Abstract
Vitamin B12 (cobalamin) is an essential cofactor for two metabolic pathways. It is obtained principally from food of animal origin. Cobalamin becomes bioavailable through a series of steps pertaining to its release from dietary protein, intrinsic factor-mediated absorption, haptocorrin or transcobalamin-mediated transport, cellular uptake, and two enzymatic conversions (via methionine synthase and methylmalonyl-CoA-mutase) into cofactor forms: methylcobalamin and adenosylcobalamin. Vitamin B12 deficiency can masquerade as a multitude of illnesses, presenting different perspectives from the point of view of the hematologist, neurologist, gastroenterologist, general physician, or dietician. Increased physician vigilance and heightened patient awareness often account for its early presentation, and testing sometimes occurs during a phase of vitamin B12 insufficiency before the main onset of the disease. The chosen test often depends on its availability rather than on the diagnostic performance and sensitivity to irrelevant factors interfering with vitamin B12 markers. Although serum B12 is still the most commonly used and widely available test, diagnostics by holotranscobalamin, serum methylmalonic acid, and plasma homocysteine measurements have grown in the last several years in routine practice. The lack of a robust absorption test, coupled with compromised sensitivity and specificity of other tests (intrinsic factor and gastric parietal cell antibodies), hinders determination of the cause for depleted B12 status. This can lead to incorrect supplementation regimes and uncertainty regarding later treatment. This review discusses currently available knowledge on vitamin B12, informs the reader about the pitfalls of tests for assessing its deficiency, reviews B12 status in various populations at different disease stages, and provides recommendations for interpretation, treatment, and associated risks. Future directions for diagnostics of B12 status and health interventions are also discussed.
Introduction
Historical background
As early as the nineteenth century, James Combe [Citation1], Thomas Addison [Citation2], and Anthony Biermer [Citation3] wrote about a fatal diathesis now recognized as vitamin B12 (B12, synonym cobalamin [Cbl]) deficiency caused by pernicious anemia (PA). William Osler and William Gardner described “progressive pernicious anemia” (a term used five years earlier by Biermer) in a 52-year-old Englishman with numbness of the fingers, hands, and forearms, as well as large red blood cells [Citation4]. Increased marrow cellularity had already been reported by Julius Cohnhein [Citation5]. The pathophysiological consequences of PA were extended to spinal cord lesions reported by Lichtheim [Citation6], with Russell et al. coining the term “subacute combined degeneration of the spinal cord” (SCDC). In 1900, Russell noted characteristics of B12 deficiency that are overlooked with surprising frequency today: “some of the most typical cases presented no anemia throughout the course, others only late in the disease, while in other cases anemia was an obtrusive symptom … and preceded the nervous symptoms by many months” [Citation7].
Whipple and Robscheit-Robbins discovered that feeding liver to anemic dogs promoted the regeneration of hemoglobin, leading George Minot and William Murphy to the first effective treatment for PA when they fed raw and later cooked liver to patients [Citation8,Citation9]. In 1934, Whipple, Minot, and Murphy received the Nobel Prize for their work. While searching for the remission-inducing component, Minot used an early method for counting reticulocytes that enabled him to address the hematological response to different liver fractions. Other than this “human bioassay” (i.e. testing liver fractions on patients with PA) no reliable laboratory diagnostics were available to support the search for the extrinsic antipernicious factor(s) over the next 20 years. A breakthrough came in 1946 when Lester Smith noticed that the most potent liver fractions were reddish. We now know that B12 contains a system of conjugated double bonds, which confer the red color and make B12 easily detectable at high concentrations. Several teams worked on isolating the colored material in quick succession, providing Dorothy Hodgkin with the opportunity to undertake her remarkable X-ray crystallographic studies for which a second Nobel Prize in the vitamin B12 field was awarded in 1964 [Citation10].
Vitamin B12 structure
Vitamin B12 is one of the largest and most structurally complex nonpolymeric biomolecules described in nature, with a molecular weight of 1355 daltons for its frequently used cyano- form. The generic term cobalamin refers to a set of structures named corrinoids, consisting of one central cobalt (Co) atom coordinated with 4 equatorial nitrogen atoms contributed by pyrrole residues (). The 5th coordination site (α) in B12, below the planar structure, is occupied by a 5′,6′-dimethyl benzimidazole residue linked to a α-ribosyl-3-phosphate. The 6th coordination site (β), above the corrin ring, is occupied by a methyl- (methylcobalamin [Me-Cbl]), 5′-deoxyadenosyl (5′-deoxyadenosylcobalamin [Ado-Cbl]), aquo- (hydroxocobalamin [OH-Cbl]), or a cyano-group (cyanocobalamin [CN-Cbl]). The metabolic utility of B12 in humans is conferred by an upper ligand consisting of either a methyl or adenosyl moiety. Cyanocobalamin is the most chemically stable form, though it has no vitamin activity per se. Cyanocobalamin undergoes enzymatic conversions during intracellular processing in human cells, as described below, and it is eventually converted to the two cofactors, Me-Cbl and Ado-Cbl [Citation11]. Me-Cbl and Ado-Cbl regularly undergo catalytic reductions and oxidations and occasionally generate OH-Cbl, which returns to the catalysis via a reactivation cycle [Citation12].
B12 sources and de novo biosynthesis
Vitamin B12 is synthesized exclusively by micro-organisms, sometimes inhabiting higher plants that cannot produce B12. Fermentation in the gastrointestinal tract of herbivorous animals supports the growth of micro-organisms that synthesize B12, which is then absorbed by the animal and incorporated into its tissues [Citation13]. Omnivores and carnivores, including humans, acquire B12 from animal tissues or animal products such as milk, cheese, and eggs. Liver in particular is a very rich source of cobalamin, followed by kidney and heart. Although the B12 content of various types of milk is not high, regular intake of milk is associated with higher serum B12 concentration in healthy elderly adults [Citation14]. Egg intake does not significantly contribute to higher serum B12 concentration [Citation14]. Vitamin B12 exists in foods in several forms; meat and fish contain mostly Ado-Cbl and OH-Cbl, OH-Cbl predominates in milk, and Me-Cbl with Ado-Cbl and OH-Cbl are found in nearly all dairy products [Citation15–17]. Animals also procure B12 through coprophagia and as a consequence of bacterial contamination of their feed.
Biosynthesis of B12 is restricted to certain bacterial strains, such as Lactobacillus rossiae and Lactobacillus reuteri [Citation18,Citation19], and involves one of two alternative routes, either the aerobic or anaerobic pathway in bacteria and archaea, respectively. Approximately 30 enzyme-catalyzed reactions are responsible for the synthesis of full Cbl. The process starts with condensation leading to uroporphyrinogen III (the first macrocyclic intermediate in tetrapyrrole synthesis), continues via the transformation of uroporphyrinogen III into cobinamide, and ends with nucleotide loop assembly and attachment to the corrin ring [Citation20]. To conserve energy, many gram-negative bacteria salvage B12 or other corrinoids and transport them into the cell [Citation21].
Dietary intake and bioavailability
Dietary intake of B12 exceeds the metabolic requirement for the majority of people and leads to the accumulation of substantial hepatic stores of ∼1–5 mg. The typical Western diet provides ∼4–6 µg/d of B12, from which 1–5 µg is absorbed [Citation22]. The bioavailability of B12 from food is assumed to be ∼50% for healthy adults with normal absorption, while the absorption of crystalline B12, present in supplements and fortified food products, is between 55 and 74%. However, the absorption from specific foods can vary greatly, for example, 24 to 36% for egg products (B12 dose 0.3–0.94 μg), 42% for fish (dose 1.95–2.18 μg), and 65% for lean meat (dose 0.95 μg) [Citation23]. Data from the 1999–2000 National Health and Nutrition Examination Survey (NHANES) indicates that the median intake of B12 for the United States (US) population is 3.4 µg/d [Citation24]. It should be noted that intakes are often reported collectively for adults from different populations [Citation25], despite the fact that dietary intake of B12 varies by sex and age. For example, the evaluation of micronutrient consumption across United Kingdom (UK) adults in their twenties, thirties, forties, and fifties showed the daily B12 intake for females to be statistically lower amongst females aged 20–59 years when compared with males of the same age (p < .001) [Citation25].
Recommended intakes
The recommended intakes were set to maintain normal hematological values and serum B12 within normal concentrations. They also assume that 50% of vitamin B12 is absorbed from the diet. Intakes of 1.5 μg/d of B12 are recommended by the UK government [Citation26] and 4 μg/d (adequate intake [AI], covers the needs of all in the group) by the European Union for adults [Citation27]. The US recommends an intake of 2.4 μg/d [Citation28]. Higher intakes are recommended for pregnancy and lactation in the US (2.6 and 2.8 μg/d, respectively) and lactation in the UK (2.0 μg/d). The recommended intakes for children vary from 0.3 μg/d for 0–6 month olds to 1.0 μg/d for 9–13 year olds in the UK and 0.4 μg/d to 1.8 μg/d for the same age groups in the US [Citation26,Citation28].
It remains debatable whether the above intakes are sufficient to achieve optimum vitamin B12 status. Using a factorial approach, which includes a summation of daily losses that need to be compensated for by dietary intake of vitamin B12, Doets et al. [Citation23] estimated that vitamin B12 intakes ranging from 3.8 μg/d to 20.7 μg/d are needed to prevent deficiency in apparently healthy adults and elderly people.
In addition to the above, due to the high prevalence of food-bound malabsorption (inability to release vitamin B12 from its binding proteins) in people >60 years of age, many European countries recommend food products fortified with crystalline vitamin B12, as its absorption is not affected in this condition [Citation29].
Vitamin B12 absorption
There are two mechanisms whereby B12 is absorbed: (i) passive diffusion and (ii) a receptor-mediated process. Only about 1–2% of an oral dose can be absorbed passively via mucous membranes and the surface of the gastrointestinal tract [Citation30]; hence, high doses of oral B12 (e.g. 1 mg daily) are required to provide an “adequate” intake of B12 if the specific transport does not function.
Absorption via receptors is initiated after release of cobalamin from the dietary matrix. This begins in the stomach due to the action of gastric acid and by the digestion of food by pepsin. The stomach contains B12–specific proteins as well as intrinsic factor (IF) and haptocorrin (HC, also known as R-binder), which are synthesized by gastric parietal cells and the salivary glands, respectively. HC and IF compete for the liberated vitamin but, at an acidic pH, HC has a higher affinity for B12 (). When gastric contents enter the first part of the duodenum, with an alkaline environment, R-binders become partly digested by pancreatic proteases, freeing up the vitamin, which subsequently attaches to IF.
Figure 2. Vitamin B12 absorption and intracellular processing via two enzymatic pathways. In the absence of vitamin B12, 5-MTHF becomes metabolically trapped in this form producing a pseudo folate-deficient state (methyl-trap) and cannot be utilized for regeneration of THF. Cbl: cobalamin; CBS: cystathionine beta-synthase; dTMP: deoxythymidine monophosphate; dUMP: deoxyuridine monophosphate; DHFR: dihydrofolate reductase; HC: haptocorrin; holoTC: holotranscobalamin; HO-Cbl: hydroxocobalamin; IF: intrinsic factor; MS: methionine synthase; Me-Cbl: methylcobalamin; MTHFR: methylene tetrahydrofolate reductase; MMA: methylmalonic acid; MCM: methylmalonyl-CoA mutase; 5-MTHF: 5-methyltetrahydrofolate; SAH: S-Adenosyl homocysteine; SAM: S-Adenosyl methionine; THF: tetrahydrofolate; TS: thymidylate synthase; TC: transcobalamin.
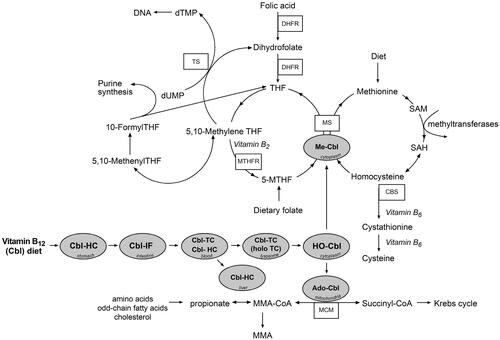
In the terminal ileum, the vitamin B12-IF complex binds to cubam receptors comprised of amnionless and cubilin protein moieties on intestinal mucosal enterocytes [Citation31]. The complex is then taken up by endocytosis into the ileal cell. This process is highly specific and only takes place at a neutral pH in the presence of calcium ions. The receptors only take up the vitamin B12 bound to IF [Citation32]. This step is rate-limiting since ileal receptors have a finite capacity for B12 amounting to 1.5–2.5 µg from a single meal. Based on early studies with isotopes, it was shown that 50% of a 1 μg oral dose was absorbed, 20% of a 5 μg dose, and 5% of a 25 μg/d dose () [Citation33,Citation34]. A recent study by Devi et al. [Citation35], which used [13C]-cyanocobalamin, achieved similar results (). The receptors are recycled to the surface and become available again within 4–6 h [Citation36]. After internalization, IF is degraded by lysosomal proteases, releasing the vitamin B12 for transport by the lysosomal transmembrane protein, LMBD1 [Citation37]. From the cytosol, B12 is exported to blood circulation by the ATP-driven exporter, multidrug resistance protein (MRP1) [Citation38]. In the blood, free B12 immediately binds two proteins: transcobalamin (TC) and HC (). Transcobalamin is apparently synthesized by all tissues and circulates in a predominately unsaturated form [Citation39]. Most newly-absorbed B12 binds to TC, forming holotranscobalamin (holoTC). HoloTC has a much faster turnover than the B12–HC complex, holohaptocorrin (holoHC), but controversies exist over its rate of clearance, which is likely to be attributed to methodologies used for this estimation. This is best exemplified by Hom et al., where authors first estimated half-life (t1/2) = 1.5 h of labeled B12 bound to TC [Citation40], and 15 h [Citation41] in their later study, based on the assumption that radioactive B12 does not reappear due to reutilization once cleared from plasma. This latter approach calculated the plasma disappearance half-life obtained from the final slope of the plasma curve, which probably included both clearance and reappearance of B12 [Citation41]. A half-life of 6 min was reported by others and, more recently, 7 min for clearance of a holoTC-conjugate in patients with malignancies [Citation42].
