ABSTRACT
Roberts CM, DeVries DR, Wright RA. 2018. Introduced yellow perch in two Southeastern US reservoirs: ecological interactions with resident fishes. Lake Reserv Manage. 34:141—153.
Yellow perch (Perca flavescens) has been introduced into Southeastern US drainages outside its native range; unfortunately, the effects of these introductions at the edge of their distribution on resident fishes have not been well documented. To determine the potential interactions between introduced yellow perch and resident fishes, we quantified diets of multiple life stages over 2 yr in 2 Alabama reservoirs. Temporal and spatial co-occurrence between larval yellow perch and native fishes was limited, and diet overlap was generally low at all life stages. Juvenile yellow perch were consumed by Micropterus spp. in both lakes during spring, suggesting some potential benefit for native piscivores. We suggest minimal negative effects of yellow perch on native fishes in these Southeastern US systems due to their generalist diet, early spawning period, and cool water temperature requirements. In addition, yellow perch may represent an alternative prey resource for piscivores.
KEYWORDS:
Nonnative fish introductions have impacted systems throughout the United States (Kitchell Citation1990, Fuller et al. Citation1999, Brooks and Jordan Citation2009), causing declines in distribution and abundance and even extinction of native fishes (Strayer Citation2010). Yellow perch (Perca flavescens) is a North American fish species native to the upper Midwest and Atlantic coast. Its range has been expanded by both unintentional and intentional introductions into the southeastern United States (Timmons Citation1975, Clugston et al. Citation1978). These introductions include the Tennessee and Chattahoochee rivers (Hackney and Holbrook Citation1978), and recently the Tallapoosa River in Alabama (Hornsby J, Alabama Department of Conservation and Natural Resources, pers. comm.), at or near the edge of the species' thermal tolerance (see Hokanson Citation1977 and Feiner and Höök Citation2015 for information on upper temperature limits).
Yellow perch is an opportunistic feeder, eating almost any appropriately sized animal prey (Knight et al. Citation2011), including small zooplankton as prolarvae (Ney Citation1978) and larger zooplankton as juveniles (Pelham et al. Citation2001). In general, after reaching ∼35 mm, yellow perch diets shift from planktivory to insectivory, and at ∼80 mm from insectivory to piscivory (Parker et al. Citation2009), although variation in these transitions clearly exists (see e.g., Feiner and Höök Citation2015). Adult yellow perch maintain a wide diet breadth, continuing to feed on zooplankton and aquatic macroinvertebrates as well as feeding on fishes (e.g., bluegill [Lepomis macrochirus]; Fullhart et al. Citation2002).
Yellow perch potentially compete with other fishes both within their native range and in systems where the species has been introduced. Several studies have demonstrated or suggested competition between yellow perch and other fishes (Hayes et al. Citation1992, Shrader Citation2000, Browne and Rasmussen Citation2009). For example, Hayes et al. (Citation1992) found evidence of competition for benthic resources between yellow perch and white suckers (Catostomus commersonii) in a Michigan lake, and Browne and Rasmussen (Citation2009) found diet shifts in brook trout (Salvelinus fontinalis) in the presence vs. absence of yellow perch in Ontario lakes. However, these results are not universal, as a yellow perch introduction into West Point Reservoir, Alabama–Georgia, did not appear to have a strong negative impact on native adult largemouth bass (Micropterus salmoides) populations (Timmons Citation1984), although potential impacts on juvenile life-stages were not determined. Generally, the influence of introduced yellow perch on native fishes, particularly in southeastern reservoirs, has not been documented.
As a prey resource, yellow perch often is important for piscivores, which has implications for both predators and other prey fishes. For example, within its native range it is a favored prey of walleye (Sander vitreus; Chevalier Citation1973, Forney Citation1974, Rudstam et al. Citation1996) and can be important prey for northern pike (Esox lucius; Margenau et al. Citation1998). The strong linkage between yellow perch and walleye can cause cyclic patterns of strong and weak year classes and these fluctuations are reflected in the growth of both species (Rudstam et al. Citation1996). Yellow perch may also serve as a buffer reducing predation on other prey (e.g., walleye; Chevalier Citation1973, Forney Citation1974). Anecdotal information suggests that yellow perch may occur in diets of both Alabama bass (Micropterus henshalli) and striped bass (Morone saxatilis) in Lake Martin, Alabama (Paramore J, Lake Martin Fishing Guide, and Nichols N, Alabama Department of Conservation and Natural Resources, pers. comm.). As such, introduced yellow perch could provide an additional prey resource for piscivores.
Here we examined interactions between introduced yellow perch and native fishes in 2 Alabama reservoirs. We evaluate the potential for positive and/or negative effects of yellow perch on resident fishes specifically to quantify both the extent of diet overlap between yellow perch and resident fishes, as well as the role of yellow perch as prey for resident piscivores. Our expectation was that the introduction of nonnative yellow perch into these systems would have effects on early life stages of resident fishes (mediated through trophic interactions).
