ABSTRACT
Lamothe KA, Allen RM, Winningham K, Dennis C, Johnson RL. 2016. Stocking for a trophy bass fishery: searching for size differences among largemouth bass and hybrids in southern Arkansas reservoirs. Lake Reserve Manage. 32:194–207.
Within the southern United States, game and fish agencies have introduced nonnative Florida bass (FB), Micropterus floridanus (Lesueur 1822), as an alternative to native northern largemouth bass (NLMB), M. salmoides (Lacèpéde 1802), for decades because of suspected maximum size differences between the 2 species. The goal of this study was to determine if bass with differing levels of FB alleles demonstrated differential size structure within 6 southern Arkansas reservoirs stocked with FB. Despite millions of FB being introduced into these reservoirs over the last decade, FB made up <2% of the sampled bass for 5 of 6 reservoirs. Most sampled populations consisted primarily of one species and hybrids genetically similar to that species. In Lake Monticello, where FB numbers were relatively high, FB had significantly greater mean lengths and weights than FB × NLMB hybrids. By contrast, other reservoir bass populations showed no significant pairwise differences among species or their hybrids. From a fisheries management perspective, introducing FB outside its native range remains a controversial issue, and long-term controlled studies are needed in Arkansas to better resolve size differences observed among FB, NLMB, and their hybrids.
Largemouth bass are some of the most popular sport fishes in North America. Resulting from allopatric evolution, 2 distinct species have been identified, although they hybridize when sympatric: Florida bass (FB), Micropterus floridanus (Lesueur 1822) and northern largemouth bass (NLMB), Micropterus salmoides (Lacèpéde 1802; Kassler et al. Citation2002, Near et al. Citation2003, Citation2004). For clarity, the term “largemouth bass” here refers to both NLMB and FB inclusively due to a long history of lumping these 2 species together as one. Historically, FB were restricted to peninsular Florida, whereas NLMB were found throughout the Midwest and northeast United States (Lee et al. Citation1980). Today, NLMB and FB have been stocked in freshwaters around the globe and represent 2 of the most valuable freshwater fish species for recreational angling.
Trophy bass angling has become a major emphasis for recreational anglers in recent years (Wilson and Dicenzo Citation2002, Dotson et al. Citation2013). For several decades, freshwater game and fish agencies of the southern United States have been stocking FB outside their native range into reservoirs with resident populations of NLMB because of FB's reputation for a larger growth potential than local NLMB (Barthel et al. Citation2015). Support for this reputation comes from genetic monitoring programs in Oklahoma (Horton and Gilliland Citation1993), Texas (Lutz-Carrillo et al. Citation2006, Tibbs Citation2008), Louisiana (Hughes and Wood Citation1995), and Arkansas (Lamothe and Johnson Citation2013), which have shown most trophy bass to be FB or recent hybrids (e.g., F1-generation).
In contrast to identifying the genotypes of trophy bass, studies measuring long-term performance differences among bass species and their hybrids in natural systems has proven difficult (Maceina and Murphy Citation1988, Johnson and Fulton Citation1999). Early controlled pond studies were typically short in duration, performed at varying latitudes, and demonstrated differing performance characteristics (e.g., mortality, growth, relative weight) of these species and their hybrids; nonetheless, results have been inconsistent (Isely et al. Citation1987, Kleinsasser et al. Citation1990, Horton and Gilliland Citation1993).
The Arkansas Game and Fish Commission (AGFC) has been evaluating the efficacy of stocking FB in southern Arkansas reservoirs for more than 2 decades. Historically, fixed allozyme loci were used for identification purposes (Philipp et al. Citation1983, Maceina et al. Citation1988); more recently, hypervariable microsatellite markers have been developed that provide greater reliability in genetic assessment (Lutz-Carrillo et al. Citation2006). These markers have been used to describe the genetic composition of largemouth bass populations across several states (Lutz-Carrillo et al. Citation2006, Barthel et al. Citation2010), including Arkansas (Allen et al. Citation2009, Lamothe et al. Citation2012).
Approximately 60% of the largemouth bass stocked in Arkansas are FB (Siepker and Casto-Yerty Citation2008). The AGFC has traditionally employed 2 approaches for stocking FB in reservoirs: (1) stocking FB into reservoirs with established NLMB populations, and (2) stocking FB into newly constructed or renovated lakes following a large-scale fish kill. Over the last decade, ∼1 million FB fingerlings have been stocked in Arkansas reservoirs each year. The primary management focus to date has been on the degree of incorporation of FB and their alleles into these systems. Lesser studied is whether stocked FB and their hybrid progeny out-perform local NLMB stocks. Therefore, we set out to answer 2 questions: (1) are FB differentially represented among longer, heavier, and older bass within these Arkansas reservoirs; and (2) do bass with differing contributions of FB alleles show differences in length and weight measurements within these Arkansas reservoirs? Because FB have been differentially represented among trophy largemouth bass in Arkansas (Lamothe and Johnson Citation2013), we hypothesized that FB and bass with high levels of FB alleles would be overrepresented among longer, heavier, and older bass.
Methods
Study locations
The AGFC has stocked 13 reservoirs with FB and sample all public reservoir bass populations where FB have been introduced. In this study, reservoirs stocked for >20 yr (n = 6) and designated as Trophy Largemouth Bass lakes by the AGFC (J. Risley, AGFC, pers. comm.) were selected to ensure the opportunity that introduced FB had matured (). All 6 of these reservoirs are located in southern Arkansas (), where water temperatures do not typically fall below 4–5 C.
Table 1. Lake characteristics and stocking information for each reservoir up to the final year of sampling. Lake Chicot is a natural oxbow of the Mississippi River. Number of stocking events (in parentheses) and total density of fish stocked rounded to the nearest whole number (number of fingerlings stocked/ha). Some yearlings were also stocked in Columbia and Lower White Oak lakes.
Figure 1. Lakes Millwood (n = 232), Lower White Oak (n = 296), Monticello (n = 478), Erling (n = 356), Columbia (n = 341), and Chicot (n = 436) in southern Arkansas. Pie charts display proportions of each genetic group for all sampled bass.