Figure 3. Relationship between the intake and percentage of absorbed B12 from a dose of [58Co]-cyanocobalamin (Adams et al. [Citation34]) and [13C]-cyanocobalamin (Devi et al. [Citation35]) in n = 10–12 healthy volunteers.
![Figure 3. Relationship between the intake and percentage of absorbed B12 from a dose of [58Co]-cyanocobalamin (Adams et al. [Citation34]) and [13C]-cyanocobalamin (Devi et al. [Citation35]) in n = 10–12 healthy volunteers.](/cms/asset/da5da3de-aa68-4441-a214-46cd3ac80f35/ilab_a_1885339_f0003_b.jpg)
Only ∼10% of total TC is normally bound to B12 and this fraction is responsible for the transport of ∼4 nmol/d of B12 into cells [Citation41]. If B12 is no longer absorbed, circulating holoTC is not formed. Because the half-life of holoTC is much shorter than that of holoHC (10 days) [Citation41], low holoTC is believed to be the earliest indicator that a patient is no longer absorbing vitamin B12 from food [Citation43].
Conversely, HC in blood is almost fully saturated with B12 and inactive B12 analogs, and although this protein carries the majority of the vitamin in the circulation (∼80%), only few sites are available for absorption of HC-B12. Haptocorrin attaches to cell surface receptors on the liver, with a turnover of 0.1 nmol/d of B12 [Citation44]. There is no evidence that HC has any role in the cellular uptake of vitamin B12, however, its role is still poorly understood. One suggestion for its function is the transportation of potentially harmful cobalamin analogs (other corrinoids) to the liver for secretion into the bile, thus ensuring that only holoTC is available for cellular uptake [Citation45].
Vitamin B12 excreted in the bile is effectively reabsorbed through enterohepatic circulation. The amount of B12 excreted in bile varies from 1 to 10 μg/d. Biliary B12 is reabsorbed across the ileal enterocytes and requires IF, in the absence of which nearly all cobalamin is excreted with the feces. People with a low dietary intake of B12 increase their efficiency of reabsorption of B12. Efficient reabsorption is the reason why it can take some time for deficiency to develop, even in those people whose diets are very low in B12. In their systematic review, Doets et al. [Citation23] estimated that 0.13% of the total body store is lost daily, while the mean store values were between 1.1 and 3.9 mg. Based on these values, total losses of B12 would range from 1.4 to 5.1 μg/d. Assuming a normal initial store (1.1–3.9 mg) and a loss of 0.13% of B12 per day, we can expect that 10% of the whole store remains after approximately 692 days. In his study, Paul Golding [Citation46] experimentally addressed the time scale for development of B12 deficiency and assessed it as 500–700 days based on vitamin B12 markers being in deficiency ranges following a period of lower or nil B12 intake. There was a 71% decrease in serum B12 after 728 days of B12 intake depletion and a 70% (609 days) and 81% (658 days) increase in plasma homocysteine and MMA concentrations, respectively. The subject in this study was diagnosed with B12 deficiency in the past, was B12 replete at baseline, and gradually decreased his B12 intake to 0 µg on day 371 of this experiment; he then took 100 µg of vitamin B12 for 4 weeks, but from day 497 to 751 his B12 intake was 0 µg [Citation46]. Despite the variations in B12 intake, the results produced in this work suggest that the liver’s stores may not maintain adequate vitamin B12 status for a long time. This was also recently suggested by Kornerup et al. [Citation47] in their study on patients following bariatric surgery, where increased methylmalonic acid and decreased holoTC were found as early as 2 months after surgery.
For factors that can affect B12 absorption, the reader is referred to the malabsorption section.
Metabolic processing and function
There are only two intracellular enzymes for which vitamin B12 is required, namely, methionine synthase (MS) in the cytosol and methylmalonyl-CoA mutase (MCM) in the mitochondria. Cellular processing leading to formation of methylcobalamin and adenosylcobalamin, the active vitamin forms supporting enzyme activity, starts with the transport of holoTC into cells. There are receptors for holoTC on the surface of all DNA-synthesizing cells. Following endocytosis of the holoTC complex by the CD320 receptor [Citation48], the complex is degraded in lysosomes and B12 is released into the cytoplasm via two proteins, LMBD1 (cblF) [Citation37] and ABCD4 (cblJ) [Citation49]. In the cytosol, the cblC protein dealkylates methylcobalamin and adenosylcobalamin as well as decyanates cyanocobalamin to cob(I)alamin, which, under aerobic conditions, is oxidized to cob(II)alamin and hydroxycob(III)alamin (cob(III)alamin) [Citation12,Citation50,Citation51]. Kolhouse et al. [Citation52] provided evidence that cob(II)alamin was the form most active in binding to apo-methionine synthase (cblG). CblC combines with the cytosolic form of cblD to direct B12, probably as cob(II)alamin, to methionine synthase (cblG) for the formation of methylcobalamin (Me-Cbl) in the presence of methionine synthase reductase (cblE) [Citation53–55]. The mitochondrial form of cblD in combination with cblC directs B12 to mitochondria where it is converted to adenosylcobalamin (Ado-Cbl) in the presence of the gene products of cblA, cblB, and methylmalonyl-CoA mutase (MCM) () [Citation11].
Methylcobalamin is essential for the remethylation of homocysteine to methionine by MS; 5-methyltetrahydrofolate (5-MTHF) supplies the methyl group and is converted to tetrahydrofolate (). Methionine is subsequently adenosylated to S-adenosylmethionine (SAM) to supply methyl groups that are critical for the methylation of proteins, phospholipids, neurotransmitters, RNA, and DNA.
Conversely, Ado-Cbl is a cofactor for MCM, catalyzing the conversion of methylmalonyl-CoA to succinyl-CoA. Methylmalonyl-CoA is a degradation product of propionate. In most mammals, propionate arises from the utilization of some amino acids (isoleucine, valine, methionine, threonine, thymine) or cholesterol as well as after β-oxidation of odd-chain fatty acids.
Inborn errors of vitamin B12 absorption and intracellular metabolism
A number of genes that encode for proteins involved in B12 absorption and transport harbor highly penetrant genetic mutations that cause inborn errors of vitamin B12 absorption and metabolism. All of these diseases are characterized by a deficiency of the coenzyme forms of B12. They can be successfully diagnosed and treated with regular high doses of B12 injections, B12 supplements, and other therapies [Citation56].
Inborn errors of B12 absorption
Congenital PA is caused by mutations in a gene (GIF, CBLIF) and defects in IF synthesis. The condition usually presents during the first five years of life, but it can manifest later if a partial defect is present [Citation57]. This disease exhibits many features resembling non-dietary adult PA, an autoimmune disorder associated with autoantibodies to gastric parietal cells or gastric IF, as well as Immerslund-Gräsbeck (IGS) disease [Citation58].
IGS is a rare, potentially life-threatening, autosomal recessive disorder caused by mutations in either the CUBN or AMN gene, responsible for the synthesis of the cubam receptors. This disorder is clinically highly heterogeneous. It is accompanied by a mild proteinuria in ∼50% of cases, owing to cubam receptor expression also in kidney cells. In terms of treatment, life-long B12 injections lead to resolution of PA and IGS-anemia [Citation58,Citation59].
Genetically predetermined haptocorrin deficiency leads to a benign condition not requiring treatment [Citation45]. Interestingly, some animals such as rodents do not produce HC and survive with just TC and IF [Citation60]. Diagnosis is often suspected following a low serum B12 concentration in the absence of any symptoms related to deficiency. Conversely, genes that cause transcobalamin deficiency will often result in a severe phenotype [Citation61] if not treated with high doses of vitamin B12 from an early age.
Inborn errors of intracellular metabolism
To rationalize the etiological heterogeneity of these disorders, they have been catalogued into a total of eight defects of Cbl metabolism [Citation57], designated cblA-cblG and mut, defined by means of in vitro somatic complementation analysis. Defects in genes encoding for cbl proteins result in a failure to utilize B12 by the target cells irrespective of its normal supply. In the cblC, cblD, cblF, and cblJ group of disorders, the synthesis of both Ado-Cbl and Me-Cbl are affected, leading to combined methylmalonic aciduria and homocystinuria. In cblA and cblB disorders, the synthesis of Ado-Cbl is impaired, resulting in methylmalonic aciduria but normal homocysteine concentrations, whilst cblE and cblG disorders result in homocystinuria without methylmalonic aciduria [Citation57]. Finally, the mut defect describes mutations (>200 identified) in the MUT gene (encodes for MCM) that partially (class mut−) or totally (class mut0) abolish MCM-mediated conversion of methylmalonyl-CoA to succinyl-CoA and so perturb substrate (odd-chain fatty acids, branched-chain amino acids, and cholesterol) utilization and energy metabolism in the mitochondria [Citation62].
Of the eight defects, the cblC defect is the most common, although still rare with an incidence ∼1/200,000 births. Clinical manifestations often appear in infancy but can also occur later in life. Typical symptoms associated with early-onset disease are feeding difficulties, failure to thrive, hypotonia, seizures, pigmentary retinopathy, developmental delay, and macrocytic anemia. Late-onset disease is frequently accompanied by neurological dysfunction, including cognitive decline, confusion, psychosis, or dementia. In comparison with early-onset patients, late-onset patients have better survival and response to therapy [Citation57,Citation63]. For a detailed description of other intracellular disorders and response to treatment the reader is referred to detailed reviews on this subject [Citation57,Citation62,Citation64].
Genetic polymorphisms associated with poor B12 status
Developments in genomic technology have allowed us to move beyond single-gene disorders and to study the polygenic basis of vitamin B12 status. This has led to the discovery of a myriad of new genes/pathways that harbor genetic polymorphisms associated with variable B12 status among different population groups. The most extreme consequences of genetic variation have already been described in the case of rare inborn errors of B12 metabolism. More common effects of genetic variation in B12 levels can be described by its heritability (h2) in different populations as well as associations between specific polymorphisms in genes and the various indicators of B12 status. Dib et al. [Citation65] have provided robust h2 estimates for all markers of vitamin B12 status for UK-based Caucasian populations that range from ∼15% in the case of MMA right up to ∼80% for holoTC, highlighting that B12 status is a complex and multifactorial trait, whereby several polymorphisms in multiple genes interact with the environment to cause variable B12 status. So far, candidate gene and genome-wide association studies (GWAS) have identified ∼59 SNPs from 19 genes involved in cobalamin metabolism and various indicators of its status [Citation66]. We refer the reader to Surendran et al. [Citation66], who provide a very useful guide for each gene and polymorphism associated with B12 status, but would like to highlight that most, if not all, of the findings thus far refer to “associations” identified mainly through observational studies with currently unclear clinical and pathophysiological significance. Therefore, “causal” genetic determinants of vitamin B12 status, functional consequences, and context dependency remain poorly understood [Citation65,Citation67].
Corrinoids and microbiome
As stated before, cobalamin belongs to a family of compounds referred to as corrinoids. Corrinoids with a cobalt ion in the corrin ring are called cobamides. Vitamin B12 is differentiated from other cobamides (analogs), which usually cannot provide cofactor activity in mammalian cells, by having a 5′,6′-dimethyl benzimidazole group attached to the lower nucleotide loop. There are also other corrinoids (analogs) that differ in the tetrapyrrole ring or have a central atom other than cobalt, such as nickel, copper, or zinc. Corrinoids are synthesized exclusively by bacteria and archaea, and are abundant in the large intestine due to the activity of the gut microbiota [Citation68]. Corrinoids play key roles in shaping the gut microbial community composition and diversity [Citation69]. A majority of human gut microbial species either directly require access to the host’s dietary-derived B12 or are dependent on analogs [Citation70] produced by other bacteria [Citation71]. In humans, bacterial synthesis of vitamin B12 and analogs takes place only in the large intestine and the cecum, and there is evidence that humans cannot utilize bacterial B12/analogs [Citation32] as part of the orchestrated steps that ensure adequate absorption and reabsorption of dietary-only Cbl. However, there is some evidence to show that certain diseases or age-related changes to the gastrointestinal tract can lead to protein-bound vitamin B12 malabsorption and that bacterial-derived analogs may play a role [Citation72–74].