Sample area and locations
Sampling was conducted in 2 reservoirs on the Tallapoosa River, Alabama: Lake Martin and the immediate downstream system, Yates Lake (). Both of these reservoirs contained introduced yellow perch populations that date back to the 1990s (Alabama Department of Natural Resources, unpubl. data). Although adjacent to one another, the reservoirs differ in physical and chemical characteristics, with Lake Martin being larger (16,188 ha vs. 680 ha) and generally less productive. Yates Lake is considered mesotropic to eutrophic while Lake Martin is considered oligotrophic to mesotrophic (AWW Citation2005, Citation2012). Lake Martin is primarily a storage impoundment (mean depth = 12 m) with a long water retention time, resulting in seasonal stratification (AWW Citation2012). Yates Lake is a shallower run-of-the-river impoundment (mean depth = 8.3 m) used for hydroelectric generation with a short water retention period and ephemeral stratification (AWW Citation2012). We sampled 5 sites in Lake Martin (the Kowaliga arm [in both Parker Creek Bay and Chapman Creek Bay], the Blue Creek arm, and the main stem [Elkahatchee Creek Bay and Irwin Shoals]), and 3 study sites in Yates Lake (the island near the AL Hwy 50 Bridge, Chanahatchee Creek, and Saugahatchee Creek; ).
Methods
Zooplankton and chlorophyll a (Chl-a) samples
Zooplankton samples were collected at each site once per week during February through May, and once per month from May through August of both sampling years. We sampled zooplankton at each site with a 50 µm mesh net (31 cm diameter, 91 cm long) with 2 replicate vertical tows through the photic zone (approximately twice the Secchi depth) or from the bottom, whichever was shallower. Samples were preserved in 70% ethanol and returned to the lab. Water samples for Chl-a analysis were collected from just beneath the surface at each site during each sampling trip. Samples were collected in 500 mL dark polyethylene bottles, which were then placed directly on ice and returned to the lab.
Larval and juvenile fish collection
Larval fish and early juvenile fish (≤ approximately 25 mm TL) that remained pelagic were sampled with a push net and a neuston net. We collected 2 replicate 5 min pushes with the push net (50 cm diameter, 150 cm long, 500 µm mesh, 1–1.5 m/sec with an attached flow meter to allow calculation of sample speed and volume of water sampled). In 2010, once larval fish were estimated to have reached 10–13 mm, a neuston net (2 m × 1 m opening, 399 cm deep, 1 mm mesh, 8–10 min tow, 1–1.5 m/sec) was used. After evaluating catches from both gear types in 2010, we determined that the push net collected larger larvae at a similar rate to the neuston net so only push net samples were collected in 2011. Larval fish sampling occurred once per week at each site from February through May, and once per month from June through August in both years.
As yellow perch grew out of the larval sizes and began to shift in their habitat use (as per Post and McQueen Citation1988), we changed the sampling gears used to collect them. After testing a variety of sampling gears to collect juvenile yellow perch (unbaited minnow traps, light traps, a beam trawl, small mesh [∼ 8 mm mesh] gill nets, boat electrofishing, prod pole electrofishing), we found night-time pulsed-DC boat electrofishing to be most effective. All juvenile yellow perch, black basses (Micropterus spp.), and crappies (Pomoxis spp.) captured were placed on ice and returned to the lab.
Adult fish collection
Adult fish were sampled from February through April of both 2010 and 2011 using pulsed-DC electrofishing at night (Smith Root 7.5 GPP) to ensure capture of prespawn, spawning, and postspawn adult yellow perch. One transect (10–30 min/transect) was electrofished at each site once per week. Adult bluegill, redear sunfish (Lepomis microlophus), striped bass, Alabama bass, largemouth bass, crappie (Pomoxis spp.), and yellow perch were collected, placed on ice and returned to the lab. In 2011, we extended weekly electrofishing to continue into May through July, with a sampling effort of 2 transects (10–30 min) at each of 2 sites within both Lake Martin (Parker, Chapman) and Yates Lake (Island, Channahatchee), and we collected adult yellow perch, largemouth bass, Alabama bass, and striped bass. This sampling focused on yellow perch and native piscivores to quantify potential predator–prey interactions.
Laboratory processing and analysis
To estimate zooplankton composition and density (No./L), the first 10 individuals encountered within each taxon were measured (body length). Subsamples were counted until at least 200 individuals of the most abundant taxa were counted (and the result extrapolated to the entire sample) or until the entire sample was counted (as in Dettmers and Stein Citation1992, Welker et al. Citation1994). Chl-a concentration (µg/L) was measured using standard fluorometry (Turner Designs Aquafluor).
Larval and juvenile fish were identified to genus, measured, and counted. We quantified diets of larval yellow perch, larval Pomoxis, larval Dorosoma, and larval Lepomis because of their potential co-occurrence in the pelagic zone. We analyzed diets of juvenile yellow perch and juvenile black basses given their spatial and temporal overlap in the littoral zone. Diets of up to 10 larval and 10 juvenile fish were quantified for each taxon per site per date, and prey items (up to 10 per taxon per fish) were measured (nearest 0.1 mm) under a dissecting microscope with an ocular micrometer.
All adult yellow perch collected from electrofishing were placed immediately on ice at the end of each transect, returned to the lab, weighed (nearest g), and measured (nearest mm TL). Stomachs from a minimum of 25 yellow perch were systematically collected from across the size range from each site for diet analysis. All bluegill, redear sunfish, black crappie, largemouth bass, Alabama bass, and striped bass were individually weighed and measured. Stomachs were collected from 10 individuals of each species per site per sample date for diet analyses.
