and τb is shear stress.
Numerical solution procedure and boundary condition
The governing equations in the hydrodynamic, heat transfer, and sediment transport models were discretized using FVM to obtain the numerical solution. For the hydrodynamic model the inlet boundary condition was given in the form of flow rate, whereas the outlet boundary condition was given in the form of water elevation. The boundary conditions of the sediment transport model were the same as that of the hydrodynamic model. As for the heat transfer model, the measured flow temperature in the inlet and zero gradient outflow boundaries were applied as boundary conditions; isothermal boundary conditions were used on the side boundary. More details can be found in Anderson (Citation1995), Chung (Citation2010), and Yao et al. (Citation2014a, Citation2014c).
Results
The eco-hydraulic model was applied to the 4 life stages of the target fish in You-shui River with a computation domain 3.2 km in length by 100 m in width (Fig. 1). For comparison, the dam operation with traditional environmental discharge (4 m3/s) was researched and used as a reference. Dam operation with an optional plan having 3 different environmental flow conservation plans (10, 20, and 40% of total discharge, as options 1, 2, and 3, respectively) was also considered. The hydraulic operation of the dam for environmental flow based on the optimization model function was finally proposed.
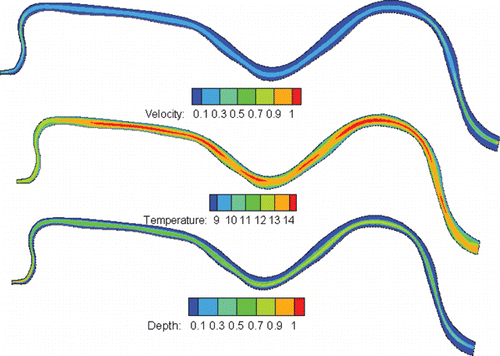
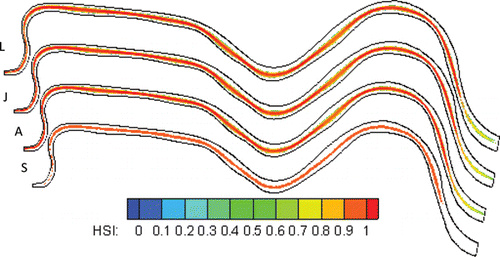
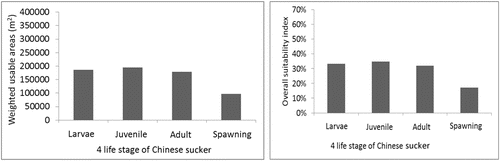
Dam operation with traditional environmental discharge
With the traditional environmental discharge (4 m3/s), the corresponding hydropower productions () and the hydrodynamic results () suggest that energy output has similar trends with the discharge. The energy output was steady at 4.5 × 104 kWh until May and then rose steadily, peaking at 1.1 × 105 kWh. The hydropower production then decreased dramatically to 4.3 × 104 kWh in November and December.
The hydrodynamic model results indicated that only the middle of the river had water, and other river areas were dry. The mean velocity in the river was 0.25 m/s and the maximum velocity was 0.5 m/s. The flow depth was 0.6 m with a maximum value of 1 m (Fig. 6). The flow temperature simulation results were also recorded (Fig. 6).
The habitat suitability conditions were calculated combining the suitability index values for the flow velocity, water depth, water temperature distribution, and substrate types using Equationequation 8(8) . The 4 life stages of Chinese sucker in You-shui River were evaluated for HSI distribution (). Due to the low flow discharge in the computation river, only parts of the computation domain had HSIs. For the life stage of larvae, the habitat suitability was good except in the upstream and downstream of the river, which had relatively lower HSIs. In the middle stream, the river side had an ideal HSI higher than the middle of the river. The habitat suitability levels for juvenile and adult life stages were similar and better than for the larval life stage. The high HSIs for juvenile and adult life stages of the target fish mainly occurred in the middle of the river. Compared to the larvae, juvenile, and adult life stages, the habitat suitability level for spawning life stage was the worst, with low HSI distribution in the majority computation domain, and a few slices of areas scattered in the river had suitable HSI.