Analogs act as cofactors for corrinoid-dependent enzymes for gut microbes, hence the constituents of the microbiome compete with the host, as well as other bacteria, for B12 or corrinoid analogs, in order to ensure viability and stability of the microbiome ecology. Previous studies have predicted that ∼86% of human gut bacteria species are dependent on corrinoids as cofactors but <25% have the capacity to synthesize them de novo [Citation75]. Although many bacteria cannot synthesize B12, they can remodel it by removing the original nucleotide and adding a new one. About 50% of total dietary Cbl is thought to be converted to other corrinoids by the gut bacteria [Citation76]. Therefore, a majority of gut bacteria either rely on Cbl-uptake mechanisms from either the host’s diet or are dependent on related corrinoids [Citation77] produced by other bacteria [Citation78]. This is likely associated with the individual variability in dietary Cbl exposure as well as the specificity of the individual gut microbial community composition. For example, Visconti et al. [Citation79] have shown that only 43% of bacterial species are shared by any two randomly selected individuals from the population. Estimates suggest high dietary deficiency rates in the population, especially in an exponentially increasing number of individuals who, for ethical and health reasons and also encouraged by scientific observations, have been shifting toward a higher consumption of plant-based food [Citation80]. Although Cbl deficiency is a modifiable risk factor, which itself is associated with a number of rare/common non-communicable (neurological/vascular) diseases [Citation81], evidence of the specific role of Cbl and other corrinoids, in shaping the gut bacterial community and as mediators of human-microbiome symbiosis, and their impact on human health, remains largely unknown [Citation76,Citation82,Citation83]. Analogs were found to delay growth in chicks [Citation84] or induce severe demyelinating disease in baboons [Citation85]. Mechanisms that prevent absorption and tissue dissemination of analogs have evolved, including the poor affinity for IF. Of those that are bound by IF, most appear to be retained by the ileum. Analogs that do reach plasma are largely bound to haptocorrin and are transported to the liver and excreted via the bile and feces. Analogs may also be bound by transcobalamin and transported to tissue [Citation83], but the amount absorbed might be insignificant [Citation86].
Diagnosis of deficiency and insufficiency – clinical and laboratory pitfalls
One might suppose that diagnosing B12 deficiency should be relatively simple. The laboratory provides a diagnostic “cutoff” point, the patient is proclaimed to be deficient, and the vitamin should be replenished. The truth can be far more complicated, especially if insufficiency of B12 (subclinical deficiency) is also considered. Clinical vitamin B12 deficiency is often the result of prolonged and severe malabsorption and patients are symptomatic, while in insufficiency, symptoms associated with B12 deficiency are not well expressed but biochemical markers, most notably total plasma homocysteine (tHcy) and methylmalonic acid (MMA), are elevated [Citation87]. In such cases, serum B12 concentration is often normal. However, relatively high holoTC, low serum B12, and high MMA may also be seen in some patient cohorts with B12 insufficiency [Citation88].
Therefore, in the course of B12 status assessment, the physician can encounter pitfalls at each step of the process including: (A) consideration of B12 deficiency as a major cause for presenting illness; (B) testing for B12 deficiency/insufficiency and interpreting results; (C) investigating the potential causes of deficiency/insufficiency; (D) treatment; and (E) considering the associated risks.
Consideration of B12 deficiency as a major cause of presenting illness
B12 deficiency can masquerade as a multitude of illnesses; therefore, the physician requires considerable clinical acumen. The disease presents different perspectives from the point of view of the hematologist, neurologist, gastroenterologist, general physician, dietician, and psychiatrist, and is a prime example of “elephanomics” [Citation89]. A high index of suspicion is required. Importantly, the absence of anemia does not preclude a positive diagnosis, a trap easily fallen into since many physicians erroneously equate B12 deficiency with PA, though the latter is only one potential cause of the former [Citation90].
Classical features of deficiency include those relating to anemia (weakness, tiredness, dyspnea on exertion), gastrointestinal symptoms (appetite loss, sore tongue and mouth, epigastric discomfort, nausea, vomiting, heartburn), neurological symptoms (numbness of extremities, pins and needles, impaired fine finger movements, gait ataxia, positive Romberg’s sign, impaired vibration and position sense, orthostatic dizziness, loss of taste or smell), and those relating to psychological and psychiatric disturbances (irritability, personality change, memory and intellectual impairment, disorientation, depression, psychomotor slowing, delirium, dementia). The deficiency predominantly affects hematological and neurological parameters, but neuropsychiatric symptoms are often the first clinical manifestation [Citation91]. Gynecological and urological symptomatology (infertility, cystitis, and pyelonephritis), though rare, should also be considered. The full spectrum of clinical features associated with deficiency is perhaps broader. In a survey of individuals with PA, symptomatology also included brittle nails, flushing, fever, hair loss and graying, and nominal aphasia [Citation92]. Occasionally, the deficiency is entirely asymptomatic, its discovery arising from the alert physician pursuing abnormal results from a routine full blood count.
Megaloblastic anemia describes the morphological features of hematopoietic tissue caused by a deficiency of B12 (and/or folate). It includes ineffective erythropoiesis, moderate hemolysis, and inefficient leukopoiesis and thrombopoiesis [Citation93]. Abnormal morphology of blood elements include normochromic and macrocytic erythrocytes, lower reticulocyte count, hyper segmented neutrophils, abnormal morphology of bone marrow cells, and megaloblastic changes in granulocytes and platelet precursors [Citation93]. Megaloblastic cells are in a state of unbalanced growth with impairment of DNA synthesis and an increase in the RNA/DNA ratio. Morphological mimics include myelodysplastic syndromes and sideroblastic anemia [Citation94]. Conversely, B12 deficiency can co-exist with iron deficiency or thalassemia, thus masking macrocytosis.
It was generally believed that hematological features preceded any neuropsychiatric abnormality, but in the last few decades it has been noted that these manifestations might be considered as two major, and sometimes entirely separate (or even excluding each other), clinical syndromes [Citation95,Citation96]. It remains unclear why some B12-deficient patients present with neurological disorders in the absence of hematological changes and why some develop a predominantly cerebral picture, while others a spinal or peripheral nerve disorder. This may be partially related to folate status, as folate deficiency is primarily responsible for hematological changes due to defective DNA synthesis. A good supply of folates may delay the hematological symptoms of B12 deficiency, even though tetrahydrofolate (THF) is not regenerated from 5-MTHF (). In the absence of vitamin B12, 5-MTHF becomes metabolically trapped in this form, producing a pseudo folate-deficient state (methyl-trap) [Citation97]. The methyl-trap hypothesis explains why vitamin B12 deficiency produces apparently identical megaloblastic anemia to that seen in folate deficiency. In both cases (folate and vitamin B12 deficiency), the cells would be deficient in folate cofactors required for DNA and RNA synthesis. It also explains the clinical observation that treating a patient with megaloblastic anemia caused by vitamin B12 deficiency with a pharmacological dose of folic acid allows the cells of the bone marrow to start to divide again. It is thought that the new folate molecules would carry out several such cycles before they are trapped as 5-MTHF (). If folic acid continued to be administered to a patient with megaloblastic anemia, which would occur if the wrong clinical diagnosis had been made, hematological remission would be maintained [Citation97].
Our “preconception” of B12 deficiency still associates it with anemia, and its diagnosis usually proceeds in three steps: the recognition of anemia, the observation that it is macrocytic, and finally, the confirmation of an underlying deficiency. However, if suspicion of the deficiency were based on the presence of macrocytic anemia alone, a substantial proportion of B12-deficient individuals would escape detection [Citation90,Citation98], a point elegantly expressed by Carmel, who notes that “The proscription that vitamin B12 deficiency should not be diagnosed unless megaloblastic changes are found is akin to requiring the presence of jaundice to diagnose liver disease” [Citation99].
Early presentation, due to increased physician vigilance and heightened patient awareness, can result in tests during a phase of the illness when laboratory findings of a “full-blown” deficiency are not yet apparent. Before patients become clinically deficient, they traverse earlier stages, beginning with insufficiency. The concept of B12 deficiency as a gradually progressive disorder [Citation99] is an important development over the last 30 years [Citation100]. The five stages of vitamin B12 status/deficiency described by Victor Herbert in 1987 [Citation100] are likely to be applicable to the majority of cases today: stage I – normal vitamin B12 status; stage II – negative vitamin B12 balance; stage III – vitamin B12 depletion accompanied by possible clinical signs and symptoms such as fatigue, dizziness, nausea or poor appetite, diarrhea, palpitations and rapid heartbeat, bleeding gums, and mouth sores; stage IV – deficient erythropoiesis with potentially reversible neurological symptoms such as numbness and tingling in the hands and feet, ataxia, muscle weakness, depression and psychosis; and stage V – related anemia with possible irreversible neurological symptoms.
Testing for B12 deficiency/insufficiency and interpretation of laboratory results
“We sanguinely hope that the laboratory will answer our questions with a dogmatic ‘yes’ or ‘no’; its usual answer is perhaps” (Anon).
There are four biological markers of B12 deficiency: total serum B12, holoTC (also known as “Active B12”), and measures of the related metabolites tHcy and MMA. Each has its limitations, which the physician should be alert to.
Serum B12 test
Serum B12 is the most frequently used laboratory marker of B12 status, measuring the circulatory concentration of B12 bound to both vitamin binding proteins, TC, and HC. As is the case for a number of other vitamins, serum B12 was initially measured by microbiological methods, for example, using Lactobacillus leichmannii, for which cobalamin is an obligate nutrient [Citation101]. Microbiological assays for B12 suffered from inter-laboratory variability, interference by antibiotics, and an extended turnaround time inherent to the assay principle. The main disadvantage however is its low specificity when compared to IF-based assays [Citation102]. These assays were challenged by nonspecific R-protein-binding assays due to availability of R-proteins from saliva, for example. However, the values obtained with these were markedly higher than those obtained with the microbiological assays, as they measured a broad variety of cobalamin analogs that are not necessarily biologically active [Citation83].
Since the 1990s, clinical laboratories have opted for high throughput automated competitive binding chemiluminescence assays (CBLA), using purified IF as a reagent, to measure total cobalamin after its release from endogenous binding proteins [Citation103]. Caution should however be observed when interpreting results, since some of these assays could be influenced by the presence of interfering anti-IF antibodies, particularly in patients with PA, thereby providing spuriously elevated serum cobalamin concentrations in otherwise B12-deficient patients [Citation104–106].
Serum B12 can also be determined using B12-antibodies and B12 enzyme-linked immunosorbent assay (ELISA) kits. Various kits are available. Although these could potentially provide an alternative to IF-based assays, they have not been completely verified [Citation107–109].
Functional indicators of B12 status, tHcy and MMA, are based on the rationale that deficiency leads to the deactivation of two key enzymes of the one-carbon cycle, namely MS and mitochondrial MCM, causing the accumulation of tHcy and MMA, respectively [Citation110]. While providing a better insight into the B12 status of different groups of individuals, the lack of consensus in choosing cutoff values for each of the biomarkers remains problematic in diagnosing B12 deficiency. Reported cutoffs for B12 vary from as low as 100 pmol/L (Immulite 2000) when associated with plasma MMA concentrations ≥260 nmol/L [Citation111], to as high as 258 pmol/L (IF radioassay, Quantaphase) on the basis of increased MMA [Citation112]. These two reports exemplify the uncertainties in the reported cutoffs for individual biomarkers of B12 status. Shelub et al. [Citation113], in a study based on NHANES III 1991–1994 and NHANES 1999–2002 involving ∼5000 middle-aged participants in both cohorts, used <148 pmol/L as the conventional cutoff for B12 deficiency. Using this cutoff, they reported a prevalence of 1.6 to 2.2% of the deficiency in NHANES III and NHANES, respectively, and showed significant differences in circulating tHcy and MMA between subjects with B12 deficiency vs normal status. In a 2001 epidemiological study based on combined NHAHES 1999–2000, 2001–2002 and 2003–2004 data totaling 12,612 middle-aged adult participants, Bailey et al. [Citation114] reported a steady increase in the prevalence of B12 deficiency, from 2.9% to 25.7% by moving decision points from <148 pmol/L to >296 pmol/L of total serum B12. They observed a similar trend but of much less importance (2.3 ± 0.2% to 5.8 ± 0.3%) when the cutoff point for MMA was decreased from >376 to >271 nmol/L. Concerned by the impact of different B12 and MMA cutoffs in the diagnosis of clinical outcomes and using data collected from the 1999–2004 surveys and different statistical models, Bailey et al. [Citation115] attempted to identify a single change point at which the relation between plasma MMA and serum B12 changes slope to differentiate between inadequate and adequate B12 status, but the results were not as simple as expected. They reported three slopes resulting in two change points for three subgroups. The first subgroup had serum B12 <126 pmol/L and the highest median plasma MMA of 281 nmol/L. This group, representing 1.2% of the population studied, was considered at high risk for severe deficiency because of the frequently accompanying elevation of MMA and tHcy. The second group, representing 67.6% of the population studied, in which serum B12 was >287 pmol/L, likely had adequate B12 status with a median MMA concentration of 120 nmol/L. The third group (33%) was classified as indeterminate and difficult to interpret because of intermediate serum concentrations of B12 (126–287 pmol/L). Although none of these studies provide a clear cutoff, they all call for the measurement of associated metabolites when assessing B12 status.