Diet contents were quantified using a dissecting microscope; fish were identified to genus (species when possible), aquatic insects to family, and all other non-zooplankton invertebrates to order. Total length of fish in the diets was measured when possible; if a prey fish was too digested, then otoliths were identified (based on species-specific otolith structure, unpubl. data), counted, and measured, and fish length estimated from system-specific otolith size–fish size regressions. For invertebrates, the first 10 head capsules were measured for each family/order and the rest were counted. All zooplankton were identified to genus (for cladocerans) or as calanoids, cyclopoids, or nauplii (for copepods) in both field samples and diets. The first 10 individuals encountered from each taxon were measured (body length) and the remaining individuals of that taxon were counted.
Prey biomass was estimated from prey length measurements using prey-specific length–weight regressions (cited in Glover et al. Citation2012, Roberts Citation2012). These biomass estimates were then used to calculate weight-based diet proportions for each prey type for each individual fish. For larval fish diet analysis, prey item proportions were compared by number, given the general similarity in size among prey items. To evaluate the potential for competition between species, we used Schoener's overlap index (Schoener Citation1970) to calculate diet overlap between yellow perch and the other sport fish species collected (i.e., crappie, black bass, bluegill) at larval, juvenile, and adult life stages. If overlap between 2 species exceeded 0.5 (index ranges from 0 to 1) at the larval life stage, prey selection by larval fish was estimated using Chesson's alpha (Chesson Citation1978, 1983) to allow comparison between species. This analysis was run for each prey type for each individual fish, and means were calculated for each prey type across individuals within each fish species. We restricted this analysis to larval fish because their diets contained only zooplankton; this allowed calculation of prey availability, which could not be done for older fish whose diets contained larger macroinvertebrates and even fish, which were not captured in our prey availability samples.
Zooplankton density, Chl-a concentrations, and electrofishing catch rates (No./electrofishing hr) of fishes, all were compared between reservoirs and years using repeated-measures mixed model analysis of variance (PROC MIXED; SAS Institute Citation2012). Auto-regressive covariance structure (AR1) appropriate for repeated measures analysis (Kincaid Citation2005) and Kenward–Roger degrees of freedom approximation, which provides greater accuracy in parameter estimation in mixed-model repeated measures ANOVA with unbalanced designs (Padilla and Algina Citation2007), were chosen for these analyses.
Results
Chlorophyll and zooplankton abundance
Chl-a concentration and zooplankton abundance did not differ significantly between Lake Martin and Yates Lake; however, both measures differed between years. In both reservoirs, Chl-a concentrations were significantly higher in 2011 (df = 1,38, F = 19.16, P < 0.0001) and average zooplankton density was greater in 2011 (df = 1,176, F = 4.47, P = 0.036).
Electrofishing catch rates
Overall average spring electrofishing CPE (catch/hr) of yellow perch was significantly higher in 2010 than 2011 and higher in Yates Lake than in Lake Martin (year effect: df = 1,44.3, F = 4.46, P = 0.04; reservoir effect: df = 1,32.3, F = 8.83, P = 0.0056). The average CPE in Yates Lake was 52.8 (SE = 18.3) in 2010 and 29.5 (SE = 6.1) in 2011. The average CPE in Lake Martin was 17.4 (SE = 18.4) in 2010 and 2.6 (SE = 1.3) in 2011. Electrofishing CPE for black crappie, largemouth bass, and Alabama bass did not differ between lakes.
Larval fishes
Average larval yellow perch density was less in Lake Martin (2010: 0.0028 fish/m3; 2011: 0.07 fish/m3) than in Yates Lake (2010: 0.028 fish/m3; 2011: 0.61 fish/m3); larvae of 7 fish taxa were collected with yellow perch, Pomoxis, Lepomis, and Dorosoma being most abundant in both years (). There was little temporal overlap of larval yellow perch with larvae of other fish species in the limnetic zone in either lake (co-occurrence was on only 0–3 sample dates and these occurred when larval yellow perch densities were very low). Although this lack of temporal overlap does not address the potential for exploitative competition, peak zooplankton densities typically occurred after larval yellow perch were present (cf. larval yellow perch peaks in abundance in with zooplankton abundances in ).
Figure 2. Average (± 1 SE) larval fish density (individuals/m3) from push net/neuston net samples plotted through time in Lake Martin and Yates Lake during the 2010 and 2011 sampling season.
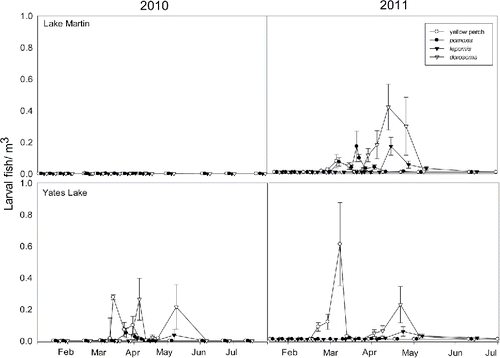
Figure 3. Average (± 1 SE) zooplankton density (individuals/L) plotted through time in Lake Martin and Yates Lake during the 2010 and 2011 sampling season.