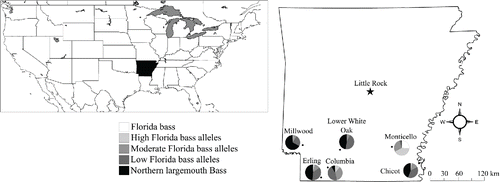
Stocking densities and duration of FB were highly variable among lakes (). Two of these reservoirs, Lakes Columbia and Monticello, were constructed in 1986 and 1992, respectively, and subsequently stocked with FB. Columbia Lake was constructed by the damming of Beech Creek and initially stocked with 40,000 FB fingerlings for 1 year, then with ∼90,000 NLMB fingerlings over a 3-year period, and FB annually thereafter. Lake Monticello was stocked solely with FB following construction and is fed by small streams. Four other lakes (Chicot, Erling, Lower White Oak, and Millwood) had established NLMB populations before being stocked on a regular basis with FB (). Lake Chicot is an oxbow of the Mississippi River, whereas all other waterbodies studied were reservoirs. Additionally, lakes Chicot and Erling were stocked episodically with NLMB (). Lake Millwood was stocked once in 1996 with ∼31,600 NLMB fingerlings, but was stocked regularly with FB before and following that single NLMB stocking event. Several lakes were occasionally stocked with low numbers of adults when hatchery production outpaced demand.
Bass sampling
The AGFC began biannually sampling largemouth bass from reservoirs stocked with FB in 2006 to determine the genetic constitution of these populations. Bass were sampled from each reservoir in spring or fall using a boom-mounted boat electrofishing unit. Fish were measured (TL, mm) and weighed (g), and fin clips were taken and preserved in ethanol for genetic analysis. The goal during collection was to sample a broad representation of bass sizes, particularly those greater than stock size (200 mm), with 10 fish per 25 mm set as a target. Sample numbers were therefore not representative of lake population size structures. Individuals were classified as 1 of 4 categories by length: less than stock size (0–199 mm), stock size (200–299 mm), quality size (300–379 mm), and preferred size (380+ mm; Gabelhouse Citation1984).
Sagittal otoliths were removed from a random subset of the pre-spawn largemouth bass for estimation of age from Lakes Columbia (n = 119), Erling (n = 60), Millwood (n = 69), and Monticello (n = 179) in 2006. Thin sectioning of otoliths was performed longitudinally with a Buehler isomet low-speed saw. Sections were then mounted on petroglyph slides and polished for visualization of annuli, which were then observed and counted using light microscopy (Buckmeier and Howells Citation2003).
Genomic DNA was extracted from 20–25 mg tissue samples of each individual using a modified version of the chloroform tris-acetate borate extraction method (Allen et al. Citation2009). Genotypes were amplified using 7 microsatellite loci: Mdo3, Mdo6, Mdo7, Lma12, Ms13, Ms21, and Msas29 (Colbourne et al. Citation1996, DeWoody et al. Citation2000, Malloy et al. Citation2000, Seyoum et al. Citation2013), with PCR parameters outlined by Lutz-Carrillo et al. (Citation2006). Microsatellite primers were synthesized with distinct fluorescent tags (Integrative DNA Technologies, Coralville, IA), specific for capillary electrophoresis using a Beckman-Coulter CEQ8000 Genetic Analysis System. Fragment sizes were determined by the internal CEQ8000 analysis program using a 400 base pair standard and manually confirmed.
Allen et al. (Citation2009) found that in addition to bass alleles in Arkansas reservoirs being exclusive to FB and NLMB, some alleles were also shared between species. To confirm this finding, STRUCTURE 2.3 (Pritchard et al. Citation2000) was first run using an admixture model with correlated allele frequencies and default settings to establish pure species and their hybrids (50,000 burn-in steps; 500,000 Markov Chain/Monte Carlo steps). Consistent with Allen et al. (Citation2009), the number of clusters (k) was identified as 2, with values ranging from 0.0 (FB) to 1.0 (NLMB).
Admixture proportions were used to classify individuals as either pure species or hybrids, following 0.05 thresholds to limit Type I errors (Schwartz and Beheregaray Citation2008). Individuals with q ≥ 0.95 were classified as NLMB, whereas individuals with q ≤ 0.05 were classified as FB. All broodstock controls were within this threshold and distinguished as pure species (NLMB: Joe Hogan Hatchery, n = 32, q = 0.996; William Donham Hatchery, n = 42, q = 0.989; FB: Andrew Hulsey Hatchery, n = 83, q = 0.002). Individuals having intermediate q-values were classified as hybrids and assigned to 1 of 3 categories of FB allele frequencies (low: 0.949–0.651; moderate: 0.351–0.650; and high: 0.350–0.051). Each FB broodfish is genetically tested annually using allozyme analysis to attempt to maintain pure species lines.
Data analyses
The primary objective of the AGFC in sampling these reservoirs was to understand the genetic composition of the bass populations; therefore, these data present challenges for investigating differences in size among NLMB, FB, and their hybrids. For example, genetic bass groupings showed skewed distributions and were differentially represented within samples; most often, FB were infrequent. Additionally, due to the nature of using admixture proportions to differentiate species, some individuals were classified as hybrids, although their admixture proportion may fall just outside the 0.05 threshold (e.g., q = 0.94, described as low FB alleles). Further, there are assumptions associated with using sampled genetic group frequencies as the expected values, such as that FB, NLMB, and their hybrids have an equal probability of being caught using boat electrofishing methods.
To investigate differences in size among genetic groups of largemouth bass within Arkansas reservoirs and to address the statistical challenges that our data presented, a permutational nonparametric multivariate analysis of variance (PERMANOVA) was performed on log transformed length and weight measurements. PERMANOVAs were performed with 9999 permutations using the adonis function from the “vegan” package in R (Anderson Citation2001, Oksanen et al. Citation2015, R Core Team Citation2015). This method was chosen over the parametric multivariate analysis of variance and multivariate regression due to the violation of normality and homogeneity of variance and covariance matrices. Pairwise comparisons were made using the pairwise.perm.t.test function from the “RVAideMemoire” package using 9999 permutations (Hervé Citation2014) if significance was found at an alpha = 0.05. Due to the high number of pairwise comparisons, family-wise Type I error was controlled using the false discovery rate argument (Benjamini and Hochberg Citation1995).