The simulation results of WUA and OSI based on equations 7a and 7b () show that the WUA values were 186,589 m2, 194,839 m2, 179,200 m2, and 96,320 m2 for larvae, juvenile, adult, and spawning life stages, respectively. The OSI values for these 4 life stages were 0.33, 0.35, 0.32, and 0.17 respectively.
Optional dam operation with three different conservation plans
The dam operation schemes for fish conservation plans with 10, 20, and 40% of discharge were proposed and the energy output was determined (). Compared to the traditional environmental scheme, we noted that the hydropower energy output decreased by increasing the environmental flow. To be specific, the highest power generation for these 3 optional schemes occurred in August with the values 9.7 × 104, 8.4 × 104, and 6.1 × 104 KW, respectively. The total power generation output for these dam operation schemes was less than the traditional scheme.
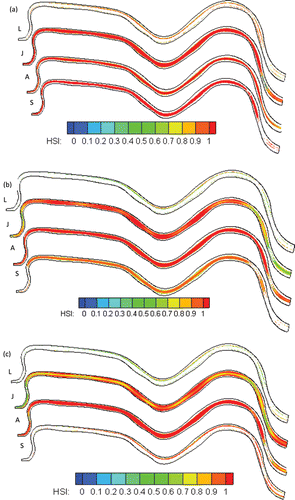
The simulation results of fish habitat situation based on the option 1, option 2, and option 3 schemes () were recorded: (1) For the 10% scheme (Fig. 10a), we found that for the larvae life stage, the habitat suitability level was less than satisfactory. The main parts of the river were unsuitable for larval Chinese sucker, mainly due to the river bed geometry and velocity. Habitat modeling indicates that the target fish in the juvenile and adult life stages had good habitat suitability conditions in the majority of the river. For the spawning life stage, the habitat suitability level was worse than the juvenile and adult life stages but better than the larvae life stage; (2) For the option 2 scheme, the larval and adult life stages had the same HSI distribution trends as the option 1 scheme. For the juvenile life stage, the habitat suitability conditions were similar except that the HSI in several minor areas was lower than the option 1 scheme. The spawning life stage habitat situation was much better than the first scheme; and (3) For the option 3 scheme, the HSI distribution in all life stages of target fish followed nearly the same trend as the option 2 plan due to the increase of the flow width in the river, whereas the velocity and water depth, substrates, and temperature remained unchanged.
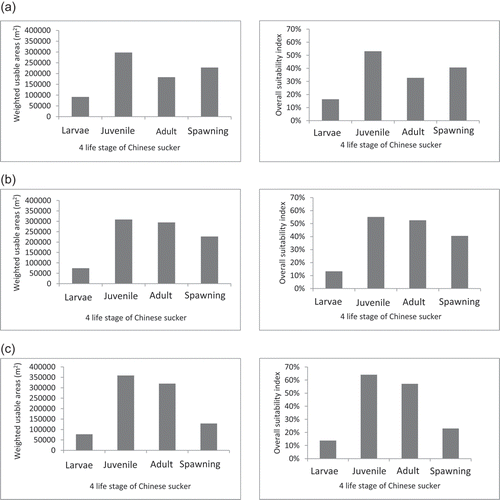
Sensitivity analysis was performed to evaluate the impact of different environmental schemes leading to changes in WUA and OSI. The results indicated that the larval life stage OSI for these 3 environmental flow schemes was nearly stable, with a value of 0.14. The OSI for the other 3 life stages of the target fish was better than the larval life stage. Specifically, the OSI values for the juvenile life stage increased from 0.53 to 0.55 and to 0.64 for the first, second, and third environmental flow schemes, respectively. For the adult life stage, the OSI value increased from 0.33 to 0.53 and to 0.57 for the first, second, and third environmental flow schemes, respectively. By contrast, the OSI for the spawning life stage decreased from 0.41 to 0.40 and to 0.23 for the first, second, and third environmental flow schemes, respectively (). The WUA follows the same trend as the OSI (Fig. 11).