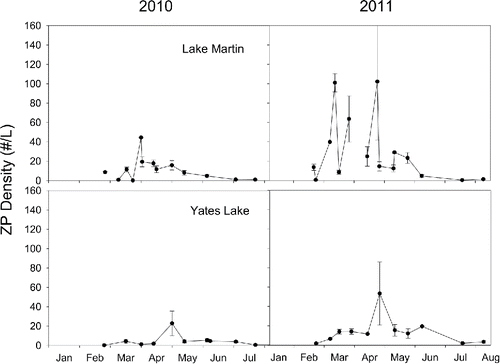
Diet overlap was consistently highest between larval Pomoxis and larval yellow perch (based on numbers; overlap values are in , diet proportions are in ). However, larval yellow perch in Lake Martin and Yates Lake generally positively selected for calanoid copepods (Chesson's alpha, range: 0.93–0.95), while larval Pomoxis positively selected for Bosmina (0.2–0.68; Roberts Citation2012). Calanoid copepods were also an important diet component of larval Dorosoma (), but seasonal co-occurrence of larval yellow perch and larval Dorosoma was minimal and occurred only when one or the other was at extremely low density. Diet overlap between larval Dorosoma and larval yellow perch could not be estimated reliably on about half the sampling dates due to low densities (less than 5 individual larvae across all collections on that date for a reservoir); otherwise, overlap values were variable in both reservoirs, ranging from 0.18 to 0.86 (). Larval Lepomis only co-occurred with larval yellow perch on one sample date (6 May 2010) and neutrally or negatively selected all zooplankton taxa. Positive selection for the same prey type, calanoid copepods, occurred on only 2 dates: on 30 April 2010 by larval yellow perch and Dorosoma in Yates Lake and on 28 March 2011 by larval yellow perch and larval Pomoxis (Roberts Citation2012).
Table 1. Schoener's diet overlap index values for larval yellow perch with larval Pomoxis, Lepomis, and Dorosoma (based on numbers), for juvenile yellow perch with Alabama bass and largemouth bass, and for adult yellow perch with bluegill, redear, black crappie, black bass, Alabama bass, and largemouth bass (based on biomass) collected from Lake Martin and Yates Lake. n/a = less than 3 individuals of one or both species were collected.
Juvenile fish diets
Too few juvenile yellow perch were collected in 2010 to allow for diet analyses. In 2011, we quantified diets of juvenile yellow perch, largemouth bass, and Alabama bass (≥20 diets per species were considered sufficient for analysis). Juvenile yellow perch first appeared in boat electrofishing samples in early May in both lakes and were regularly collected into June. Diets of juvenile yellow perch contained several prey types, with zooplankton contributing the greatest proportion (by biomass) in both lakes (). Largemouth bass diets in both lakes were similarly diverse with zooplankton contributing the largest proportions, while Alabama bass consumed primarily native fishes (). Diet overlap values between juvenile yellow perch and largemouth bass were 0.48 in Lake Martin and 0.55 in Yates Lake; overlap between yellow perch and Alabama bass in Yates Lake was 0.17. Insufficient numbers of juvenile Alabama bass were collected in Lake Martin to estimate diet overlap.
Adult fish diets
Diet analysis was only conducted on adult fish collected by night electrofishing (with the exception of striped bass). Adult yellow perch diets contained diverse prey types, differing somewhat between years in Lake Martin, with zooplankton being most prevalent (49%) in 2010 and native fishes contributing the largest portion of the biomass (30%) in 2011 (). Bluegill diets also included zooplankton (38%) in 2010, and chironomids were the primary prey of bluegill (47%), black crappie (55%), and redear sunfish (68%) in 2011 (). Black bass diets contained primarily native fishes in both years (), and in 2011, yellow perch were found in high numbers in the diets of both largemouth bass and Alabama bass in a 3-week period during May in Lake Martin (). In Yates Lake, Chaoborus and chironomids were important diet components of adult yellow perch, while diets of adult bluegill, black crappie, and redear sunfish included chironomids and other macroinvertebrates (). Diet overlap between adult yellow perch and black basses (0.17) and redear sunfish (0.45) in Lake Martin was low, but was higher for bluegill (0.80) (). Diet overlap in Yates Lake was moderate between yellow perch and bluegill (0.57) and low with redear sunfish (0.30) and largemouth bass (0.45) ().
Figure 6. Proportion of diet by biomass for adult fishes and yellow perch collected during 2010 and 2011 in Lake Martin and Yates Lake. The number on top of each bar indicates the number of fish that had food items in their stomach. YP = yellow perch, BG = bluegill, RS = redear sunfish, BB = black basses, BC = black crappie, LB = largemouth bass, AB = Alabama bass.
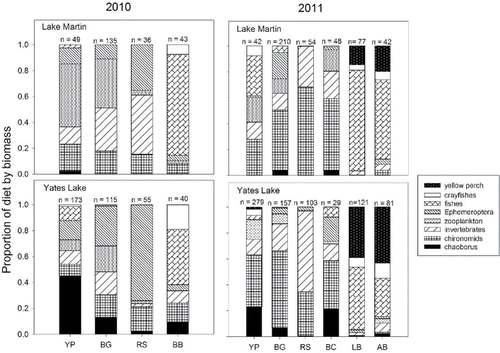
Figure 7. The proportion of diet biomass contributed by yellow perch in diets of Alabama bass and largemouth bass in both Lake Martin and Yates Lake during 2011.