Holotranscobalamin testing
HoloTC, the bioactive form of B12, is also routinely used for evaluating B12 status, often in combination with an MMA test [Citation116,Citation117]. It remains debatable whether holoTC “alone” serves any better than the measurement of serum B12. It has particularly been criticized by Golding [Citation118], who provides an extensive review and argumentation concluding that “the holoTC immunoassay cannot be used to measure B12 status any more reliably than total B12, or to predict the onset of a metabolic deficiency, because it is based on an erroneous hypothesis and a flawed model for the staged development of B12 deficiency.” This conclusion is challenged by Kornerup et al. [Citation47], who provide evidence that holoTC is superior to total B12 in detecting early changes in the vitamin’s status following bariatric surgery. Jarquin Campos et al. [Citation119] also demonstrated from a cohort study of 11,833 samples that holoTC had the highest diagnostic accuracy for recognizing B12 insufficiency, with significantly higher diagnostic accuracy than B12 and tHcy. The sensitivity of holoTC was found to be between 0.55–0.87 vs 0.33–0.76 for total B12, whilst the specificity ranged from 0.50–0.96 and 0.41–0.98 for holoTC and B12, respectively, in various studies summarized by Golding [Citation118]. The variations in sensitivity and specificity values largely depended on the MMA cutoffs used, which served as the proxy gold standard marker representative of metabolic vitamin B12 deficiency in these studies. Most of these studies demonstrated only a slightly higher sensitivity and specificity for holoTC than total B12. Conversely, the correlation coefficient (r), which reflects the relationship between holoTC and B12, ranged from 0.42 to 0.882 in twenty-two studies summarized by Golding in his review [Citation118]. A high correlation over a wide range of values may imply that holoTC could not detect the onset of B12 deficiency earlier than total B12 [Citation118]. However, separate r values for low and high B12 were not provided in most of the studies. Herrmann and Obeid [Citation120] additionally reported r = 0.524 for low total B12 <300 pmol/L and 0.403 for values above ≥300 pmol/L. The authors concluded that total B12 was a poor predictor of holoTC, and low holoTC occurred at higher total vitamin B12 concentrations [Citation120]. The r value (Spearman’s) based on randomly selected, consecutive diagnostic results from the authors’ laboratory (AS-M and DJH) was 0.741 (N = 298) across the whole holoTC and B12 range (unpublished data). Separate correlation analysis of holoTC <25 pmol/L (deficiency cutoff) with total B12 gave r = 0.404 (N = 175) and for holoTC ≥25 pmol/L, r = 0.627 (N = 123) ( and ). Assuming B12 deficiency based on holoTC <25 pmol/L, 56.0% of patients would have been classified as sufficient if the serum total B12 test with a cutoff of 138 pmol/L had been used ().
Figure 4. The correlation between holoTC and serum B12 in the low holoTC range, <25 pmol/L. The horizontal line on the y-axis represents the serum B12 deficiency cutoff of 138 pmol/L used in the authors’ laboratory (AS-M and DJH).
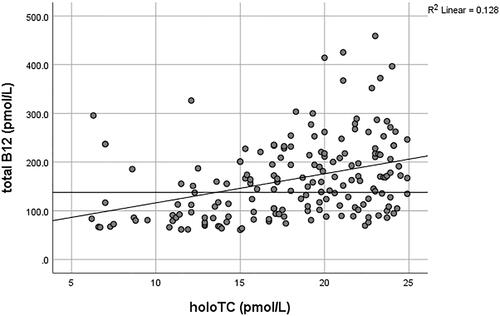
Figure 5. The correlation between holoTC and serum B12 in the holoTC range ≥25 pmol/L. The horizontal line on the y-axis represents the serum B12 deficiency cutoff of 138 pmol/L and the vertical line represents the holoTC cutoff of 70 pmol/L for B12 replete patients as used in authors’ laboratory (AS-M and DJH). Samples with holoTC results between 25–70 pmol/L are referred for additional testing.
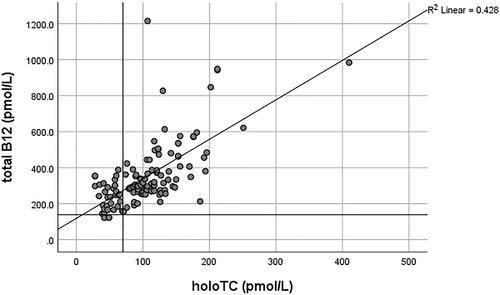
In addition, holoTC has been shown to be unaffected by assay interference from high-titer IF antibody levels [Citation121]. Furthermore, holoTC is not subject to the ∼50% fall as seen for total B12 in pregnancy [Citation122]. The diagnostic utility of holoTC in other cohorts requires further study and evaluation.
The holoTC assay is useful when transcobalamin and haptocorrin deficiencies are suspected (). In haptocorrin deficiency, holoTC concentration is normal/high while total serum B12 (the sum of holohaptocorrin and holoTC) can indicate severe deficiency. In haptocorrin deficiency, the serum B12 test measures the holoTC fraction only. Without the availability of holoTC testing, a clinician may wrongly diagnose a patient and incorrectly prescribe B12 replacement for an otherwise benign condition. In transcobalamin deficiency however, early treatment can lead to a good clinical outcome. In this condition, holoTC is unmeasurable since deficiency of the transcobalamin protein does not allow for B12 binding and the formation of holoTC. Ultimately, genetics analysis can confirm the diagnosis of both conditions.
Table 1. The commonly seen vitamin B12 marker patterns in selected clinical scenarios.
Conversely, unmeasurable holoTC may rarely imply variants in the transcobalamin gene that interfere with assays such as “Active B12” [Citation123,Citation124]. For instance, the minor allele rs35838082 (p.R215W), which is rare in Caucasians with a minor allele frequency (MAF) of <0.01 but more common in South Asians (MAF ∼0.02) and those of African origin (MAF ∼0.25), was found to interfere with the “Active B12” test [Citation124]. HoloTC results in these patients are erroneously low (<5 pmol/L), despite all other B12 laboratory markers being normal and an absence of clinical symptoms [Citation124].
Transcobalamin receptor (TCblR/CD320) polymorphisms may also have an impact on holoTC concentrations. In an elderly population, 5% were found to have a heterozygous codon 88 GAG deletion [Citation125]. This is associated with proportionately more serum B12 bound to holoTC. Hence, holoTC might not be a marker of “true” intracellular B12 status in some individuals; an elevated holoTC may reflect decreased cellular uptake due to the GAG deletion.
Functional markers of B12 status
Plasma tHcy and serum MMA are very sensitive markers, especially for B12 insufficiency, but are also influenced by other conditions independently of B12 status. Most notably, renal impairment and hypothyroidism lead to elevations of tHcy and MMA, making these tests unreliable. MMA begins to accumulate from the early stages of renal impairment, leading to a decreased specificity for B12 deficiency. Urinary MMA/creatinine ratio (uMMA/C) may have diagnostic utility, due to the stable urinary excretion of MMA before severe renal failure [Citation126]. With a threshold of 1.45 µmol/mmol, the uMMA/C ratio has been associated with good diagnostic performance for B12 deficiency as a second line assay [Citation127].
High tHcy is also a strong indicator of folate deficiency, therefore concomitant assessment of both vitamins is required if tHcy is being utilized as a marker of B12 status. Postprandial and orthostatic variations, as well as the prompt separation of plasma following blood collection, are preanalytical factors that need to be addressed before a sample is collected for tHcy measurement [Citation128]. Usage of special tubes may be considered if separation of plasma within 1 h of blood collection is not viable [Citation128]. Bacterial overgrowth and hemoconcentration may additionally influence MMA concentrations. Both markers are age-dependent, and tHcy is higher in males.
All the above factors need to be considered when interpreting B12 results (). A combination of tests for the assessment of B12 status is preferable, but tests need to be carefully chosen while considering factors affecting B12 markers independently of status. An understanding of the laboratory reference ranges, and using them as a guidance only rather than an indicator of “firm” B12 status, will also help to avoid misdiagnosing patients [Citation129].
Combined indicator of B12 status
If markers of B12 status do not show a “clear-cut” deficiency, the absence of a definitive diagnostic test can cause difficulty. Fedosov et al. [Citation130] proposed a solution to this diagnostic dilemma using a “combined” indicator of B12 status (cB12 or 4cB12). This is a rigorously derived mathematical model combining all four markers (total B12, holoTC, tHcy, and MMA), which makes adjustments for age and folate status. It yields one of five diagnoses: elevated B12, adequate B12, decreased B12, possibly B12-deficient, and probably B12-deficient [Citation131]. The interpretive comments from individual B12 markers combined vs the outcome from 4cB12 calculations in n = 44 patient samples analyzed in the authors’ laboratory (AS-M and DJH) showed 100% agreement in B12 status assessment (unpublished data).
The main drawbacks of 4cB12 are cost and the relative lack of availability of all tests in routine practice. A refined model now provides “missing markers” [Citation131], and although additional clinical research is required, this approach should be more widely appreciated. Our calculations using the 2cB12 formula (holoTC and MMA values only) for n = 3290 patients with holoTC 25-70 pmol/L showed that 1% of patients within this group were classified as possibly or probably B12-deficient (“need immediate intervention with intramuscular (IM) injections”), while 14% were classified as having decreased B12 (“start B12 supplements”). Using the MMA test as a confirmatory marker for this holoTC range in the same cohort yielded 24% of patients with elevated MMA [Citation116].
The cB12 model has currently not been validated for use in pregnancy, however, our unpublished results suggest its potential use [Citation132].
Considering groups at risk and potential causes for B12 deficiency/insufficiency
Once a diagnosis of B12 deficiency/insufficiency is made, it is incumbent on the physician to determine potential causes of deficiency/insufficiency, an important but often overlooked stage. Dietary and family history, pregnancy, age, concomitant nutrient deficiencies (folate and iron in particular), malabsorption, surgery, bacterial overgrowth, or pharmaceutical interactions should all be considered or investigated, as appropriate. This will determine the appropriate type, and duration, of treatment.
Restricted dietary intake (veganism/vegetarianism)
Vegetarian and vegan diets have become very popular in the last few decades, especially in developed countries, in view of their potential benefits to prevent coronary heart disease, cancer, and type 2 diabetes, as well as due to ethical and environmental issues [Citation133]. However, these diets have restricted the intake of certain nutrients, including vitamin B12. Also, non-vegetarians in the developing world can have low vitamin B12 intake due to the high cost of meat in these areas [Citation134]. In addition, in countries such as India or Bangladesh, vegetarianism has been practiced for centuries for religious and cultural reasons [Citation135] and it is well documented that the prevalence of vitamin B12 deficiency is high in these regions. Both vegans/vegetarians are unlikely to achieve recommended dietary allowances, since plant derived foods have no, or trace, B12 contents [Citation136,Citation137]. A certain amount of B12 may be provided by plants contaminated with B12-producing bacteria through fertilization with manure [Citation135], since feces are a good source of vitamin B12 [76]. It was also observed that in largely vegetarian populations in places where hygiene standards might be low, poor people had a better B12 status than the urban middle-class. This is possibly due to microbial vitamin B12 from ingestion of contaminated food and water. Using 150 pmol/L as threshold, 81% of urban middle-class men had low vitamin B12-concentration compared to 51% of slum residents [Citation138].
In a European study of 29 vegans, 66 lacto-vegetarians or lacto-ovo-vegetarians, and 79 omnivores who were not taking supplements, vegans had the lowest B12 status; 92% had holoTC <35 pmol/L and 83% had MMA >271 nmol/L [Citation139]. The prevalence of low holoTC and high MMA was also high in lacto-vegetarians and lacto-ovo-vegetarians (77% and 68%, respectively) compared to omnivores in this study (11% and 5%, respectively) [Citation139]. Therefore, dietary supplements are essential to vegans and should be considered by those with poor intake of milk and milk products.
The choice of supplement should also be carefully considered, since the B12 content of dietary supplements can vary greatly. When the B12 content was determined in 19 dried Chlorella cells, which are used in dietary supplements, it varied from <0.1 µg/100 g to 415 µg/100 g of dried weight [Citation140]; as Chlorella does not synthesize B12 and is instead dependent on symbiotic microorganisms, their content is greatly variable [Citation141]. Chlorella represents a group of eukaryotic green microalgae. Further analysis by liquid chromatography-tandem mass spectrometry (LC-MS/MS) showed the presence of inactive corrinoid compounds, a cobalt-free corrinoid, and 5-methoxybenzimidazolyl cyanocobamide in four and three high B12-containing Chlorella tablets, respectively. In four Chlorella tablet types with high and moderate B12 content, Ado-Cbl (∼32%) and Me-Cbl (∼8%) were present, whereas the unnaturally occurring corrinoid cyanocobalamin was present at the lowest concentrations. Chlorella sorokiniana is commonly used in dietary supplements and does not have a requirement of B12 for growth despite B12 uptake from the medium being observed. In further experiments, B12-dependent MCM and MS activities were detected in cell homogenates. These results suggest that B12 contents of Chlorella tablets reflect the presence of B12-generating organic ingredients in the medium or the concomitant growth of B12-synthesizing bacteria under open culture conditions [Citation140].