Balance between fish habitat conservation and energy output
To create a balance between the hydropower generation and downstream fish habitat conservation, the optimal model was proposed. The relationship between hydropower loss and conservation of Chinese sucker habitat in the You-shui River () shows that, in a typical year, the hydropower decreased along with the increase of environmental discharge in the river. The OSI of 4 life stages experienced an increase first, however, before decreasing along with the increase of environmental discharge in the river. The hydropower output (Fig. 12)also suggests that the dam operation schemes with the maximum F were established when the environmental discharge was 29, 29, 43, and 43 m3/s for larval, juvenile, adult, and spawning stages, respectively. Therefore, the suitable dam operation plan with the F equal to 0.92, 1.21, 1.14, and 1.05 in the 4 life stages, respectively, was proposed as an optimal option for hydropower production and river ecosystem protection in a typical year in the You-shui River.
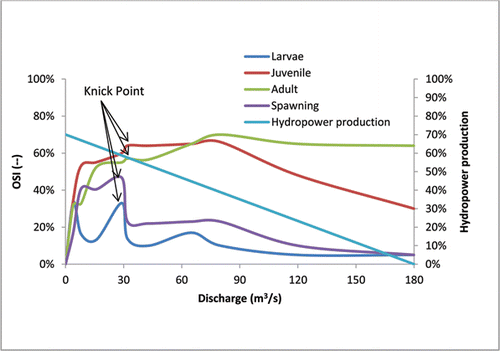
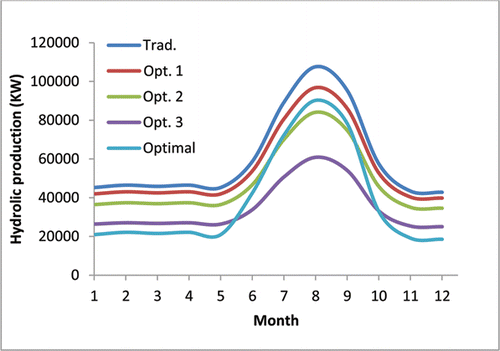
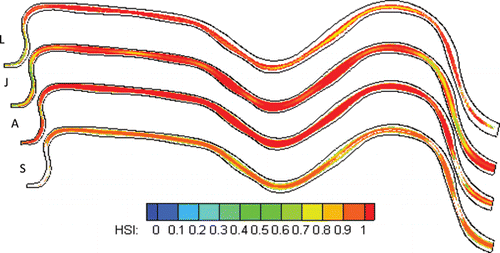
After the maximum F value was established, the discharge in the tunnel, hydropower production, and environmental discharge were also determined (Fig. 13). Note that from January to May the hydropower production remained at 2.2 × 104 kWh and then rose steadily and peaked at 9.0 × 104 kWh. Following that peak, the hydropower production experienced a dramatic decrease to 1.9 × 104 kWh in November and December.
The habitat suitability situation under the optimal model () suggests that the 4 life stages of target fish will remain in a stable situation, and the WUA and OSI will have high values. Specifically, the WUA values were 185,069, 347,351, 316,763, and 261,887 for the larval, juvenile, adult, and spawning life stages, respectively, and the OSI values were 0.33, 0.62, 0.57, and 0.47, respectively ().
Discussion
The values of F were compared for the traditional, 3 optional, and optimal schemes (). The F values for the optimal scheme are clearly higher than the other schemes for all life stages, with values of 0.92, 1.21, 1.14, and 1.05 for larvae, juvenile, adult, and spawning, respectively. Also note that in the larval stage, the F values for the traditional scheme are better than the optional 3 schemes, and for the other stages, the F values with the traditional scheme are lowest among all schemes.