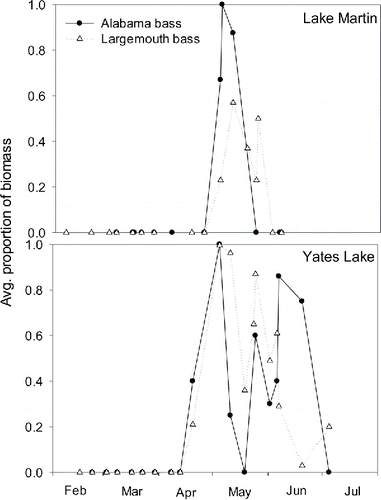
During the 2 sampling seasons, 16 adult striped bass were sampled and their diets collected in both Lake Martin and Yates Lake (these diets were analyzed from all gears due to the extremely low sample size). Of those fish sampled, diet biomass consisted of crayfish (31%), mayfly larvae (29%), shad (26%), and sunfish (14%). No yellow perch were found in diets of striped bass collected.
Discussion
Shea and Chesson (Citation2002) state 3 main factors that affect the potential success of an invading species: resources, natural enemies, and physical environment. First, food resources were likely available to yellow perch because of generally low diet overlap. Thus, the potential for competition between yellow perch and resident fishes appeared to be relatively low at all life stages. In addition, we saw no apparent reduction in zooplankton resources after yellow perch larvae and juveniles were present in these systems each spring, suggesting no depletion of zooplankton resources. For juvenile and adult fishes, overlap was either moderated by differential diet transitions and habitat use (yellow perch and largemouth bass juveniles) or a prey resource that was likely abundant and not limiting (for yellow perch, black crappie, and bluegill; see the next section). Second, yellow perch likely benefited from a lack of their natural enemies (e.g., diseases, parasites, predators [walleye]) in these Southeastern US systems. In contrast, we demonstrated that while generalist native predators (black basses) did prey on yellow perch, this only occurred within a short time window. In both systems, abundant juvenile yellow perch provided prey for some resident piscivores in the spring and early summer. This interaction may be one factor providing some degree of limitation to yellow perch populations in these reservoirs. The third factor was a difficult physical environment (in this case one that is warmer than in their native range), which may limit yellow perch success in these reservoirs. However, reservoirs may also create some circumstances (e.g., cool water hypolimnetic releases from Lake Martin Dam into Yates Lake) that allow for yellow perch success (in addition, basic population information supports that these populations were successfully sustaining themselves; Roberts Citation2012). Overall, our results suggest that reservoir systems may have allowed the establishment of yellow perch, but that these southern yellow perch populations have minimal negative effects on native fishes while providing some benefits. Below we consider these negative and positive effects more closely.
Potential negative impacts
Yellow perch hatched and their larvae occurred in the pelagic zone earlier in the spring than did larvae of native Pomoxis, Dorosoma, or Lepomis (see also Kaemingk et al. Citation2012). This timing generated minimal temporal overlap and, therefore, less opportunity at the larval life stage for diet overlap and direct competition between yellow perch larvae and larvae of native fishes. Certainly, by hatching prior to native species, yellow perch larvae could reduce zooplankton resources thereby reducing forage availability; however, zooplankton abundance did not differ with the presence versus absence of larval yellow perch and no apparent change in zooplankton abundance patterns through time were observed. A similar trend occurred with yellow perch larvae in lakes of the US upper Midwest (Schael et al. Citation1991, Jolley et al. Citation2010, Kaemingk et al. Citation2012). VanDeHey et al. (Citation2014) also found little evidence of negative interactions between larval yellow perch and larval gizzard shad (Dorosoma cepedianum) in natural lakes of southern South Dakota. In our study, co-occurrence occurred primarily between larval yellow perch and Pomoxis during April of both years in these systems. Analysis of prey selection showed that larval yellow perch primarily selected copepods (also seen in Graeb et al. Citation2004 and Jolley et al. Citation2010), while larval crappie had a more diverse diet. Therefore, all evidence suggested that the potential for competition between larval yellow perch and larvae of native fishes was minimal.
At the juvenile stage, yellow perch diets overlapped only moderately with those of largemouth bass and Alabama bass (all combined across dates). Yellow perch juveniles in the study reservoirs (as in their native range) primarily fed on zooplankton (Galbraith Citation1967, Hansen and Wahl Citation1981, Mills and Forney Citation1983, Graeb et al. Citation2004, Wallus Citation2006), with macroinvertebrates found in diets of juveniles >50 mm (Fisher and Willis Citation1997, Graeb et al. Citation2006). When co-occurring at sites sampled with yellow perch juveniles, black bass juveniles (<70 mm) had already transitioned to feed on fishes and various macroinvertebrates, as has been documented in several studies (Wicker and Johnson Citation1987, Hirst and DeVries Citation1994, Phillips et al. Citation1995). This difference in diet between species limited the amount of diet overlap occurring at the juvenile stage between yellow perch and black basses, and so reduced the potential for competitive interactions.