Pregnancy state
Balanced nutrition during pregnancy is essential for the mother’s and offspring’s health status. Most importantly, pregnant women are at high risk of developing deficiency as the requirements for vitamin B12 are exceptionally high during pregnancy as a result of increased metabolic rate and fetal demands. Studies conducted on maternal-fetal dyads demonstrate higher cord blood B12 concentrations than those of the respective mothers and a direct relationship between the two pools [Citation142–145].
Moreover, maternal nutrition before and during pregnancy is the main determinant of the nutritional status of the offspring. Layden et al. [Citation146] demonstrated that not only the mean cord blood B12 concentration correlated to that of the mother at delivery, but it was almost tripled (maternal: 230.9 pmol/L, cord blood: 662.1 pmol/L, p < .0001), confirming fetal dependency on good maternal B12 status. Low cobalamin intake prior to and during pregnancy has been related to increased risk of preterm delivery, low birth weight [Citation147,Citation148], and lower colostrum and early milk B12 contents [Citation149,Citation150]. Importantly, the deficient stores, resulting from inadequate B12 intake during pregnancy, have been shown to reflect on the newborn’s hepatic B12 stores [Citation17]. Vitamin B12 deficiency has also been suggested as a cause of recurrent spontaneous pregnancy loss [Citation151].
Pregnancy is probably the most challenging state for the diagnosis of B12 deficiency, since all B12 markers are affected due to physiological and hormonal changes during pregnancy while B12 status is also declining. Decades ago, observational studies of apparently healthy pregnant women with a normal hematological pattern and megaloblastic anemia showed a fall in serum B12 concentration during pregnancy but that pre-pregnancy concentrations resumed soon after delivery [Citation152–154]. In one study in the early 1960s, 33% of women had B12 concentrations below 147 pmol/L compared with only 17% of non-pregnant controls [Citation153]. In another study conducted five decades ago and involving 518 patients, 70% of pregnant women had serum B12 concentrations below the normal value for non-pregnant subjects (110 pmol/L), including 20% of women with exceptionally low concentrations <37 pmol/L (L. Leichmannii assay was used) [Citation152]. The question was raised whether this fall in circulating B12 was the result of physiological processes or reflected true deficiency. To answer this question, Metz et al. [Citation154] compared serum tHcy and MMA in pregnant women who had low B12 (<150 pmol/L) vs women who had B12 concentrations within the reference range for non-pregnant women. Interestingly, they found no correlation between these biomarkers and serum B12, but they observed elevated MMA (>376 nmol/L) in one-third of women and elevated tHcy (>13.8 µmol/L) in only two women (3%). The authors concluded that there was a lack of evidence concerning the association of functional deficiency with low serum B12 status. The same publication suggested homocysteine as a valuable marker in assessment of B12 status during pregnancy. Pardo et al. [Citation155] later confirmed the above observations and conclusions. In short, these early studies indicated that using references ranges for non-pregnant women during pregnancy is specious and warrants the use of separate reference ranges. We now know that serum B12 concentrations decrease during pregnancy because of hemodilution and decreased synthesis of haptocorrin, making this test unreliable [Citation122]. HoloTC is considered a superior diagnostic tool in pregnancy as it is less impacted by pregnancy-related changes [Citation122,Citation143]. Hemodilution and hormonal changes have likewise been proposed as modulators of MMA and tHcy concentrations during pregnancy [Citation143]. In addition, folic acid supplementation, usually taken in the first trimester, influences tHcy concentration, making this marker less specific for B12 deficiency [Citation156]. lists the values for B12 markers measured in longitudinal studies in pregnant women, which were conducted more recently [Citation142–144,Citation157–159]. Although absolute values may differ, these studies unanimously show changes in B12 markers during pregnancy, which are likely to be a result of both pregnancy-related factors and declining B12 status. In addition, Milman et al. [Citation158] and Schroder and Tan et al. [Citation159] established pregnancy related reference intervals for some B12 markers for all trimesters and the first two trimesters, respectively. Serum B12 and MMA reference intervals were in good agreement between these studies. Schroeder and Tan et al. [Citation159] also showed differences between women of European and South-Asian descent, reporting change points based on MMA in pregnancy and interestingly finding that these were not ethnicity-dependent. Accordingly, total B12 <186 and <180 pmol/L and holoTC concentration <62.2 and <67.5 pmol/L in the 1st and 2nd trimesters, respectively, would indicate an increased risk of deficient B12 status.
Table 2. Selected serum/plasma results from longitudinal studies of uncomplicated pregnancies: pre-conception, pregnancy, labor, post-delivery and cord samples.
Newborns and infants
The mechanisms that determine B12 status in newborns are not well defined, particularly during the early breastfeeding period. During the immediate postnatal period, the gastrointestinal tract undergoes profound colostrum-dependent growth, morphological changes, and functional maturation, including the capacity of acid secretion [Citation160]. From binding studies of IF and of purified human milk HC using the immortalized enterocyte cell line Caco-2, and IF gastric secretion measured in terms of breastfed infant fecal extracts, Adkins et al. [Citation161] have shown IF-dependent B12 absorption mechanisms in breast-fed newborns. However, with IF concentrations appearing seemingly too low in this period of life to participate effectively in B12 absorption, they proposed that HC could take control until maturation of the IF component.
Importantly, the deficient stores resulting from inadequate B12 intake during pregnancy have been shown to reflect on the newborn’s hepatic B12 stores [Citation17]. Furthermore, exclusively breastfed infants are at risk of developing symptoms of deficiency, including anemia, irritability, failure to thrive, and developmental delays, often after five months of life if maternal B12 stores, and hence breast milk, remain low [Citation129,Citation162,Citation163]. Lower serum B12 and holoTC were found in breastfed infants of mothers with poor vitamin B12 status compared with breastfed infants receiving solid foods or solid foods/milk substitutes [Citation164]. Chandyo et al. [Citation165] reported that 11% and 24% of 6–11 month old breastfed infants (n = 316) were B12-deficient (<148 pmol/L) or marginally deficient (148–221 pmol/L), respectively. Interestingly, raised tHcy (>10 µmol/L) and MMA (>280 nmol/L) concentrations were observed in 53% and 75% of toddlers, respectively. They attributed these high frequencies to sub-optimal maternal nutritional status and the largely vegetarian additional feeding. Intervention clinical trials with 400 µg IM cobalamin showed a marked improvement in B12 markers (serum B12, tHcy, and MMA) of breastfed infants compared to controls, as well as an improvement in motor function, providing evidence that biochemical abnormalities seen in infants reflect vitamin B12 status in addition to immature metabolism and that long-term exclusive breastfeeding does not provide adequate B12 to the growing infant [Citation166–168]. A plasma homocysteine value of 6.5 µmol/L was suggested as the cutoff for defining impaired B12 function in infants [Citation168]. Therefore, infants suspected of poor development and having feeding difficulties should be screened for vitamin B12 deficiency before tests of the inborn errors of metabolism are carried out. Exclusive breastfeeding beyond 4–5 months of age should be another pointer for B12 assessment.
Malabsorption
Malabsorption leading to B12 deficiency is not only prevalent in the elderly; it can result from gastritis, intestinal diseases and infections, surgical resections, drugs, or it can have a genetic origin as described earlier. In B12-deficient patients, for whom no “obvious” cause is apparent and who are IF antibody negative, the capacity to absorb the vitamin should be established. Unfortunately, the traditional Schilling tests used for this purpose have been discontinued due to the safety factors related to use of radioactive B12. One option is the CobaSorb test based on changes in circulating holoTC before and after administrating oral B12 [Citation122]. Importantly, this test cannot be used once the patient is treated with B12 [Citation169]. Although the CobaSorb test has a potential diagnostic use, poor availability of holoTC measurements in many clinical settings, its dynamic design, as well as a lack of interpretative knowledge hinder its wider use.
Ageing
Malabsorption due to autoimmune disease or gastritis is the main cause of B12 deficiency in the elderly, followed by inadequate dietary intake. For example, in a French study of 172 elderly patients with B12 deficiency, 2% of the cases were a consequence of inadequate intake [Citation170]. Atrophic gastritis is the main cause of dietary cobalamin malabsorption in the elderly and its prevalence increases with age [Citation170,Citation171] from as much as 24% in those aged 60–69 years to 37% in those aged >80 years [Citation172].
The incidence of B12 deficiency increases overall with age. In one longitudinal study, it was estimated that the mean annual decline in serum B12 is 3.4 pmol/L for men and 3.2 pmol/L for women [Citation173]. Estimates of B12 deficiency of 1.6% were found in subjects over 51 years [Citation174]. Five percent of deficiency was estimated in people 65–74 years and more than 10% in people 75 years or older (based on serum B12 <150 pmol/L or B12 <150 pmol/L and tHcy >20 µmol/L) [Citation175]. In the same population-based cross-sectional study of people living in the UK, it was found that folate deficiency also increased with age, but only about 10% of people with low B12 concentrations also had low folate concentrations [Citation175]. This is relevant in view of the findings that elderly people who have high folate concentrations (>45.3 nmol/L) accompanied by low B12 (<148 pmol/L) have higher metabolic evidence of B12 deficiency than when folate is normal [Citation176]. This has a potential implication for vitamin B12 status, especially in the countries where mandatory folic acid has been implemented but not B12 fortification.
The prevalence of vitamin B12 deficiency in the elderly may be even higher in other countries. Using a serum B12 cutoff of 220 pmol/L, an Iranian study found that 56% and 93% of people aged 65–74 and ≥ 75 years, respectively, were at high risk of B12 deficiency [Citation177].
The mechanisms behind decreasing B12 status in the elderly are not known. However, decreases of B12 with age were also found in total brain levels, particularly decreases in Me-Cbl that were observed in the frontal cortex [Citation178]. The authors attributed these to changes in activity to one or more transport processes across the lifespan, such as transport across the choroid plexus via megalin. Equally, cognitive deficits have been observed in elderly people who are well-nourished but have low B12 status [Citation179]. For more information on cognition, the reader is referred to the vitamin B12, cognition, and dementia section below.
Autoimmune disease and gastritis
Some cases of pernicious anemia (PA) can be described as the end stage of autoimmune gastritis (AIG), in which parietal cell antibodies (PCAs) lead to the destruction of IF-producing parietal cells in the stomach, leading to B12 malabsorption and, consequently, deficiency [Citation180]. The prevalence of PA in the general population is 0.1%, increasing to 1.9% in those over the age of 60 [Citation59,Citation181]. Clinical features often include anemia, thrombocytopenia, neutropenia, glossitis, cardiomyopathy, jaundice, weight loss, and neurological symptoms [Citation180]. PCAs are detected in more than 90% of patients with PA. However, these antibodies are not only specific to PA and are found in other autoimmune conditions that often occur concomitantly with PA, such as Graves’ disease, hypothyroidism, thyroiditis, and Addison’s disease [Citation180]. Two types of antibodies related to IF have been detected in the sera of PA patients: type I blocks the binding of B12 to IF (found in 70% of PA patients); type II prevents the attachment of IF or the IF-B12 complex to ileal receptors (in ∼40% of PA). Antibodies to vitamin B12 binding proteins have also been reported [Citation182]. These antibodies lead to high serum B12 and holoTC concentrations, and they have not only been seen in cases of B12 injections but also in patients not receiving B12 supplements [Citation183]. The presence of these antibodies does not appear to interfere with the binding of B12 to TC, but they may impair the ability of cellular delivery. One study has shown that the ability to deliver B12 to cells in vitro was impaired and clearance of B12 in vivo of B12 was abnormal in the presence of these TC antibody complexes [Citation183]. Precipitation of these complexes with polyethylene glycol resulted in the normalization of serum B12 concentrations in many patients with autoimmune diseases, hematological disorders, and plasma cell neoplasms [Citation182]. Although the presence of these complexes is thought not to be pathological, one needs to be aware of them, especially in patients with normal/high B12 concentrations and clinical signs of deficiency [Citation180,Citation182]. Development of diagnostic methods for the presence of immune complexes would help with the assessment of vitamin B12 status.
Intestinal diseases and infections
Hypochlorhydria associated with atrophic gastritis hinders the release of B12 from food and additionally causes intestinal bacterial overgrowth. The bacteria might compete for the available B12, contributing further to B12 deficiency [Citation184,Citation185].
Additionally, Helicobacter pylori infection in the intestines, which is highly prevalent in the general population, predisposes individuals to PA through inducing autoantibodies to antigens in the gastric mucosa. This effect is observed in half of infected patients, suggesting that gastric atrophy may be caused by a Helicobacter pylori driven autoimmune process [Citation180,Citation186].
Some patients develop B12 deficiency as a result of conditions that slow the movement of food through the intestine, allowing intestinal bacteria to multiply and overgrow in the upper part of the small intestine. These bacteria utilize vitamin B12 for their own metabolism, rather than allowing it to be absorbed by the body. Parasites, most notably the fish tapeworm, also lead to B12 malabsorption since they consume B12 for their own needs [Citation187].