Comparing the results of traditional and environmental flow approaches and the results determined by the optimal model indicates that the optimal model has advantages in several respects. First, the optimal model considers the benefits of both hydropower production needed by humans and river habitat demanded by fish species. In addition, the optimal model systematically analyzes the river hydrodynamic variables, target fish, and their ecological characteristics, which could significantly improve the accuracy of the optimal model. Additionally, based on the habitat conditions and seasonal needs of the target fish species, the life stage of the target fish was divided into 4 stages, which helps to increase the hydropower production in rivers without reducing the OSI in rivers. This approach is particularly recommended by researchers and experts (Acreman and Dunbar Citation1999, Olden and Naiman Citation2010).
The optimal model proposed in this study was obtained after considering several important aspects and could be widely accepted and used by both river managers and ecologists. River managers often must focus on providing the minimum environmental discharge intended to fix the flow values to protect river ecosystems; however, fish abundance is strongly associated with flow and river habitat suitability, and the fixed low discharge often cannot meet the requirements of the ecosystem in rivers and streams. A significant decline of hydropower is also an unacceptable strategy for a river manager, however. Many researchers also recommend the optimal model as a dam management method (Palmieri et al. Citation2001, Chen et al. Citation2013).
Table 1 Comparison of F values under the traditional, 3 optional, and optimal environmental flow schemes.
Basically, dam management strategies should be included as both a hydropower production strategy and an ecosystem protection strategy. The application of the new optimal model in the You-shui River, and the comparison with other methods, gives confidence to the accuracy and advantages of model simulation and evaluation.
Conclusions
We developed an optimal dam operation model for environmental flow in the You-shui River by coupling the hydropower production and the habitat requirements for 4 life stages of a target fish. The target fish suitability index curves for velocity, water depth, water temperature, and substrates were selected to calculate the fish habitat protection, and a typical annual flow pattern was used for simulation. A comparison of production output and habitat situation was also made among the optimal model, the traditional scheme, and the habitat scheme.
The calculated results indicate that under the traditional environmental flow regime, the habitat suitability level in You-shui River is poorly suited for the target fish at all life stages except the larval stage. Although this result would not satisfy the requirement of a healthy river ecosystem, the hydropower energy output was the largest for this scheme among the 3 environmental flow schemes. To improve the habitat suitability level in the You-shui River, the environmental discharge enhancement was used. This solution could satisfy the demands to protect habitat when the river discharge is 40% of the total flow, but would significantly reduce hydropower production, which is unacceptable for water managers. Due to the requirement of hydropower energy by water managers and suitable habitat levels for fish, the optimal dam operation model was proposed and calculated. This simulation indicated that our optimal dam operation model can help balance ecosystem requirements for fish and hydropower energy needs by humans.
Although the optimal model was only applied to the You-shui River as one case study, it can also be used to evaluate other fish species in other rivers and dams based on the generic formulation. In future works, the fish preference curves could be evaluated, and a more precise solution may be proposed and used. The fish population was also a key factor for ecosystem evaluation, and the population will change by altering the river flow. Additionally, variables such as climate change and habitat patterns could influence ecosystems, fish populations, and species persistence in streams and rivers, and hence need further investigation.
References
- Acreman MC, Dunbar MJ. 1999. Defining environmental river flow requirements–a review. Hydrol Earth System Sci. 8:861–876.
- Agostinho AA, Pelicice FM, Gomes LC. 2008. Dams and the fish fauna of the Neotropical region: impacts and management related to diversity and fisheries. Braz J Biol. 68:1119–1132.
- Anderson JD. 1995. Computational fluid dynamics (Vol. 32). New York: McGraw-Hill.
- Arthington AH, Rall JL, Kennard MJ, Pusey BJ. 2003. Environmental flow requirements of fish in Lesotho Rivers using the DRIFT methodology. River Res Appl. 19:641–666.