Additionally, to investigate differences in weight × length relationships among genetic groups within reservoirs, a permutational analysis of covariance (pANCOVA) was performed. A permutation approach was chosen, again, due to the violation of normality. The following formula was used in the analysis: log(Weight)∼log(Length) × Genetic Group. The pANCOVA model was first run to provide an observed F-value and subsequently 9999 times with randomizations of individuals among genetic groups to develop the randomized F-distribution for significance thresholds (Manly Citation2007).
Analyses of the Lake Monticello bass
Lake Monticello is considered the premier trophy bass lake in the state of Arkansas (AGFC Citation2002, Risley and Johnson forthcoming), and therefore the AGFC samples this reservoir more frequent and intensively than other reservoirs in the state. A G-test of goodness-of-fit was used to determine if genetic groups were differentially distributed among age or weight groups within Lake Monticello. Expected frequencies were the overall sampled genetic group frequency within a reservoir. The G-test was used to compare the within-reservoir frequencies of bass >age 6 and bass >2268 g relative to the overall sample frequencies. This age and weight were chosen to be consistent with AGFC black bass management goals for establishing quality bass fisheries (AGFC Citation2002), which identifies 5 lb (2268 g) as a trophy bass. In our study, age 6 bass on average weighed 2215.97 ± 773.27 g (mean ± SD).
As described above, a PERMANOVA was used to evaluate length and weight relationships among genetic groups for all reservoirs, and additionally, by sex within Lake Monticello. Moreover, a similar approach was taken using pANCOVA to investigate the differences in length and weight measurements among genetic groups for all sexed Lake Monticello bass. All analyses were run in R 3.1.3 (R Core Team Citation2015).
Results
Genetic characteristics of bass populations
Florida bass made up <2% of the sampled population in 5 of the 6 reservoirs (). Reservoirs primarily stocked with FB after construction had mean q-values of 0.657 (Columbia Lake) and 0.254 (Lake Monticello), whereas Lakes Chicot, Erling, Lower White Oak, and Millwood, which have had FB stocked on top of existing NLMB populations, showed q-values ranging from 0.855 to 0.906 (). A high proportion of individuals in Lake Monticello had high and moderate levels of FB alleles. Surprisingly, most bass of Columbia Lake contained low and moderate levels of FB alleles. The other 4 lake populations sampled were characterized by NLMB and bass having low levels of FB alleles (). Further, many bass of lakes Chicot, Erling, and Lower White Oak not designated as NLMB had q-values ranging from 0.90 to 0.94 (about one-third of bass categorized as having low levels of FB alleles), indicating that many bass of these lakes had a single FB allele.
Figure 2. Frequency of bass genetic groups (FB, high, moderate, and low levels of FB alleles, and NLMB) for stock size or greater bass from Lakes Chicot (n = 423), Columbia (n = 294), Erling (n = 284), Lower White Oak (n = 246), Millwood (n = 273), and Monticello (n = 471).
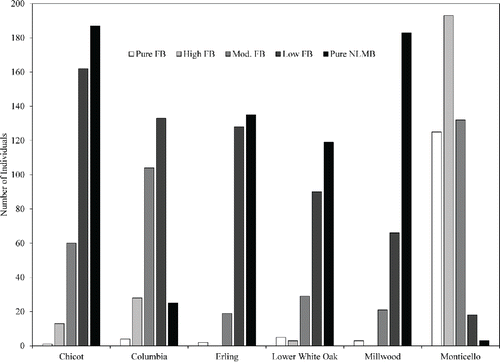
Table 2. Sample years, sample size, and admixture proportions of each largemouth bass population for Florida bass (FB) and northern largemouth bass (NLMB). Joe Hogan and William Donham hatcheries provided NLMB for stocking and Andrew Hulsey hatchery produced FB for stocking.
Investigating size differences among genetic groups
Three of the 6 reservoir populations (Chicot, Millwood, and Monticello) showed significant differences in length and/or weight measurements among genetic groups (). Although bass of lakes Chicot and Millwood having higher levels of FB alleles were generally heavier than bass having lower levels of FB alleles, only the genetic groups in Lake Monticello exhibited significant pairwise differences (). Different from the other lake samples, Lake Monticello did not have a large enough sample size to include NLMB in the analysis of length and weight (n < 5). In Lake Monticello, FB were longer and heavier than all categories of FB × NLMB hybrids (). Among the reservoir bass populations that showed no pairwise or significant difference in length and weight measurements, few FB were identified, therefore limiting the comparisons among genetic groups ().
Table 3. PERMANOVA results comparing log transformed length (mm) and weight (g) between largemouth bass genetic groups classified by q-value (1 = ≤0.05, 2 = 0.051–0.350, 3 = 0.351–0.650, 4 = 0.651–0.949, 5 = ≥0.95) as determined by STRUCTURE for individual reservoir bass populations. The genetic groups included in the test are given. P-values are based on 9999 permutations. N/A = Not applicable.
Within populations, there were no significant differences among genetic groups in slopes of weight × length regressions (). Significant effects of genetic grouping on weight measurements were observed for Lakes Chicot and Millwood (). Lake Chicot bass had decreasing intercept values with increasing levels of NLMB alleles (). Florida bass were not included in the Lake Chicot comparison due to small sample size (n < 5). Among bass sampled from Lake Millwood, bass with low levels of FB alleles had the greatest intercept value, followed by NLMB, and lastly by bass with moderate levels of FB alleles ().
Table 4. Results of the pANCOVA models for log(Weight)∼log(Length) × Genetic Group within reservoirs. P-values are based on 9999 randomizations.
Lake Monticello size structure among genetic groups
Lake Monticello was the only reservoir with a sufficient sample size to compare genetic group distributions of fish >age 6 and >2268 g. Here, significant differences in genetic group distributions were observed for fish >age 6 (G2,103 = 23.793, P < 0.001) and bass >2268 g (G3,71 = 12.863, P = 0.002) when compared to the overall reservoir sample distribution (). Degrees of freedom varied among the tests because there were too few NLMB for the weight comparisons and too few NLMB and individuals with low levels of FB alleles observed older than age 6 in Lake Monticello. Florida bass were observed at greater frequency as length, weight, and age increased ().
Figure 3. Linear regressions of log(Weight) × log(Length) for a) Lake Chicot (n = 433) and b) Lake Millwood (n = 296) bass.