Patients with ileal Crohn’s and Celiac disease will develop vitamin B12 deficiency because of reduced absorption as a result of villous atrophy and mucosal impairment, respectively. One study has found B12 deficiency in 33% of patients with Crohn’s disease compared to 16% of patients with ulcerative colitis [Citation188]. The study also showed that the serum B12 test was insensitive during B12 deficiency detection in these patients, as only 5% of the cases were deficient using this marker, compared to 32% using holoTC/MMA in 89 patients who underwent paired testing [Citation188].
Patients suffering from chronic pancreatitis also have affected vitamin B12 absorption since pancreatic enzymes are required for the release of cobalamin from R-binders [Citation189]. Other intestinal disorders affecting vitamin B12 include tropical sprue, intestinal lymphoma, amyloidosis, and short bowel syndrome [Citation32].
In HIV infected patients, B12 deficiency is also quite common. The causes of this deficiency can be ileal dysfunction, diminished IF secretion, or IF antibodies [Citation190].
Surgery
Surgery involving any part of the gastrointestinal tract will lead to diminished absorption of vitamin B12 and, consequently, B12 deficiency in some patients. About 30% of patients following a partial gastrectomy will develop vitamin B12 insufficiency [Citation32]. Vitamin B12 deficiency was found in 48% and 65% of patients with ileal resections of ≤20 cm and >20 cm, respectively [Citation188].
B12 deficiency is also common in patients undergoing bariatric surgery, causing poor absorption of food-bound B12 postoperatively. The prevalence of deficiency following bariatric surgery varies between studies but may depend on the type of surgery. The highest prevalence of B12 deficiency, ranging from ∼35% to 75%, was reported following Roux-en-Y gastric bypass (RYGB), as no gastric acid remains in the gastric pouch [Citation191], compared to post-sleeve gastrectomy (SG) [Citation47]. It was also recently found that MMA increased as early as two months following surgery, and holoTC decreased in patients following RYGB and SG, indicating a negative B12 homeostasis immediately following surgery [Citation47]. However, changes in serum B12 were observed after 6 months in this study indicating that holoTC and MMA may be more appropriate for monitoring B12 status post-bariatric surgery.
Surgical anastomoses, which create gastrointestinal cul de sacs, can lead to severe bacterial overgrowth [Citation192] and, consequently, B12 inadequacy.
Pharmaceutical interactions
Metformin
Metformin is a medication for the treatment of type 2 diabetes (T2D), prediabetes, and polycystic ovary syndrome. It is a drug known to affect mitochondrial function and glucose metabolism [Citation193]. A range of evidence since 2003, including observational, experimental, and meta-analyses, suggests that the use of this oral hypoglycemic medication interacts negatively with and leads to a marked reduction in B12 status [Citation194,Citation195]. However, the associations between B12 levels and metformin appear not to be linked to the duration of therapy and dosage [Citation196]. Not many metformin-related studies have used functional markers of B12 status. In those that have, elevations in tHcy and MMA were found in addition to low serum B12 concentrations [Citation197]. In other studies, no difference or lower MMA was found in T2D patients using metformin [Citation198,Citation199].
Although it is widely accepted that metformin induces malabsorption of B12 via inhibition of the Ca+-dependent binding of the IF-Cbl complex to cubam receptors in the ileum (an effect that can be reversed by increasing calcium intake [Citation200]), other mechanisms affecting B12 status are also possible. Notably, Liu et al. [Citation201] showed that adaptations in mitochondrial substrate utilization underlie metformin sensitivity/resistance and can result in perturbations in the tricarboxylic acid cycle and ATP production, generation of reactive oxygen species, and alterations to nucleotide/lipid synthesis. It was also shown that genetic variation in HIBCH, MUT genes, as well as genes encoding for proteins/enzymes in the branched chain amino acids pathway, affected the mitochondria as a direct consequence of metformin exposure [Citation201]. We suggest that current data support the view that patients on long-term metformin treatment should have their functional B12 status checked at regular intervals.
Protein pump inhibitors and H2-receptor antagonists
Proton pump inhibitors (PPIs), such as omeprazole, lansoprazole, and esomeprazole, and H2-receptor antagonists, such as ranitidine, cimetidine, famotidine, and nizatidine, are used to reduce gastric acid secretion for the treatment of gastroesophageal reflux disease and peptic ulcers [Citation194]. Similar to metformin, associations of these drugs with lower serum B12 have been found. However, none of these studies were a randomized controlled trial, nor did they assess changes in B12 markers or the onset of clinical indications of deficiency [Citation194]. A higher incidence of B12 deficiency has been found in older adults using PPIs for more than 12 months in case-control studies of [Citation202,Citation203]. The same was not concluded in a study of elderly patients taking PPIs for more than three years [Citation204]; however, the cross-sectional design of this work, as well as a slightly higher proportion of controls positive for Helicobacter pylori infection, albeit not significant (p = .09), may have accounted for the lack of difference in B12 status between PPI users and controls. The use of omeprazole in patients with duodenal ulcers with concurrent Helicobacter pylori infections augments the pH-increasing effect of PPIs [Citation205].
Genetic polymorphisms that diminish the microsomal cytochrome P450 driven catabolism of omeprazole have been shown to have an impact on serum B12 concentration [Citation206,Citation207]. Those with a heterozygous mutation in the CYP2C19 polymorphism with a prevalence of 47% in Orientals and 30% in Caucasians had much lower B12 concentrations compared to homozygotes (wt/wt) after >1 year therapy with 20 mg omeprazole daily [Citation206]. Drinking acidic fruit juice concurrently with B12 may improve B12 absorption in PPIs users [Citation208].
Oral contraceptives
Oral contraceptives (OCs) are among the most commonly used drugs in developing countries. They frequently contain both estrogen and progestin or progestin-only pills. The serum concentration of B12 has consistently been reported as lower in OC users when compared to nonusers [Citation209]; however, the values were still within normal limits in most women [Citation210]. The findings were the same for users of the combined pills [Citation209,Citation211] as well as progestin-only pills [Citation210]. Lower vitamin binding capacity and lower haptocorrin levels have been suggested as potential causes for lower B12 in OC users [Citation209]. It has been hypothesized that hormones used in OCs may affect the synthesis of haptocorrin, since the same is observed in pregnancy as well [Citation209]. Some studies also measured holoTC in addition to total B12; in one cross-sectional study of 264 females, holoTC was found to be 25% lower in OC users than in controls [Citation211]. The women in this study were most frequently on combination tablets containing the synthetic estrogen ethinylestradiol and the progestogens, levonorgestrel or drospirenon [Citation211]. The proportional decreases in both cobalamin markers in this study indicates that cobalamin bound to HC and to TC was equally affected in OC users. However, the same group has previously shown that total TC is not significantly lower in OC users [Citation212]. Hence, the mechanism for the observed decrease in plasma holoTC is not clear and warrants further investigation.
Consequently, it seems reasonable to take OC usage into consideration when interpreting B12 markers, especially total B12 and holoTC. Nonetheless, this fall in abundance does not appear to reflect a functional deficient state, as no differences in tHcy or MMA have been observed [Citation211]. Therefore, redistribution of B12, rather than depletion of intracellular cobalamin, has been proposed in OC users [Citation211].
Nitrous oxide
Nitrous oxide (N2O) is commonly used for sedation and pain relief, but it is also abused. The gas is also a food additive when used as a propellant for whipped cream, hence its low price and easy access [Citation213]. The gas is inhaled, typically by discharging N2O cartridges into another object, such as a balloon, or directly into the mouth. Empty silver canisters of N2O have become a common sight not only outside night clubs but also on the streets in residential areas in the UK ().
Nitrous oxide oxidizes the active cobalt atom of cob(I)alamin from the 1+ to the 3+ valence state at a high rate, outperforming the reductive recovery system via cblC and MS-reductase [Citation214,Citation215]. This leads to a stable shift toward cob(III)alamin, followed by dissociation of Cbl from MS and inactivation of apo-MS () [Citation216]. Inactivation of the MS enzyme will lead to the impairment of homocysteine remethylation to methionine and, subsequently, all methylation reactions become affected due to a low level of SAM. In contrast, the second Cbl-dependent enzyme, MCM, is unaffected by N2O from the start, because MCM does not generate cob(I)alamin but instead generates cob(II)alamin, which is insensitive to N2O. However, long-term abuse of N2O leads to repeated reductions and oxidations of Cbl, giving off reactive oxygen species upon oxidation (e.g. H2O2 [Citation214]). These reactive molecules can convert Cbl to inactive “stable yellow corrinoids” with a broken pattern of the conjugated bonds [Citation217]. Such degradation of Cbl causes gradual inactivation of MCM as well. Therefore, biochemically, highly elevated tHcy is seen first in patients who abuse N2O, followed by a slow decrease in serum B12 and holoTC, as well as slight elevations in MMA. Serum B12 is often normal at presentation [Citation218,Citation219], which may lead to an incorrect differential diagnosis. It has been also suggested that inactive Me-Cbl is converted to Ado-Cbl [Citation216].
Some of the short-term effects of N2O include euphoria, numbness, sedation, giddiness, uncontrolled laughter, uncoordinated movements, blurred vision, confusion, dizziness and/or lightheadedness, sweating, and tiredness. If taken in large doses, N2O can cause loss of blood pressure, heart attack, or death due to hypoxia. Long-term effects lead not only to severe B12 deficiency but also memory loss, myelopathy, incontinence, demyelinating polyneuropathy, subacute combined degeneration, limb spasms, weakened immune system, disruption to reproductive systems, depression, and psychosis [Citation213,Citation218]. In the authors’ hospital, which is located in central London, N2O poisoning cases are seen regularly. Most patients are in their early twenties and often present to the A & E department with lower leg weakness and sensory loss, urinary incontinence, tingling in the fingertips, and difficulty walking. Their plasma tHcy is frequently >100 µmol/L. Some patients reported ordering canisters off the internet in bulk and taking ∼100 canisters when at a party or an event. A marked improvement in neurological symptoms is often seen following IM hydroxycobalamin injections and homocysteine normalization.
A single use of N2O for anesthetic purposes should not pose a health risk; however, more work may be required to confirm this since some studies suggested possible adverse health effects. In a study by Zanardo et al. [Citation220] both plasma homocysteine of mothers and cord blood of the respective offspring were higher in 25 women undergoing an elective cesarean section under nitrous oxide general anesthesia than in 25 undergoing vaginal delivery. Moreover, maternal homocysteine levels significantly correlated with cord levels of cesarean and vaginally delivered neonates (r = 0.57; p < .01 and r = 0.66; p < .001, respectively) [Citation220].
In a different study involving 394 patients, postoperative hyperhomocysteinemia was associated with an increased risk of major complications with a risk ratio (RR) of 2.8 (95% CI: 1.4–5.4, p = .002) and cardiovascular events, with an RR of 5.1 (95% CI: 3.1–8.5, p < .0005) in in the N2O group [Citation221].
Data on occupational exposure to N2O is scarce. One study reported increased oxidative DNA damage in medical personnel of operating theaters. The extent of genetic injury was especially evident among nurses and anesthesiologists exposed to N2O in concentrations exceeding occupational exposure limits (180 mg/m3) [Citation222].
Treatment and prevention regimens
The treatment choice for clinical deficiency depends on whether there is neurological involvement; a specialist should manage such patients. If a specialist is not immediately available, 1 mg of hydroxocobalamin should be given intramuscularly on alternate days until there is no further improvement, then intramuscularly every 2 months [Citation223]. For those without neurological involvement, 1 mg hydroxocobalamin should be administered intramuscularly three times a week for 2 weeks to replenish stores. This should result in retention of up to 3–4 mg of B12, sufficient to bring the body’s stores to within the normal range [Citation33]. On-going maintenance dosage depends on whether the deficiency is diet-related. For patients with deficiency that is not of dietary or drug-related origins, but caused by life-long malabsorption, one should continue with 1 mg intramuscularly every 2 months for life [Citation223].
Patients who have undergone bariatric surgery are recommended oral, sublingual, or liquid B12 with 350–1000 µg daily, as well as nasal sprays as directed by manufacturer or parenteral (IM or subcutaneous) with 1000 µg monthly prevent deficiency [Citation224]. A 1000 µg/d of B12 to achieve normal levels is recommended for bariatric patients with deficiency [Citation224]. An oral vitamin B12 with 350 μg deemed appropriate to correct low B12 concentrations in many patients following bariatric surgery [Citation225].
Those whose deficiency is likely diet-related can consider oral supplementation with 50–150 µg B12 daily or have a twice-yearly 1 mg hydroxocobalamin injection [Citation223]. In severe cases, one could also consider commencing treatment with several injections to replenish B12 stores. In vegans, treatment may need to be life-long. In others, replacement can be stopped once B12 levels are corrected and the diet has improved; it is important to give dietary advice about foods rich in B12 such as meat, fish, eggs and dairy products.
If there are doubts regarding the formal diagnosis of deficiency, there is little to be lost, and much to be gained, by instigating a trial of replacement. Alternative administration routes (see further) can be considered instead of injections to keep costs low [Citation226].