Interactions among adult yellow perch and resident species in these reservoirs appears to be limited as well. While there was high diet overlap among yellow perch, black crappie, and bluegill with the primary shared resource being chironomids, these midge larvae are often abundant in reservoirs (Cooper and Knight Citation1985) and are then eaten by these opportunistic feeding fish species. Ultimately, determining whether chironomid larvae actually are unlimited during the period of high diet overlap, or whether they might represent a limiting prey resource, would require additional experimental work beyond the scope of the current study. We also observed little direct predation by yellow perch on resident fishes.
An overall negative impact of the introduction of yellow perch into Lake Martin and Yates Lake on resident game fishes would result in reduced abundance in surveys and eventually in angler catches of the resident species as was observed in Phillips Reservoir in Oregon (Shrader Citation2000). While we cannot control for other potential changes in the environment, there is no such evidence of reduced abundance of resident game fishes. Tournament bass anglers reported increasing catches of largemouth bass in Lake Martin over the period since the yellow perch introduction (Abernethy Citation2014). Similarly, catch rate in electrofishing surveys in Lake Martin for sport fishes before vs. after yellow perch introduction through 2011 by Alabama Department of Conservation and Natural Resources have shown no evidence of decline (Lovell et al. Citation2011). Unfortunately, agency surveys of Yates Lake were not conducted routinely over this time period. Overall, however, there were no indications of negative impacts from the yellow perch introductions. Given that the maximum water temperature in both lakes reached upper limits for yellow perch in the summer (Hokanson Citation1977, Feiner and Höök Citation2015), yellow perch individuals had to locate refuge habitat to survive (likely deeper, colder water in Lake Martin, and the upper tailrace with hypolimnetic release in Yates Lake), possibly tempering negative effects that they might have had on native species. While such limitation would require additional research, it certainly would provide insight into how introduced populations at the edge of their range might interact with native fishes. Such insights will be increasingly important to lake and reservoir management as systems are altered due to climate change.
Potential positive impacts
The inclusion of juvenile yellow perch in the diets of largemouth bass and Alabama bass versus native fishes in 2011 suggests that yellow perch may represent an important source of prey for these piscivores. In our study, juvenile yellow perch were first observed in electrofishing samples during mid-May of 2011 (weekly inshore sampling during 2010 focused on adult yellow perch, and thus ceased in Apr when adults left the littoral zone), peaking in abundance at 412 fish/hr in Yates Lake and 36 fish/hr in Lake Martin. These seasonal peaks in abundance occurred as juvenile yellow perch recruited to the littoral zone. Yellow perch first appeared in black bass diets in late April 2011 and continued to be consumed through July. Purcell (Citation2011) had a similar observation, with Alabama bass diets containing a relatively high proportion of yellow perch despite their low occurrence in fish collections. Therefore, age-0 yellow perch appear to represent a seasonally important prey resource for black basses in both lakes. Previous studies have shown that juvenile yellow perch occupy the littoral zone (particularly at night) making them more vulnerable to predators (Kelso and Ward Citation1977, Ryder and Kerr Citation1978, Helfman Citation1981, Lyons Citation1987, Post and McQueen Citation1988).
Striped bass is another piscivore present in both reservoirs that could benefit from yellow perch as prey. Anecdotal information suggested that angled striped bass had primarily consumed threadfin shad (Dorosoma petenense) and gizzard shad (Parramore J, fishing guide for striped bass anglers, pers. comm.). Ruderhausen et al. (Citation2005) found age-1 striped bass in North Carolina to prey primarily on yellow perch during late spring; however, they did not contribute a high proportion of the diets of all age classes throughout the year. We also found that striped bass consumed primarily clupeids. Although thermal stratification of Lake Martin may create the potential for spatial overlap between yellow perch and striped bass and, therefore, increased opportunity for striped bass to consume yellow perch, we found no evidence to support that yellow perch was an important prey item for striped bass.
In general, yellow perch populations in both reservoirs do not appear to be having a broad negative impact on native fishes. This conclusion is based on evidence that temporal/spatial (for larval fishes) and diet overlap (for larval and juvenile fishes) and hence the general potential for competition at these life stages is limited. For adults, shared prey sources (zooplankton, chironomids) do not appear to be limiting. In addition to minimal evidence for negative/competitive impacts, juvenile yellow perch also provided a seasonally abundant prey resource for resident black basses in late spring when many native prey fishes were still in the larval stages and unavailable as prey to adult basses.
Acknowledgments
We thank Tammy DeVries for her help with zooplankton analysis, Chl-a, diet analysis, and lab workup of fish, and all the graduate students and technicians at the Ireland Center who helped along the way (in alphabetical order): Emily DeVries, Zach DeVries, Nathan Hartline, Keith Henderson, Tommy Purcell, Brandon Simcox, Madeline Wedge, and Stephen Woodard. Mike Maceina provided guidance on reading otoliths, Jon Hornsby helped with identification of study sites, and Alan Wilson and 4 anonymous reviewers provided helpful comments on a previous draft of this manuscript. This research was supported by the Alabama Department of Conservation and Natural Resources through Federal Aid in Sport Fish Restoration Project F-40-R and by the Alabama Agricultural Experiment Station.
References
- Abernethy DL. 2014. Bass Anglers Information Team, 2013 annual report. Birmingham (AL): Alabama Department of Conservation and Natural Resources.
- [AWW] Alabama Water Watch. 2005. Saugahatchee Creek watershed: past, present, and future. Auburn (AL).