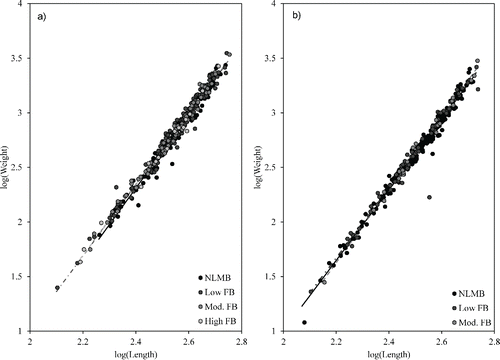
Of the sexed Lake Monticello bass, 3 genetic groups (FB, high, and moderate levels of FB alleles) were represented with a large enough sample size to investigate differences in size among genetic groups within sexes. Significant differences among genetic groups in mean length and weight measurements were observed for females (PERMANOVA: length: F1,81 = 8.644, P = 0.004; weight: F1,81 = 8.412, P = 0.005) but not males (length: F1,84 = 0.497, P = 0.506; weight: F1,84 = 1.600, P = 0.208). Female FB were significantly longer and heavier than females with moderate and high levels of FB alleles (). Furthermore, female bass possessing moderate levels of FB alleles were significantly longer and heavier than individuals with high levels of FB alleles ().
Figure 4. Distribution of bass genetic groups within Lake Monticello for stock size (n = 80), quality size (n = 84), preferred size (n = 307), age >6 (n = 103), bass >2,268 g (n = 71), and overall (n = 478).
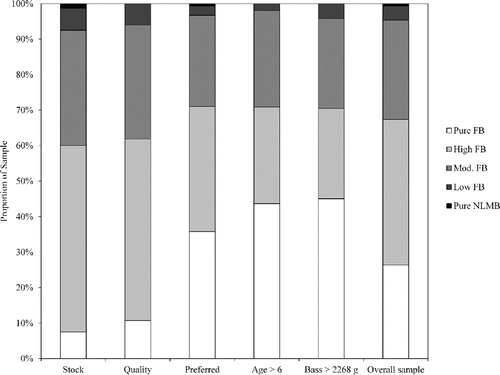
Likewise, there were significant differences among genetic groups in slopes of weight × length regressions for females (F2,78 = 5.139, P = 0.001; ) within Lake Monticello but not for males (F2,81 = 1.988, P = 0.061; ). Among females, FB had the greatest intercept value, followed by bass with moderate levels of FB alleles, and finally by individuals with high levels of FB alleles (). By comparison, among male FB within Lake Monticello, bass with moderate levels of FB alleles had the greatest intercept, followed by FB, and finally by bass with high levels of FB alleles ().
Figure 5. Boxplot of a) Length) and b) Weight for sexed female bass from Lake Monticello (n = 83). Significant pairwise differences between genetic groups determined using a PERMANOVA using log transformed values and are indicated with letters above each boxplot for both variables.
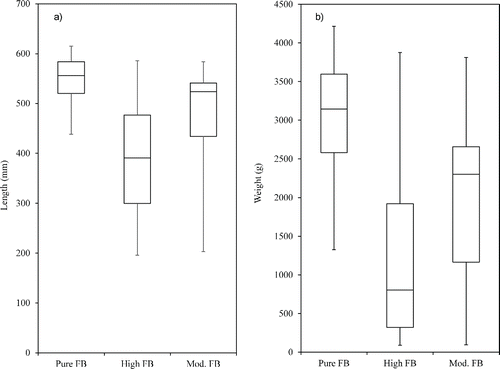
Figure 6. Linear regressions of log(Weight) × log(Length) for a) female (n = 88) and b) male (n = 91) bass from Lake Monticello.
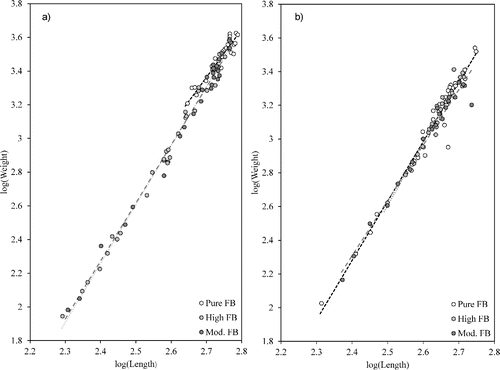
Sample size was reduced substantially for largemouth bass >3.8 kg. The 10 individuals were females from Lake Monticello, with 1 individual each identified as having low, moderate, and high levels of FB alleles, and the remaining 7 fish identified as FB. Further investigation into bass >575 mm showed 23 bass from Lake Monticello with the following genetic group distributions: 1 with low levels of FB alleles, 4 with moderate levels, 5 with high levels, and 13 FB.
Discussion
Effectiveness of stocking regimens
Mean admixture proportions and the genetic group distributions were consistent with AGFC stocking histories (). The occurrence of NLMB in newly constructed reservoirs stocked with FB (e.g., Lake Monticello and Lake Columbia) is common (Gilliland and Whitaker Citation1989, Forshage and Fries Citation1995, Hughes and Wood Citation1995, Allen and Johnson Citation2009). Because Lake Columbia was inconsistently stocked on 2 occasions with NLMB fingerlings shortly following construction in the late 1980s, we expected NLMB alleles in bass of this reservoir to be common, although q-values were greater than expected. Lake Monticello, which had never been stocked with NLMB, did have bass with moderate levels of NLMB alleles, possibly due to historical contamination of the FB broodstock before vigorous genetic testing (Maceina et al. Citation1988, Gilliland and Whittaker Citation1989, Barthel et al. Citation2010), movement of native NLMB into reservoirs by feeder streams (Johnson and Fulton Citation1999, Allen et al. Citation2009), flooding of local streams following reservoir construction (Allen et al. Citation2009), reservoir inundation of existing NLMB ponds (Maceina et al. Citation1988, Allen et al. Citation2009), and/or fisherman transfer (Allen et al. Citation2009, Lamothe and Johnson Citation2013).