- Barros MT, Tsai FT, Yang SL, Lopes JE, Yeh WW. 2003. Optimization of large-scale hydropower system operations. J Water Resour Plan Manage. 129:178–188.
- Bednarek AT. 2001. Undamming rivers: a review of the ecological impacts of dam removal. Environ Manage. 27:803–814.
- Chen Q, Chen D, Li R, Ma J, Blanckaert K. 2013. Adapting the operation of two cascaded reservoirs for ecological flow requirement of a de-watered river channel due to diversion-type hydropower stations. Ecol Modell. 252:266–272.
- Choi SU, Yoon B, Woo H. 2005. Effects of dam‐induced flow regime change on downstream river morphology and vegetation cover in the Hwang River, Korea. River Res Appl. 21:315–325.
- Chung TJ. 2010. Computational fluid dynamics. Cambridge University Press.
- Coleman SE, Nikora VI.2009 Exner equation: A continuum approximation of a discrete granular system. Water Resources Research, 45(9).
- Doyle MW, Stanley EH, Orr CH, Selle AR, Sethi SA, Harbor JM. 2005. Stream ecosystem response to small dam removal: lessons from the Heartland. Geomorphology. 71:227–244.
- Escudero LF, de la Fuente JL, Garcia C, Prieto FJ. 1996. Hydropower generation management under uncertainty via scenario analysis and parallel computation. IEEE Transactions on Power Systems. 11:683–689.
- Gordon E, Meentemeyer RK. 2006. Effects of dam operation and land use on stream channel morphology and riparian vegetation. Geomorphology. 82:412–429.
- Hart DD, Johnson TE, Bushaw-Newton KL, Horwitz RJ, Bednarek AT, Charles DF, Velinsky DJ. 2002. Dam removal: challenges and opportunities for ecological research and river Restoration. BioScience. 52:669–682.
- Hughes DA. 2001. Providing hydrological information and data analysis tools for the determination of ecological instream flow requirements for South African rivers. J Hydrol. 241:140–151.
- Ligon FK, Dietrich WE, Trush WJ. 1995. Downstream ecological effects of dams. BioScience. 45:183–192.
- Liu S, Chen D, Duan X, Qiu S, Wang L. 2002. Status of Reeves shad and corresponding resources protection measures in China. Aquat Biol. 26:679–684.
- Liu J, He B. 1992. China freshwater fishery cultivation. Beijing (China): Science Press. Chinese.
- Kanehl PD, Lyons J, Nelson JE. 1997. Changes in the habitat and fish community of the Milwaukee river, Wisconsin, following removal of the Woolen Mills Dam. N Am J Fish Manage. 17:387–398.
- Koel TM, Sparks RE. 2002. Historical patterns of river stage and fish communities as criteria for operations of dams on the Illinois River. River Res Appl. 18:3–19.
- Johnson SE, Graber BE. 2002. Enlisting the social sciences in decisions about dam removal. The application of social science concepts and principles to public decisionmaking about whether to keep or remove dams may help achieve outcomes leading to sustainable ecosystems and other goals in the public interest. BioScience. 52:731–738.
- MacKenzie SH. 1996. Integrated resource planning and management: the ecosystem approach in the Great Lakes basin. Washington (DC): Island Press.
- Mallekh R, Boujard T, Lagardère JP. 1999. Evaluation of retention and environmental discharge of nitrogen and phosphorus by farmed turbot (Scophthalmus maximus). N Am J Aquacult. 61:141–145.
- Miao DY, Huang WW, Li YP, Yang ZF. 2014. Planning water resources systems under uncertainty using an interval-fuzzy De Novo programming method. J Environ Inform. 24:11–23.
- Olden JD, Naiman RJ. 2010. Incorporating thermal regimes into environmental flows assessments: modifying dam operations to restore freshwater ecosystem integrity. Freshwater Biol. 55:86–107.