Dosage, formulation, and schedule of administration vary between countries, probably reflecting a paucity of randomized research fulfilling the strict criteria of evidence-based medicine [Citation59]. Cyanocobalamin and hydroxocobalamin are the most commonly used formulations, but methylcobalamin and adenosylcobalamin are also available, especially via the internet. In some countries, the market has been flooded with the methylcobalamin form of vitamin B12, promulgating it as the preferred formulation for treatment [Citation227]. However, using unstable photo-sensitive methylcobalamin and adenosylcobalamin may not be advantageous over cyanocobalamin and hydroxocobalamin, as they are likely to be converted to hydroxocobalamin in vivo during intracellular processing. The newly internalized alkylcobalamins (i.e. methylcobalamin and adenosylcobalamin) probably undergo dealkylation by the enzyme MMACHC (cblC) [Citation50]. It is therefore likely that these forms of B12 are no superior to cyanocobalamin in daily oral therapy regimes [Citation228].
Moreover, the solvent vehicle the vitamin is dissolved in can have an impact on treatment frequency. It was shown that cyanocobalamin in an oily suspension (Betolvex) had a longer treatment response than cyanocobalamin in aqueous solution (Cytobion) [Citation229].
Cyanocobalamin and hydroxocobalamin have differing pharmacokinetic properties. Hydroxocobalamin is retained far more efficiently than cyanocobalamin. It disperses more slowly from the injection site, binds more strongly to plasma, and has a slower diffusion rate than cyanocobalamin [Citation230–232]. Hence, cyanocobalamin is administered at 1000 µg once a month, while the same dose of hydroxocobalamin can be administered every two to three months. Treatment for PA in France involves daily administration of 1 mg of cyanocobalamin for one week, followed by 1 mg per week for 1 month, and then by monthly 1 mg injections for life [Citation59]. In some other countries, such as Sweden and Canada, a high oral dose is the treatment of choice [Citation233]. Oral doses ≥500 µg per day of cyanocobalamin have been found effective at treating B12 deficiency, as ∼1% of this quantity is absorbed via passive diffusion [Citation30]. A recent pragmatic randomized multi-center trial of the effectiveness of oral vs intramuscular B12 in elderly deficient patients showed that 1 mg oral administration was no less effective than IM administration at 8 weeks, although small differences were found between administration routes after 1 year (73.6% achieved normal B12 in the oral arm vs 80.4% in the IM arm [Citation234]. This study confirms the findings of other earlier studies, which found that oral cobalamin treatment with large vitamin B12 doses (1–2 mg daily) was as effective as IM cobalamin treatment [Citation235,Citation236].
Vitamin B12 administered sublingually and intranasally has also been shown to be effective at treating B12 deficiency [Citation237,Citation238]. A sublingual dose of 50 µg/d (350 µg/week) of cobalamin, instead of the commonly used 2000 µg/week provided in a single dose was suggested by Del Bo et al. [Citation239] to reach a state of nutritional adequacy of B12 in vegetarians and vegans.
A recent survey found that one in ten of Pernicious Anemia Society members resort to unlicensed formulations to “supplement” their prescribed treatment regime [Citation92]. These include nasal-sprays, sublingual drops and sprays, transdermal patches, suppositories, self-administered subcutaneous injections, and even intravenous infusions. None can be deemed to be any superior, as there is very little evidence base for these preparations.
It is noteworthy that early publications concerning parenteral B12 refer to IM and subcutaneous routes as modes of administration. Self-administered B12 via subcutaneous injection should perhaps be explored as an alternative to current treatment regimes. This would significantly reduce costs and undoubtedly benefit developing countries where deficiency is highly prevalent but nursing care is scarce. However, there is an inadequate research base differentiating between “IM” and “subcutaneous” routes, and further work is required to fully evaluate their relative efficacies.
Many people choose to supplement with vitamin B12 as a conscious health decision, and supplementation of vitamin B12 has been shown to be well-tolerated without significant adverse effects when consumed by healthy adults or B12-deficient patients [Citation240]. The elderly, vegans, and vegetarians may likely benefit from voluntary supplementation. Vitamin B12 supplementation is also highly recommended during pregnancy; however, the recommendations for the dose and the duration have not been fully validated. Doses of 50 µg and 250 µg daily have been effectively used in clinical trials in countries with a high prevalence of vitamin B12 deficiency [Citation149,Citation150], but whether a physiological dose of B12 will be protective against deficiency during pregnancy remains to be established [Citation241]. In their study in Indian lactovegetarians with low vitamin B12, Naik et al. [Citation242] have shown that the physiological dose of B12 (2 × 2.8 µg/d) for eight weeks cannot provide a fast replenishment of cobalamin stores and restoration of a normal metabolic status.
Considering associated risks
It is beyond the scope and size of this review to address all the potential risks associated with low B12 status thus far. Here we can count (i) hyperhomocysteinemia, which results from vitamin B12 deficiency among other causes; (ii) its impact on disease risk [Citation243] and; (iii) cobalamin interactions with folate [Citation244].
Importantly, in a hospital setting, there are twice as many patients with high serum B12 than with its low concentrations [Citation116]. Whilst many of these patients are on B12 supplements, some cases of high B12 can be indicative of disease state/risk [Citation245,Citation246].
Only the selected health risk/diseases will be discussed in the following section.
Vitamin B12, PA, and cancer risk
Pernicious anemia is considered to be a premalignant condition. Some tumors in PA patients arise as a consequence of gastric atrophy, and subsequently, the prolonged elevated gastrin levels have a trophic effect on cells that gastric tumors originate from [Citation247]. Patients with PA have a significantly increased risk of gastric carcinoid tumors, adenocarcinomas, tonsillar cancer, hypopharyngeal cancer, esophageal squamous cell carcinoma, small intestinal cancer, liver, myeloma, acute myeloid leukemia, and myelodysplastic syndrome, but a lower risk of rectal cancer [Citation247]. Based on their study in PA patients, Kokkola et al. [Citation248] confirmed a high risk for gastric carcinoids in this patient group, though discrepancies exist regarding advice about regular gastroscopic follow-up of PA patients [Citation247]. However, it would be prudent to consider thrice-yearly endoscopic surveillance of those PA patients with a family history of gastric cancer, or advanced age (≥70 years), pending further prospective cohort studies and cost effectiveness analyses [Citation249].
It is unknown if vitamin B12 deficiency contributes to cancer risk by affecting DNA synthesis and methylation potential (via the remethylation pathway), or if an excess of cobalamin is associated with cancer risk. Similar mechanisms were purported in folate deficiency and excess in relation to cancer risk [Citation244]. The associations of high B12 with cancer or liver diseases have been explained by a release of HC from proliferating leukocytes/malignant tissues/damaged hepatocytes, as well as by an increased synthesis or decreased clearance of TC and HC proteins [Citation245,Citation246], hence high B12 concentrations are accumulated in blood. A screening strategy for addressing high serum B12 values was proposed [Citation246]; however, this strategy did not suggest using cobalamin as a diagnostic marker for cancer, but only suggested following it, if unexplainable high concentrations were encountered. A value of 1000 pmol/L has been suggested as a cutoff for high serum/plasma B12 concentrations if the elevated level did not originate from regular intakes of high vitamin doses [Citation246]. Importantly, Arendt et al. [Citation250] showed that high plasma B12 increased the risk of subsequently diagnosed cancer, mostly within the first year of follow-up. Moreover, a recent large study, which used pre-diagnostic biomarker data from 5183 case-control pairs nested within 20 prospective cohorts and genetic data from 29,266 cases and 56,450 controls, found that higher vitamin B12 concentrations were positively associated with overall lung cancer risk, with the authors concluding that high vitamin B12 status increases the risk of lung cancer [Citation251]. Of note is the fact that patients with values of B12 >850 pmol/L were excluded from analysis in this study.
Vitamin B12, cognition, and dementia
B12 deficiency can present as “cognitive impairment” in some individuals and is a recognized cause of reversible dementia [Citation252]. Its relative contribution to the etiology of specific dementias, including Alzheimer’s disease (AD), is of high interest given the dearth of current prophylaxis for dementias. Associations between B12 deficiency and AD emerged in the 1980s [Citation253,Citation254], and it was unclear whether the two were definitely related. The discovery of low B12 concentrations in a family with genetically determined AD confirmed a genuine association [Citation255]. The advent of homocysteine as a marker for B12 deficiency resulted in an avalanche of literature describing its association with AD, vascular dementia, and other neurological disorders [Citation243,Citation256]. Lowering homocysteine with high dose B vitamins, including B12, has proven effective in slowing cognitive decline and brain atrophy [Citation257,Citation258]. Credible mechanisms underlie the association, which fulfills Bradford-Hill’s criteria suggesting causality [Citation259]. Further trials are needed, but the physician should be especially alert to middle-aged and elderly patients with insufficiency of B12, in order to potentially slow cognitive decline and perhaps avert or delay a devastating dementia.
Vitamin B12 and multiple sclerosis
Multiple sclerosis (MS) is an autoimmune neurodegenerative disease of the central nervous system leading to axonal loss and demyelination. Cobalamin deficiency is prevalent in MS patients, although not all studies, which used serum B12 as a deficiency marker, have demonstrated this, thereby questioning the role of B12 in MS [Citation260,Citation261]. Significantly higher unsaturated R-binder capacities were also found in MS, compared to patients with other neurological impairments or normal controls [Citation262]. It is possible that B12 deficiency, whatever its cause might be, could render the patient more vulnerable to the putative viral and/or immunologic mechanisms, which have been suggested as the pathogenesis of this disease [Citation260]. Conversely, chronic immune reactions or recurrent myelin repair processes in MS may increase the demand for vitamin B12 [262]. More importantly, Nijst et al. [Citation263] showed significantly lower B12 in cerebrospinal fluid (CSF) in MS patients than in reference patients, but the same was not seen for serum B12. Serum and CSF B12 correlated positively in 293 neurological patients, including 58 with MS in this study, but in individual patients, CSF B12 concentrations varied considerably for a given serum concentration in some patients. Of note is the fact that CSF B12 was very low, while serum B12 was normal, suggesting a blood-brain barrier transport defect [Citation263]. A resemblance of MS to PA was also suggested (despite age of presentation differences), because the sex, racial, and geographical distribution of MS and PA are similar [Citation264].
The studies using B12 supplementation in MS are scarce and the benefits of regular B12 supplementation to MS patients are yet to be demonstrated. For example, high methylcobalamin supplementation improved visual and brainstem auditory evoked potentials in one study [Citation265], while adenosylcobalamin injections restored memory and speech as well as improved balance and mobility in another study, though only one patient was involved [Citation266].
Conclusions and future directions
Despite significant advances in elucidating mechanisms of vitamin B12 absorption and intracellular processing, challenges remain in the diagnosis and prognosis of vitamin B12 deficiency/insufficiency, as well as the frequency and the type of treatment being administered. We recommend that robust assessment of B12 status should include at least two markers, especially in instances where discrepancies between values and clinical presentation exist, and the combined index cB12 may also be calculated. Finding the cause of deficiency before commencing treatment is detrimental to the future management of patients. In the event where treatment should be initiated promptly due to severe presentation, blood samples should be taken and saved for future analysis as appropriate.
The elucidation of the mechanism of B12 crossing the blood-brain barrier, a better understanding of the role of the microbiome and corrinoids on vitamin B12 status and their impact on markers of B12, validation of the CobaSorb or development of new absorption tests for use in diagnostic settings, as well as a better understanding of the impact of long term vitamin B12 injections on B12 status/markers as well as cobalamin’s role in cancer, MS, and dementia are needed to improve B12 diagnosis and health interventions.
Abbreviations | ||
AI | = | adequate intake |
AD | = | Alzheimer’s disease |
AIG | = | autoimmune gastritis |
CSF | = | cerebrospinal fluid |
Cbl | = | cobalamin |
Co | = | cobalt |
cB12 | = | combined indicator of vitamin B12 status |
CBLA | = | competitive binding chemiluminescence assays |
CN-Cbl | = | cyanocobalamin |
Ado-Cbl | = | 5’-deoxyadenosylcobalamin |
ELISA | = | enzyme-linked immunosorbent assay |
GWAS | = | genome-wide association studies |
HC | = | haptocorrin |
holoHC | = | holohaptocorrin |
holoTC | = | holotranscobalamin |
OH-Cbl | = | hydroxocobalamin |
IGS | = | Imerslund-Gräsbeck |
IM | = | intramuscular |
IF | = | intrinsic factor |
LC-MS/MS | = | liquid chromatography-tandem mass spectrometry |
MS | = | methionine synthase |
Me-Cbl | = | methylcobalamin |
MMA | = | methylmalonic acid |
MCM | = | methylmalonyl-CoA mutase |
5-MTHF | = | 5-methyltetrahydrofolate |
MAF | = | minor allele frequency |
MRP1 | = | multidrug resistance protein |
MS | = | multiple sclerosis |
NHANES | = | National Health and Nutrition Examination Survey |
N2O | = | nitrous oxide |
OC | = | oral contraceptives |
PCA | = | parietal cell antibodies |
PA | = | pernicious anemia |
PPIs | = | proton pump inhibitors |
RR | = | risk ratio |
RYGB | = | Roux-en-Y gastric bypass |
SAM | = | S-adenosylmethionine |
SG | = | sleeve gastrectomy |
SCDC | = | subacute combined degeneration of the spinal cord |
THF | = | tetrahydrofolate |
tHcy | = | total plasma homocysteine |
TC | = | transcobalamin |
T2D | = | type 2 diabetes |
UK | = | United Kingdom |
US | = | United States |
uMMA/C | = | urinary MMA/creatinine ratio |
B12 | = | vitamin B12 |
Acknowledgments
Renata Gorska of the Nutristasis Unit and Karol Piera of the Free University of Berlin/ETH Zürich are thanked for their artistic skills in drawing the figures.