Despite the mixing of species within these reservoir populations, newly constructed reservoirs stocked with FB had a greater proportion of sampled FB than reservoirs that contained a resident NLMB population before FB introductions, consistent with previous studies (Gilliland and Whitaker Citation1989, Forshage and Fries Citation1995, Hughes and Wood Citation1995, Allen et al. Citation2009). Correlations of physical characteristics (age, surface area, latitude) or stocking characteristics in Arkansas reservoirs (number and density of fish stocked, number of stocking events, reservoir age at first stocking) showed no relationships with stocking success as measured by q-values (unpublished data). The stocking of FB had little effect on mean admixture proportions in mature reservoirs with preexistent NLMB populations, and therefore the results do not support stocking FB as an effective way to increase FB allele frequencies.
Performance among largemouth bass genetic groups
Similar to previous studies on relative condition of NLMB and FB (Isely et al. Citation1987, Maceina and Murphy Citation1988, Kleinsasser et al. Citation1990, Horton and Gilliland Citation1993), differences in size among the species and their hybrids in Arkansas reservoirs are not clear-cut. Reservoirs stocked with FB make poor laboratories due to a lack of initial experimental design, leading to large discrepancies in numbers among representative genetic groups. Furthermore, a high number of physical and biological variables interact in reservoir systems, adding additional layers to this complex management approach.
Nonetheless, several reservoir bass populations showed differences in size structure among genetic groups. In Lake Monticello where FB were plentiful, FB were longer and older than fish containing NLMB alleles. In reservoirs where FB were infrequent, there were no length or weight differences among genetic groups. Perhaps the low sample sizes of FB and the bias of hybrid fish having q-values approaching the 0.95 threshold reduced the power of analyses in those reservoir populations. Further, we found no significant differences in the slopes of within reservoir weight × length regressions, indicating a lack of difference in overall size measurements; these findings were consistent with bass populations in South Carolina (Leitner et al. Citation2002). Among female bass from Lake Monticello, the data suggest lower length and weight potentials of later generational backcrossed bass, consistent with findings of Philipp et al. (Citation2002). Additional data could help to evaluate this potential trend of outbreeding depression.
The greater length of FB in Lake Monticello is consistent with trophy bass programs in the southern United States, which have shown a predominance of FB and/or F1-hybrids among larger fish (Forshage et al. Citation1989, Horton and Gilliland Citation1993, Forshage and Fries Citation1995, Hughes and Wood Citation1995, Lutz-Carrillo et al. Citation2006, Tibbs Citation2008, Lamothe and Johnson Citation2013). Additionally, there has been an increase in the frequency of state record bass caught in several southern states following the stocking of FB (Horton and Gilliland Citation1993, Forshage and Fries Citation1995, Hughes and Wood Citation1995). In 2012, the AGFC had to disqualify what would have been the state record largemouth bass (7.4 kg), a record held for 37 years, due to a lack of angler licensure. This bass was genetically confirmed using microsatellite analysis to be an FB in a reservoir not previously stocked with FB but was within 2 km of a reservoir that had been stocked with FB (Lamothe and Johnson Citation2013).
The greater age of FB associated with their greater lengths and weights in Lake Monticello is consistent with Crawford et al. (Citation2002), who showed that longevity is a critical factor for trophy largemouth bass production in Florida lakes. As largemouth bass continue to grow throughout their lifespan, the additional years of growth for FB, particularly females, may allow them to become larger than NLMB or their hybrids. In a simultaneous study of existing and proposed slot limits on bass size structure in Lake Monticello (Risley and Johnson forthcoming), 10 of the 70 fish collected from the existing slot (406–533 mm) were age 13 and represented the original stocked FB cohort. Sampling 4 years later showed 1 male bass to be age 17 and also part of the original cohort.
The question remains whether differences in maximum age among species are due to susceptibility to anglers, heritable longevity, disease resistance, or predation. In contrast to earlier pond studies that were inconclusive in demonstrating differences in angling susceptibility (Zolczynski and Davies Citation1976, Inman et al. Citation1978, Wright and Wigtil Citation1980, Kleinsasser et al. Citation1990), it has been demonstrated in both ponds and reservoirs that FB are less susceptible to angling than are NLMB (Garrett Citation2002, Lutz-Carrillo and Dumont Citation2012). A selective removal, and therefore increased mortality of NLMB, by angling would result in increases in FB frequency with increasing fish age. Nonetheless, it would be reasonable to predict that these larger bass would be more difficult to harvest by anglers.
Although angler selection and harvest may explain some of the longevity differences among genetic groups, creel surveys comparing harvest relative to actual genetic structure has not been used to test this hypothesis for Arkansas reservoir bass populations. However, creel and mail-in surveys of Lake Monticello anglers revealed that 95% of all largemouth bass caught were released, and of those caught that could be harvested (slot limit of 406–533 mm), 88% were voluntarily released (Risley and Johnson forthcoming). This high voluntary release rate has become a national trend among largemouth bass anglers (Allen et al. Citation2008, Myers et al. Citation2008), which would reduce direct harvest mortality associated with genetic structure. Nonetheless, indirect effects of angling could include interference with reproductive success, post-catch mortality, or reduced feeding, and therefore present a secondary effect on genetic structure of mature bass (Gustaveson et al. Citation1991, Muoneke and Childress Citation1994, Philipp et al. Citation1997, Suski and Philipp Citation2004, Philipp et al. Citation2015).
Other factors have been proposed for differing survival of NLMB and FB. For example, genetic groups may have differential mortalities associated with stress, disease, and predation, although these possibilities have been poorly studied. Both Wright and Wigtil (Citation1980) and Maceina et al. (Citation1988) found lowered mortalities for F1-hybrids versus FB, and greater mortality for NLMB in Oklahoma ponds and a Texas reservoir, respectively. In contrast, Kleinsasser et al. (Citation1990) found no differences in mortality among phenotypes in Texas ponds. Sudden temperature shocks have been identified as causing mortality of FB during the winter in Illinois ponds (Philipp and Whitt Citation1991), although the thermal regimes of Illinois and southern Arkansas differ greatly. Outbreeding depression at both population and species levels has been observed in more northern latitudes (Philipp and Claussen Citation1995, Philipp et al. Citation2002) and in laboratory settings (Cooke et al. Citation2001, Goldberg et al. Citation2005, Cooke and Philipp Citation2006). More research into the observed differences found in female bass among genetic groups in Lake Monticello may help to evaluate the likelihood of outbreeding depression.