- [AWW] Alabama Water Watch. 2012. Citizen volunteer watershed stewardship of Alabama's Reservoirs: Lake Martin watershed. Auburn (AL).
- Brooks WR, Jordan RC. 2009. Enhanced interspecific territoriality and the invasion success of the spotted tilapia Tilapia mariae in South Florida. Biol Invasions. 12:865–874.
- Browne DR, Rasmussen JB. 2009. Shifts in the trophic ecology of brook trout resulting from interactions with yellow perch: an intraguild predator–prey interaction. T Am Fish Soc. 138:1109–1122.
- Chesson J. 1978. Measuring preference in selective predation. Ecology 59:211–215.
- Chesson J. 1983. The estimation and analysis of preference and its relationship to foraging models. Ecology 64:1297–1304.
- Chevalier JR. 1973. Cannibalism as a factor in first year survival of walleye in Oneida Lake. Trans Am Fish Soc. 102:739–744.
- Clugston JP, Oliver JL, Ruelle R. 1978. Reproduction, growth, and standing crops of yellow perch in southern reservoirs. Am Fish Soc Spec P. 11:89–99.
- Cooper CM, Knight LA Jr. 1985. Macrobenthos-sediment relationships in Ross Barnett Reservoir, Mississippi. Hydrobiologia. 126:193–197.
- Dettmers JM, Stein RA. 1992. Food consumption by larval gizzard shad: zooplankton effects and implications for reservoir communities. T Am Fish Soc.121:494–507.
- Feiner ZS, Höök TO. 2015. Environmental biology of percids. In Kestemont P, Dabrowski K, Summerfelt RC, editors. Biology and culture of percid fishes: principles and practices. Netherlands: Springer.
- Fisher SJ, Willis DW. 1997. Early life history of yellow perch in South Dakota glacial lakes. J Freshwater Ecol. 12:421–429.
- Forney JL. 1974. Interactions between yellow perch abundance, walleye predation, and survival of alternate prey in Oneida Lake, New York. Trans Am Fish Soc. 103:15–24.
- Fuller PL, Nico LG, Williams JD. 1999. Nonindigenous fishes introduced into inland waters of the United States. Am Fish Soc Spec P. 27.
- Fullhart HG, Parsons BG, Willis DW, Reed JR. 2002. Yellow perch piscivory and its possible role in structuring littoral zone fish communities in small Minnesota lakes. J Freshwater Ecol. 17:37–43.
- Galbraith MG Jr. 1967. Size-selective predation on daphnia by rainbow trout and yellow perch. T Am Fish Soc. 96:1–10.
- Glover DG, DeVries DR, Wright RA. 2012. Effects of temperature, salinity, and body size on routine metabolism of coastal largemouth bass Micropterus salmoides. J Fish Biol. 81:1463–1478.
- Graeb BDS, Dettmers JM, Wahl DH, Caceres CE. 2004. Fish size and prey availability affect growth, survival, prey selection, and foraging behavior of larval yellow perch. T Am Fish Soc. 133:504–514.
- Graeb BDS, Mangan MT, Jolley JC, Wahl DH, Dettmers JM. 2006. Ontogenetic changes in prey preference and foraging ability of yellow perch: insights based on relative energetic return of prey. T Am Fish Soc. 135:1493–1498.
- Hackney PA, Holbrook JA II. 1978. Sauger, walleye, and yellow perch in the southeastern United States. Am Fish Soc Spec P. 11:74–81.
- Hansen MJ, Wahl DH. 1981. Selection of small Daphnia pulex by yellow perch fry in Oneida Lake, New York. T Am Fish Soc. 110:64–71.
- Hayes DB, Taylor WW, Scheider JC. 1992. Response of yellow perch and the benthic invertebrate community to a reduction in the abundance of white suckers. T Am Fish Soc. 121:36–53.
- Helfman GS. 1981. Twilight activities and temporal structure in a freshwater fish community. Can J Fish Aquat Sci. 38:1405–1420.
- Hirst SC, DeVries DR. 1994. Assessing the potential for direct feeding interactions among larval black bass and larval shad in two southeastern reservoirs. T Am Fish Soc. 123:173–181.
- Hokanson KEF. 1977. Temperature requirements of some percids and adaptations to the seasonal temperature cycle. J Fish Res Board Can 34:1524–1550.
- Jolley JC, Willis DW, Holland RS. 2010. Match-mismatch regulation for bluegill and yellow perch larvae and their prey in sandhill lakes. J Fish Wildl Manag. 2:73–85.
- Kaemingk MA, Jolley JC, Willis DW, Chipps SR. 2012. Priority effects among young-of-the-year fish: reduced growth of bluegill sunfish (Lepomis macrochirus) caused by yellow perch (Perca flavescens)? Freshwater Biol. 57: 654–665.
- Kelso JRM, Ward FJ. 1977. Unexploited percid populations of West Blue Lake, Manitoba, and their interactions. J Fish Res Board Can. 34:1655–1669.
- Kincaid C. 2005. Guidelines for selecting the covariance structure in mixed model analysis. Proceedings of the Thirtieth Annual SAS Users Group International Conference. Cary (NC): SAS Institute Inc.
- Kitchell JF.1990. The scope for mortality caused by sea lamprey. T Am Fish Soc. 119:642–648.