- Palmer MA, Bernhardt ES, Allan JD, Lake PS, Alexander G, Brooks S, Sudduth E. 2005. Standards for ecologically successful river restoration. J Appl Ecol. 42:208–217.
- Palmieri A, Shah F, Dinar A. 2001. Economics of reservoir sedimentation and sustainable management of dams. J Environ Manage. 61:149–163.
- Poff NL, Allan JD, Bain MB, Karr JR, Prestegaard KL, Richter BD, Sparks R, Stromberg J. 1997. The natural flow regime: a new paradigm for river in conservation and restoration. Bioscience. 47:769–784.
- Poff NL, Richter BD, Arthington AH, Bunn SE, Naiman RJ, Kendy E, Warner A. 2010. The ecological limits of hydrologic alteration (ELOHA): a new framework for developing regional environmental flow standards. Freshwater Biol. 55:147–170.
- Renöfält B, Jansson R, Nilsson C. 2010. Effects of hydropower generation and opportunities for environmental flow management in Swedish riverine ecosystems. Freshwater Biol. 55:49–67.
- Richter BD, Thomas GA. 2007. Restoring environmental flows by modifying dam operations. Ecol Soc. 12:12.
- Schmidt JC, Webb RH, Valdez RA, Marzolf GR, Stevens LE. 1998. Science and values in river restoration in the Grand Canyon. BioScience. 48:735–747.
- Shafroth PB, Wilcox AC, Lytle DA, Hickey JT, Andersen DC, Beauchamp VB, Warner A. 2010. Ecosystem effects of environmental flows: modelling and experimental floods in a dry land river. Freshwater Biol. 55:68–85.
- Suen JP, Eheart JW. 2006. Reservoir management to balance ecosystem and human needs: incorporating the paradigm of the ecological flow regime. Water Resour Res. 42:W03417.
- Symphorian GR, Madamombe E, Van der Zaag P. 2003. Dam operation for environmental water releases; the case of Osborne dam, Save catchment, Zimbabwe. Phys Chem Earth, A B C. 28:985–993.
- Tharme RE. 2003. A global perspective on environmental flow assessment: emerging trends in the development and application of environmental flow methodologies for rivers. River Res Appl. 19:397–441.
- Vicuña S, Leonardson R, Hanemann MW, Dale LL, Dracup JA. 2008. Climate change impacts on high elevation hydropower generation in California's Sierra Nevada: a case study in the Upper American River. Clim Change. 87:123–137.
- Wang GX, Li SL, Chen J. 2010. Flow of the Yangtze River estuary ecology. Hydro Eng. 3:53–58. Chinese.
- Wu W, Rodi W, Wenka T. 2000. 3D numerical modeling of flow and sediment transport in open channels. J Hydraul Eng. 126(1): 4--15
- Yao W, Bui MD, Rutschmann P. 2014b. Application of habitat and population modeling in river management, the case of rainbow trout, p. 207--216. In Internationales Symposium Wasser- und Flussbau im Alpenraum. ETH: Zürich, Switzerland.
- Yao W, Rutschmann P, Sudeep B. 2014a. Modeling of river velocity, temperature, bed deformation and its effects on rainbow trout (Oncorhynchus mykiss) habitat in Lees Ferry, Colorada River. Int Environ Res. 8:887–896.
- Yao W, Rutschmann P, Bamal S. 2014c. Modeling of river velocity, temperature, bed deformation and its effects on rainbow trout (Oncorhynchus mykiss) habitat in Lees Ferry, Colorado River. Int J Environ Res. 8:887–896.
- Yao W, Rutschmann P. 2014. Use of a self-propelled, fish-shaped, computer model to study the effects of shape and tail-beat frequency on torque and velocity in salmon-shaped fish. Mar Freshw Behav Physiol. 47:161–172.
- Zheng CH. 2010. Minimum ecological water demand in the river mud song. Resources and Environment in the Yangtze Basin. 19329--334.