Management implications
Central Arkansas might represent the northern limits of temperature ranges suitable for differential FB growth. The AGFC has historically used heating degree days (3400; Gilliland Citation1992) to establish northern boundaries of FB stocking limits but presently uses a minimum water temperature of 4–5 C (Gilliland G, Oklahoma Department of Conservation, pers. comm.). This thermal limit runs centrally through the state, north of Little Rock (), and therefore the AGFC does not stock northern Arkansas lakes with FB (one exception being SWEPCO Lake, a discharge reservoir from the Flint Creek Power Plant).
From a fisheries management perspective, stocking FB into nonnative waters may present a conflict between overall bass size structure and catchability. The differential representation of FB among larger, older fish in Lake Monticello may lead to an increase in trophy catches, although due to their lower catchability, they may not be proportional to their population abundance. Establishment of creel surveys by the AGFC with a genetic component would provide insight into this key question. Despite this caveat, the opportunity to catch trophy FB has been shown to increase fishing activity in stocked lakes, impacting economies on a local level and resulting in a greater number of fishing licenses sold, therefore promoting the ongoing stocking of FB by game and fish agencies (Chen et al. Citation2003). In fact, most anglers fish Lake Monticello for the purpose of catching a trophy bass (Risley and Johnson forthcoming). As we have shown, in several reservoirs FB were poorly represented among bass in reservoirs stocked for more than 20 years, and therefore the potential benefits of stocking FB may not be observed.
The stocking of nonnative fish is controversial given the value of maintaining the genetic integrity of local fish populations (Philipp Citation1991, Olver et al. Citation1995, Cooke et al. Citation2001, Philipp et al. Citation2002, Barthel et al. Citation2010, Porak et al. Citation2015) and the potential for outbreeding depression of mixed stocks (Philipp Citation1991, Philipp et al. Citation2002, Edmands Citation2007). Ray et al. (Citation2012) showed that FB haplotypes can reach habitats far from the managed reservoirs in Texas where they were introduced (80 km upstream). The long-term effects of stocking nonnative bass may offset the short-term benefits to serve a public community. Finding that balance of serving a constituency while developing long-term sustainable management strategies is critically important for public agencies in maintaining the viability of native aquatic ecosystems.
Acknowledgments
We thank C. Cato, K. Hopkins, S. Wooldridge, B. Hobbs, V. Rolland, and K. M. Somers for technical and statistical support. We also thank our anonymous reviewers for improving the quality of this manuscript.
Funding
The AGFC through the Federal Aid to Sport Fish Restoration under Project F-39-R funded this research.
References
- Allen R, Cato C, Dennis C, Johnson RL. 2009. Condition relative to phenotype for bass populations in southern Arkansas lakes. J Arkansas Acad Sci. 63:20–27.
- Allen R, Johnson RL. 2009. Temporal changes of largemouth bass alleles in a northern Arkansas lake stocked with Florida bass. J Arkansas Acad Sci. 63:28–33.
- Allen MS, Walters CJ, Myers R. 2008. Temporal trends in largemouth bass mortality, with fishery implications. N Am J Fish Manage. 28:418–427.
- Anderson MJ. 2001. A new method for non-parametric multivariate analysis of variance. Austral Ecol. 26:32–46.
- [AGFC[ Arkansas Game and Fish Commission. 2002. Arkansas largemouth bass management plan. Little Rock (AR).
- Barthel BL, Allen MS, Porak WF, Kerns J. 2015. Florida bass Micropterus floridanus (Lesueur, 1822). In: Tringali MD, Long JM, Birdsong TW, Allen MS, editors. Black bass diversity: multidisciplinary science for conservation. American Fisheries Society Symposium 82. Bethesda (MD): American Fisheries Society. p. 44–54.
- Barthel BL, Lutz-Carrillo DJ, Norberg KE, Porak WF, Tringali MD, Kassler TW, Johnson WE, Readel AM, Krause RA, Philipp DP. 2010. Genetic relationships among populations of Florida bass. T Am Fish Soc. 139:1615–1641.
- Benjamini Y, Hochberg Y. 1995. Controlling the false discovery rate: a practical and powerful approach to multiple testing. J Roy Stat Soc B Met. 57:289–300.
- Buckmeier DL, Howells RG. 2003. Validation of otoliths for estimating ages of largemouth bass to 16 years. N Am J Fish Manage. 23:590–593.
- Chen RJ, Hunt KM, Ditton RB. 2003. Estimating the economic impacts of a trophy largemouth bass fishery: issues and applications. N Am J Fish Manage. 23:835–844.
- Colbourne JK, Neff BD, Wright JM, Gross MR. 1996. DNA fingerprinting of bluegill sunfish (Lepomis macrochirus) using (GT)n microsatellites and its potential for assessment of mating success. Can J Fish Aquat Sci. 53:342–349.
- Cooke SJ, Kassler TW, Philipp DP. 2001. Physiological performance of largemouth bass related to local adaptation and interstock hybridization: implications for conservation and management. J Fish Biol. 59:248–268.
- Cooke SJ, Philipp DP. 2006. Hybridization among divergent stocks of largemouth bass (Micropterus salmoides) results in altered cardiovascular performance: the influence of genetic and geographic distance. Physiol Biochem Zool. 79:400–410.
- Crawford S, Porak WF, Renfro DJ, Cailteux RL. 2002. Characteristics of trophy largemouth bass populations. In: Philipp DP, Ridgway MS, editors. Black bass: ecology, conservation and management. American Fisheries Society Symposium 31. Bethesda (MD): American Fisheries Society. p. 567–582.
- DeWoody JA, Fletcher DE, Wilkins SD, Nelson WS, Avise JC. 2000. Genetic monogamy and biparental care in an externally fertilizing fish, the largemouth bass (Micropterus salmoides). Proc R Soc Lond B. 267:2431–2437.
- Dotson JR, Allen MS, Kerns JA, Pouder WF. 2013. Utility of restrictive harvest regulations for trophy largemouth bass management. N Am J Fish Manage. 33:499–507.
- Edmands S. 2007. Between a rock and a hard place: evaluating the relative risks of inbreeding and outbreeding for conservation and management. Mol Ecol. 16:463–475.
- Forshage AA, Durocher PP, Webb MA, Lewis DG. 1989. Management application of angler recognition program data. Proceedings of the Annual Conference of the Southeastern Association of Fish and Wildlife Agencies. 43:182–190.