- Knight RL, Margraf FJ, Carline RF. 2011. Piscivory by walleyes and yellow perch in western Lake Erie. T Am Fish Soc. 113:677–693.
- Lovell GR, Catchings ED, McVay RA. 2011. Martin Reservoir management report, Spring 2011. Birmingham (AL): Alabama Department of Conservation and Natural Resources.
- Lyons J. 1987. Prey choice among piscivorous juvenile walleyes (Stizostedion vitreum). Can J Fish Aquat Sci. 44:758–764.
- Margenau TL, Rasmussen PW, Kampa JM. 1998. Factors affecting growth of northern pike in small northern Wisconsin lakes. N Am J Fish Manage. 18:625–639.
- Mills EL, Forney JL. 1983. Impact on Daphnia pulex of predation by young yellow perch in Oneida Lake, New York. T Am Fish Soc. 112:154–161.
- Ney JJ. 1978. Selected coolwater fishes of North America. Am Fish Soc Spec P 11:1–12.
- Padilla MA, Algina J. 2007. Type I error rates of the Kenward-Roger adjusted degree of freedom F-test for a split-plot design with missing values. J Mod App Stat Meth. 6:66–80.
- Parker AD, Uzarski DG, Ruetz CR III. 2009. Diets of yellow perch (Perca flavescens) in wetland habitats of Saginaw Bay, Lake Huron. J Freshwater Ecol. 24:347–355.
- Pelham ME, Pierce CL, Larscheid JG. 2001. Diet dynamics of the juvenile piscivorous fish community in Spirit Lake, Iowa, USA, 1997–1998. Ecol Freshw Fish. 10:198–211.
- Phillips JM, Jackson JR, Noble RL. 1995. Hatchling date influence on age-specific diet and growth of age-0 largemouth bass. T Am Fish Soc. 124:370–379.
- Post JR, McQueen DJ. 1988. Ontogenetic changes in the distribution of larval and juvenile yellow perch (Perca flavescens): a response to prey or predators? Can J Fish Aquat Sci. 45:1820–1826.
- Purcell TR. 2011. The relationship between shoreline development and resident fish communities in Lake Martin, Alabama. [master's thesis]. [Auburn (AL)]. Auburn University.
- Roberts CM. 2012. Introduced yellow perch in Lake Martin and Yates Lake, Alabama: interactions with native fishes. [master's thesis]. [Auburn (AL)]. Auburn University.
- Ruderhausen PJ, Tuomikoski JE, Buckel JA, Hightower JE. 2005. Prey selectivity and diet of striped bass in Western Albemarle Sound, North Carolina. T Am Fish Soc. 134:1059–1074.
- Rudstam LG, Green DM, Forney JL, Stang DL, Evans JT. 1996. Evidence of interactions between walleye and yellow perch in New York State lakes. Ann Zool Fenn. 33:443–449.
- Ryder RA, Kerr SR. 1978. The adult walleye in the percid community: a niche definition based on feeding behavior and food specificity. Am Fish Soc Spec P 11:39–51.
- SAS Institute. 2012. SAS Version 9.4. Cary (NC): SAS Institute Inc.
- Schael DM, Rudstam LG, Post JR. 1991. Gape limitation and prey selection in larval yellow perch (Perca flavescens), freshwater drum (Aplodinotus grunniens), and black crappie (Pomoxis nigromaculatus). Can J Fish Aquat Sci. 48:1919–1925.
- Schoener TW. 1970. Non-synchronous spatial overlap of lizards in patchy habitats. Ecology. 51:408–418.
- Shea K, Chesson P. 2002. Community ecology theory as a framework for biological invasions. Trends Ecol Evol. 17:170–176.
- Shrader T. 2000. Effects of invasive yellow perch on gamefish and zooplankton populations of Phillips Reservoir. Information Reports 2000–03. Salem (OR): Oregon Department of Fish and Wildlife.
- Strayer DL. 2010. Alien species in fresh waters: ecological effects, interactions with other stressors, and prospects for the future. Freshwater Biol. 55(Suppl. 1):152–174.
- Timmons TJ. 1975. Range extension of the yellow perch, (Perca flavescens (Mitchell)), in Tennessee. J Tennessee Acad Sci. 50:101–102.
- Timmons TJ. 1984. Comparative food habits and habitat preference of age-0 largemouth bass and yellow perch in West Point Lake, Alabama-Georgia. P Annu Conf Southeast Assoc Fish Wildlife Agencies. 38:302–312.
- VanDeHey JA, Willis DW, Harris JM, Blackwell BG. 2014. Effects of gizzard shad introductions on walleye and yellow perch populations in prairie glacial lakes. Fish Res. 150:49–59.
- Wallus R. 2006. Yellow perch, Perca flavescens (Mitchell). In: Simon TP, Wallus R. Reproductive biology and early life history of fishes in the Ohio River drainage. Percidae—perch, pikeperch, and darters. Volume 4. Boca Raton (FL): CRC Press, Taylor & Francis Group. p. 550–569.
- Welker MT, Pierce CL, Wahl DH. 1994. Growth and survival of larval fishes: roles of competition and zooplankton abundance. T Am Fish Soc. 123:703–717.
- Wicker AM, Johnson WE. 1987. Relationships among fat content, condition factor, and first-year survival of Florida largemouth bass. T Am Fish Soc. 116:264–271.