- Forshage AA, Fries LT. 1995. Evaluation of the Florida largemouth bass in Texas, 1972–1993. In: Schramm HL Jr, Piper RG, editors. Uses and effects of cultured fishes in aquatic ecosystems. American Fisheries Society Symposium 15. Lawrence (KS): Allen Press. p. 484–491.
- Gabelhouse DW Jr. 1984. A length categorization system to assess fish stocks. N Am J Fish Manage. 4:273–285.
- Garrett GP. 2002. Behavioral modification of angling vulnerability in largemouth bass through selective breeding. In: Philipp DP, Ridgway MS, editors. Black bass: ecology, conservation and management. American Fisheries Society Symposium 31. Bethesda (MD): American Fisheries Society. p. 387–392.
- Gilliland ER. 1992. Experimental stocking of Florida largemouth bass into small Oklahoma reservoirs. Proceedings of the Annual Conference of the Southeastern Association of Fish and Wildlife Agencies. 46:487–494.
- Gilliland ER, Whitaker J. 1989. Introgression of Florida largemouth bass introduced into northern largemouth bass populations in Oklahoma reservoirs. Proceedings of the Annual Conference of the Southeastern Association of Fish and Wildlife Agencies. 43:182–190.
- Goldberg TL, Grant EC, Inendino KR, Kassler TW, Claussen JE, Philipp DP. 2005. Increased infectious disease susceptibility resulting from outbreeding depression. Conserv Biol. 19:455–462.
- Gustaveson AW, Wydoski RS, Wedemeyer GA. 1991. Physiological response of largemouth bass to angling stress. T Am Fish Soc. 120:629–636.
- Hervé M. 2014. RVAideMemoire: diverse basic statistical and graphical functions. R package version 0.9-45-2; [cited 18 May 2015]. Available from: http://CRAN.R-project.org/package=RVAideMemoire
- Horton RA, Gilliland ER. 1993. Monitoring trophy largemouth bass in Oklahoma using a taxidermist network. Proceedings of the Annual Conference of the Southeastern Association of Fish and Wildlife Agencies. 47:679–685.
- Hughes JS, Wood MG. 1995. Development of a trophy largemouth bass fishery in Louisiana. Proceedings of the Annual Conference of the Southeastern Association of Fish and Wildlife Agencies. 49:58–68.
- Inman CR, Dewey RC, Durocher PP. 1978. Growth comparisons and catchability of three largemouth bass strains. Proceedings of the Annual Conference of the Southeastern Association of Fish and Wildlife Agencies. 30:40–47.
- Isely JJ, Noble RL, Koppelman JB, Philipp DP. 1987. Spawning period and first-year growth of northern, Florida, and intergrade stocks of largemouth bass. T Am Fish Soc. 116:757–762.
- Johnson RL, Fulton T. 1999. Persistence of Florida largemouth bass alleles in a northern Arkansas population of largemouth bass, Micropterus salmoides Lacèpéde. Ecol Freshw Fish. 8:35–42.
- Kassler TW, Koppelman JB, Near TJ, Dillman CB, Levengood JM, Swofford DL, Van Orman JL, Claussen JC, Philipp DP. 2002. Molecular and morphological analyses of the black basses: implications for taxonomy and conservation. In: Philipp DP, Ridgway MS, editors. Black bass: ecology, conservation and management. American Fisheries Society Symposium 31. Bethesda (MD): American Fisheries Society. p. 291–322.
- Kleinsasser LJ, Williamson JH, Whiteside BG. 1990. Growth and catchability of northern, Florida, and F1 hybrid largemouth bass in Texas ponds. N Am J Fish Manage. 10:462–468.
- Lamothe KA, Allen RM, Cato C, Winningham K, Dennis C, Johnson RL. 2012. Shifting genetic composition of largemouth bass populations in dendritic arms of two large Arkansas reservoirs through stocking of Florida largemouth bass. Proceedings of the Annual Conference of the Southeastern Association of Fish and Wildlife Agencies. 66:82–87.
- Lamothe KA, Johnson RL. 2013. Microsatellite analysis of trophy largemouth bass from Arkansas reservoirs. J Arkansas Acad Sci. 67:71–80.
- Lee DS, Gilbert CR, Hocutt CH, Jenkins RE, McAllister DE, Stauffer JR Jr. 1980. Atlas of North American freshwater fishes. Raleigh (NC): North Carolina State Museum of Natural History.
- Leitner J, Bulak J, Dunham R. 2002. A comparison of first and third year growth of two strains of largemouth bass in South Carolina. In: Philipp DP, Ridgway MS, editors. Black bass: ecology, conservation and management. American Fisheries Society Symposium 31. Bethesda (MD): American Fisheries Society. p. 365–370.
- Lutz-Carrillo DJ, Dumont S. 2012. Subspecies composition of angled and electrofished largemouth bass in Texas Reservoirs. Proceedings of the Annual Conference of the Southeastern Association of Fish and Wildlife Agencies. 66:75–81.
- Lutz-Carrillo DJ, Nice CC, Bonner TH, Forstner MRJ, Fries LT. 2006. Admixture analysis of Florida largemouth bass and northern largemouth using microsatellite loci. T Am Fish Soc. 135:779–791.
- Maceina MJ, Murphy BR. 1988. Variation in the weight-to-length relationship among Florida and northern largemouth bass and their intraspecific F1 hybrid. T Am Fish Soc. 117:232–237.
- Maceina MJ, Murphy BR, Isely JJ. 1988. Factors regulating Florida largemouth bass stocking success and hybridization with northern largemouth bass in Aquilla Lake, Texas. T Am Fish Soc. 117:221–231.
- Malloy TP, van den Bussche RA, Coughlin WD, Echelle AA. 2000. Isolation and characterization of microsatellite loci in smallmouth bass, Micropterus dolomieu (Teleostei: Centrarchidae), and cross-species amplification in spotted bass, M. punctulatus. Mol Ecol. 9:1946–1948.
- Manly BFJ. 2007. Randomization, bootstrap and Monte Carlo methods in biology. 3rd ed. New York (NY): Chapman and Hall/CRC.
- Muoneke MI, Childress WM. 1994. Hooking mortality: a review for recreational fisheries. Rev Fish Sci. 2:123–156.
- Myers R, Taylor J, Allen M, Bonvechio TF. 2008. Temporal trends in voluntary release of largemouth bass. N Am J Fish Manage. 28:428–433.
- Near TJ, Bolnick DI, Wainwright PC. 2004. Investigating phylogenetic relationships of sunfishes and black basses (Actinopterygii: Centrarchidae) using DNA sequences from mitochondrial and nuclear genes. Mol Phylogenet Evol. 32:344–357.
- Near TJ, Kassler TW, Koppelman JB, Dillman CB, Philipp DP. 2003. Speciation in North American black basses, Micropterus (Actinopterygii: Centrarchidae). Evolution. 57:1610–1621.
- Oksanen J, Blanchet FG, Kindt R, Legendre P, Minchin PR, O'Hara RB, Simpson GL, Solymos P, Stevens MHH, Wagner H. 2015. vegan: Community Ecology Package. R package version 2.2-1; [cited 18 May 2015]. Available from: http://cran.r-project.org/web/packages/vegan/index.html
- Olver CH, Shuter BJ, Minns CK. 1995. Toward a definition of conservation principles for fisheries management. Can J Fish Aquat Sci. 52:1584–1594.
- Philipp DP. 1991. Genetic implications of introducing Florida largemouth bass, Micropterus salmoides floridanus. Can J Fish Aquat Sci. 48:58–65.
- Philipp DP, Childers WF, Whitt GS. 1983. A biochemical genetic evaluation of the northern and Florida subspecies of largemouth bass. T Am Fish Soc. 112:1–20.
- Philipp DP, Claussen JE. 1995. Fitness and performance differences between two stocks of largemouth bass from different river drainages within Illinois. In: Schramm HL Jr, Piper RG, editors. Uses and effects of cultured fishes in aquatic ecosystems. American Fisheries Society Symposium 15. Lawrence (KS): Allen Press. p. 236–243.
- Philipp DP, Claussen JE, Kassler TW, Epifanio JM. 2002. Mixing stocks of largemouth bass reduces fitness through outbreeding depression. In: Philipp DP, Ridgway MS, editors. Black bass: ecology, conservation and management. American Fisheries Society Symposium 31. Bethesda (MD): American Fisheries Society. p. 349–363.
- Philipp DP, Claussen JE, Koppelman JB, Stein JA, Cooke SJ, Suski CD, Wahl DH, Sutter DAH, Arlinghaus R. 2015. Fisheries-induced evolution in largemouth bass: linking vulnerability to angling, parental care, and fitness. In: Tringali MD, Long JM, Birdsong TW, Allen MS, editors. Black bass diversity: multidisciplinary science for conservation. American Fisheries Society Symposium 82. Bethesda (MD): American Fisheries Society. p. 223–236.
- Philipp DP, Toline CA, Kubacki MF, Philipp DBF, Phelan FJS. 1997. The impact of catch-and-release angling on the reproductive success of smallmouth bass and largemouth bass. N Am J Fish Manage. 17:557–567.
- Philipp DP, Whitt GS. 1991. Survival and growth of northern, Florida, and reciprocal Fl hybrid largemouth bass in central Illinois. T Am Fish Soc. 120:58–64.
- Porak WF, Barthel BL, Stout RB, Tringali MD, Matthews M, Pouder WF, Philipp DP. 2015. The development of a genetic conservation program for the Florida bass in its native range. In: Tringali MD, Long JM, Birdsong TW, Allen MS, editors. Black bass diversity: multidisciplinary science for conservation. American Fisheries Society Symposium 82. Bethesda (MD): American Fisheries Society. p. 585–600.
- Pritchard JK, Stephens M, Donnelly P. 2000. Inference of population structure using multilocus genotype data. Genetics. 155:945–959.
- R Core Team. 2015. R: A language and environment for statistical computing. R Foundation for Statistical Computing. Vienna, Austria; [cited 18 May 2015]. Available from: http://www.R-project.org/
- Ray JW, Husemann M, King RS, Danley PD. 2012. Genetic analysis reveals dispersal of Florida bass haplotypes from reservoirs to rivers in Central Texas. T Am Fish Soc. 141:1269–1273.
- Risley JT, Johnson RL, Forthcoming 2015. Catch and release impacts trophy bass fishery in Arkansas. Proceedings of the Annual Conference of the Southeastern Association of Fish and Wildlife Agencies. 69.
- Schwartz TS, Beheregaray LB. 2008. Using genotype simulations and Bayesian analyses to identify individuals of hybrid origin in Australian bass: lessons for fisheries management. J Fish Biol. 72:435–450.
- Seyoum S, Barthel BL, Tringali MD, Davis MC, Schmitt SL, Bellotti PS, Porak WF. 2013. Isolation and characterization of eighteen microsatellite loci for the largemouth bass, Micropterus salmoides, and cross amplification in congeneric species. Conserv Genet Resour. 5:697–701.
- Siepker MJ, Casto-Yerty M. 2008. A survey of fishery agency supplementary largemouth bass stocking practices in large United States reservoirs. Am Fish S. 62:441–451.
- Suski CD, Philipp DP. 2004. Factors affecting the vulnerability to angling of nesting male largemouth and smallmouth bass. T Am Fish Soc. 133:1100–1106.
- Tibbs JE. 2008. Factors related to the genetic composition and fishing quality of largemouth bass fisheries in Texas reservoirs. Proceedings of the Annual Conference of the Southeastern Association of Fish and Wildlife Agencies. 62:104–108.
- Wilson DM, Dicenzo VJ. 2002. Profile of a trophy largemouth bass fishery in Briery Creek Lake, Virginia. In: Philipp DP, Ridgway MS, editors. Black bass: ecology, conservation and management. American Fisheries Society Symposium 31. Bethesda (MD): American Fisheries Society. p. 583–592.
- Wright GL, Wigtil GW. 1980. Comparison of growth, survival, and catchability of Florida, northern, and hybrid largemouth bass in a new Oklahoma reservoir. Proceedings of the Annual Conference of the Southeastern Association of Fish and Wildlife Agencies. 34:31–38.
- Zolczynski SJ Jr, Davies WD. 1976. Growth characteristics of the northern and Florida subspecies of largemouth bass and their hybrid, and a comparison of the catchability between the subspecies. T Am Fish Soc. 105:240–243.