Disclosure statement
No potential conflict of interest was reported by the author(s).
Correction Statement
This article has been corrected with minor changes. These changes do not impact the academic content of the article.
References
- Combe JS. History of a case of anaemia. Trans Med Chir Soc Edinb. 1824;1:194–204.
- Addison T. Anaemia-Disease of the supra-renal capsules. London Hospital Gazette. 1849;43:517–518.
- Biermer MA. Eine eigenthümliche Form von progressiver perniciöser Anämie. Correspondenz-Blatt Für Schweizer Aerzte. 1872;2:15–18.
- Osler WG. A case of progressive pernicious anemia (idiopathic of Addison). Can Med Surg J. 1877;5:385–404.
- Cohnheim J. Erkrankung des knochenmarkes bei perniziöser anämie. Archiv f Pathol Anat. 1876;68(2):291–293.
- Lichtheim L. Zur kenntniss der perniciösen anäemie. Munchener Medizinische Wochenschrift. 1887;34:300–306.
- Russell JSR, Batten FE, Collier J. Subacute combined degeneration of the spinal cord. Brain. 1900;23(1):39–110.
- Minot GR, Murphy WP. Treatment of pernicious anemia by a special diet. By George R. Minot and William P. Murphy. JAMA. 1983;250(24):3328–3335.
- Whipple Fsr-R GH. Blood regeneration in severe anemia. Am J Physiol. 1925;72:395–407.
- Hodgkin DC, Kamper J, Mackay M, et al. Structure of vitamin B12. Nature. 1956;178(4524):64–66.
- Gherasim C, Lofgren M, Banerjee R. Navigating the B(12) road: assimilation, delivery, and disorders of cobalamin. J Biol Chem. 2013;288(19):13186–13193.
- Kim J, Hannibal L, Gherasim C, et al. A human vitamin B12 trafficking protein uses glutathione transferase activity for processing alkylcobalamins. J Biol Chem. 2009;284(48):33418–33424.
- Girard CL, Santschi DE, Stabler SP, et al. Apparent ruminal synthesis and intestinal disappearance of vitamin B12 and its analogs in dairy cows. J Dairy Sci. 2009;92(9):4524–4529.
- Brouwer-Brolsma EM, Dhonukshe-Rutten RA, van Wijngaarden JP, et al. Dietary sources of vitamin B-12 and their association with vitamin B-12 status markers in healthy older adults in the B-PROOF study. Nutrients. 2015;7(9):7781–7797.
- Farquharson J, Adams JF. The forms of vitamin B12 in foods. Br J Nutr. 1976;36(1):127–136.
- Sharma S, Sheehy T, Kolonel LN. Contribution of meat to vitamin B12, iron and zinc intakes in five ethnic groups in the USA: implications for developing food-based dietary guidelines. J Hum Nutr Diet. 2013;26(2):156–168.
- Dror DK, Allen LH. Vitamin B-12 in human milk: a systematic review. Adv Nutr. 2018;9(suppl_1):358S–366S.
- De Angelis M, Bottacini F, Fosso B, et al. Lactobacillus rossiae, a vitamin B12 producer, represents a metabolically versatile species within the Genus Lactobacillus. PLoS One. 2014;9(9):e107232.
- LeBlanc JG, Milani C, de Giori GS, et al. Bacteria as vitamin suppliers to their host: a gut microbiota perspective. Curr Opin Biotechnol. 2013;24(2):160–168.
- Warren MJ, Raux E, Schubert HL, et al. The biosynthesis of adenosylcobalamin (vitamin B12). Nat Prod Rep. 2002;19(4):390–412.
- Fang H, Kang J, Zhang D. Microbial production of vitamin B12: a review and future perspectives. Microb Cell Fact. 2017;16(1):15.
- Bor M, Nexø E. Vitamin B12–cobalamin. In: Herrmann WOR, editor. Vitamins in the prevention of human diseases. Berlin: DeGruyter; 2011. p. 187–271.
- Doets EL, In 't Veld PH, Szczecińska A, et al. Systematic review on daily vitamin B12 losses and bioavailability for deriving recommendations on vitamin B12 intake with the factorial approach. Ann Nutr Metab. 2013;62(4):311–322.
- Ervin RW, Wang CY, Kennedy-Stephenson J. Dietary intake of selected vitamins for the United States population: 1999-2000. Hyattsville (Maryland): National Center for Health Statistics: Advance Data from Vital and Health Statistics; 2004.
- Derbyshire E. Micronutrient intakes of British adults across mid-life: a secondary analysis of the UK National Diet and Nutrition Survey. Front Nutr. 2018;5:55.
- Salmon J. Dietary reference values: a guide. London (UK): Department of Health, HMSO Publications Centre; 1991. p. 29.
- EFSA Panel on Dietetic Products NaA. Scientific opinion on Dietary Reference Values for cobalamin (vitamin B12). Parma (Italy): EFSA Panel on Dietetic Products NaA; 2015. p. 4150–4214.
- Medicine IO. Dietary reference intakes: thiamin, riboflavin, niacin, vitamin B6, folate, vitamin B12, pantothenic acid, biotin, and choline. Washington (DC): National Acedemies Press; 2000.
- Doets EL, Cavelaars AE, Dhonukshe-Rutten RA, et al. Explaining the variability in recommended intakes of folate, vitamin B12, iron and zinc for adults and elderly people. Public Health Nutr. 2012;15(5):906–915.
- Allen LH, Miller JW, de Groot L, et al. Biomarkers of nutrition for development (BOND): vitamin B-12 review. J Nutr. 2018;148(suppl_4):1995S–2027S.
- Fyfe JC, Madsen M, Hojrup P, et al. The functional cobalamin (vitamin B12)-intrinsic factor receptor is a novel complex of cubilin and amnionless. Blood. 2004;103(5):1573–1579.
- Schjønsby H. Vitamin B12 absorption and malabsorption. Gut. 1989;30(12):1686–1691.
- Chanarin I. The megaloblastic anemias. Oxford (UK): Blackwell Scientific; 1979.
- Adams JF, Ross SK, Mervyn L, et al. Absorption of cyanocobalamin, coenzyme B 12 , methylcobalamin, and hydroxocobalamin at different dose levels. Scand J Gastroenterol. 1971;6(3):249–252.
- Devi S, Pasanna RM, Shamshuddin Z, et al. Measuring vitamin B-12 bioavailability with [13C]-cyanocobalamin in humans. Am J Clin Nutr. 2020;112:1504–1515.
- Heyssel RM, Bozian RC, Darby WJ, et al. Vitamin B12 turnover in man. The assimilation of vitamin B12 from natural foodstuff by man and estimates of minimal daily dietary requirements. Am J Clin Nutr. 1966;18(3):176–184.
- Rutsch F, Gailus S, Miousse IR, et al. Identification of a putative lysosomal cobalamin exporter altered in the cblF defect of vitamin B12 metabolism. Nat Genet. 2009;41(2):234–239.
- Beedholm-Ebsen R, van de Wetering K, Hardlei T, et al. Identification of multidrug resistance protein 1 (MRP1/ABCC1) as a molecular gate for cellular export of cobalamin. Blood. 2010;115(8):1632–1639.
- Quadros EV, Rothenberg SP, Jaffe EA. Endothelial cells from human umbilical vein secrete functional transcobalamin II. Am J Physiol. 1989;256(2 Pt 1):C296–303.
- Hom BL. Plasma turnover of 57cobalt-vitamin B12 bound to transcobalamin I and II. Scand J Haematol. 1967;4(5):321–332.
- Hom BL, Olesen HA. Plasma clearance of 57cobalt-labelled vitamin B12 bound in vitro and in vivo to transcobalamin I and II. Scand J Clin Lab Invest. 1969;23(3):201–211.
- Collins DA, Hogenkamp HP, O'Connor MK, et al. Biodistribution of radiolabeled adenosylcobalamin in patients diagnosed with various malignancies. Mayo Clin Proc. 2000;75(6):568–580.
- Herbert V, editor. Vitamin B12 deficiency. Vitamin B12 deficiency – an overview. London: Royal Society of Medicine Press Ltd; 2002. p. 1–8.
- Nexo E, Gimsing P. Turnover in humans of iodine- and cobalamin-labeled transcobalamin I and of iodine-labeled albumin. Scand J Clin Lab Investig. 1975;35(5):391–398.
- Morkbak AL, Poulsen SS, Nexo E. Haptocorrin in humans. Clin Chem Lab Med. 2007;45(12):1751–1759.
- Golding PH. Experimental vitamin B12 deficiency in a human subject: a longitudinal investigation of the performance of the holotranscobalamin (HoloTC, Active-B12) immunoassay. Springerplus. 2016; 5:184.
- Kornerup LS, Hvas CL, Abild CB, et al. Early changes in vitamin B12 uptake and biomarker status following Roux-en-Y gastric bypass and sleeve gastrectomy. Clin Nutr. 2019;38(2):906–911.
- Quadros EV. Advances in the understanding of cobalamin assimilation and metabolism. Br J Haematol. 2010;148(2):195–204.
- Coelho D, Kim JC, Miousse IR, et al. Mutations in ABCD4 cause a new inborn error of vitamin B12 metabolism. Nat Genet. 2012;44(10):1152–1155.
- Kim J, Gherasim C, Banerjee R. Decyanation of vitamin B12 by a trafficking chaperone. Proc Natl Acad Sci U S A. 2008;105(38):14551–14554.
- Hannibal L, Kim J, Brasch NE, et al. Processing of alkylcobalamins in mammalian cells: a role for the MMACHC (cblC) gene product. Mol Genet Metab. 2009;97(4):260–266.
- Kolhouse JF, Utley C, Stabler SP, et al. Mechanism of conversion of human apo- to holomethionine synthase by various forms of cobalamin. J Biol Chem. 1991;266(34):23010–23015.
- Gherasim C, Hannibal L, Rajagopalan D, et al. The C-terminal domain of CblD interacts with CblC and influences intracellular cobalamin partitioning. Biochimie. 2013;95(5):1023–1032.
- Froese DS, Kopec J, Fitzpatrick F, et al. Structural Insights into the MMACHC-MMADHC Protein Complex Involved in Vitamin B12 Trafficking. J Biol Chem. 2015;290(49):29167–29177.
- Bassila C, Ghemrawi R, Flayac J, et al. Methionine synthase and methionine synthase reductase interact with MMACHC and with MMADHC. Biochim Biophys Acta Mol Basis Dis. 2017;1863(1):103–112.
- Huemer M, Diodato D, Schwahn B, et al. Guidelines for diagnosis and management of the cobalamin-related remethylation disorders cblC, cblD, cblE, cblF, cblG, cblJ and MTHFR deficiency. J Inherit Metab Dis. 2017;40(1):21–48.
- Rosenblatt DS, Cooper BA. Inherited disorders of vitamin B12 metabolism. Blood Rev. 1987;1(3):177–182.
- Gräsbeck R. Imerslund-Gräsbeck syndrome (selective vitamin B(12) malabsorption with proteinuria). Orphanet J Rare Dis. 2006;1:17.
- Andres E, Serraj K. Optimal management of pernicious anemia. J Blood Med. 2012;3:97–103.
- Hygum K, Lildballe DL, Greibe EH, et al. Mouse transcobalamin has features resembling both human transcobalamin and haptocorrin. PLoS One. 2011;6(5):e20638.
- Trakadis YJ, Alfares A, Bodamer OA, et al. Update on transcobalamin deficiency: clinical presentation, treatment and outcome. J Inherit Metab Dis. 2014;37(3):461–473.
- Froese DS, Gravel RA. Genetic disorders of vitamin B12 metabolism: eight complementation groups–eight genes. Expert Rev Mol Med. 2010;12:e37.
- Biancheri R, Cerone R, Rossi A, et al. Early-onset cobalamin C/D deficiency: epilepsy and electroencephalographic features. Epilepsia. 2002;43(6):616–622.
- Kirsch SH, Herrmann W, Obeid R. Genetic defects in folate and cobalamin pathways affecting the brain. Clin Chem Lab Med. 2013;51(1):139–155.
- Dib MJ, Elliott R, Ahmadi KR. A critical evaluation of results from genome-wide association studies of micronutrient status and their utility in the practice of precision nutrition. Br J Nutr. 2019;122(2):121–130.
- Surendran S, Adaikalakoteswari A, Saravanan P, et al. An update on vitamin B12-related gene polymorphisms and B12 status. Genes Nutr. 2018;13(1):132.
- Dalmia A, Dib MJ, Maude H, et al. A genetic epidemiological study in British adults and older adults shows a high heritability of the combined indicator of vitamin B12 status (cB12) and connects B12 status with utilization of mitochondrial substrates and energy metabolism. J Nutr Biochem. 2019;70:156–163.
- Grasbeck R. Hooked to vitamin B12 since 1955: a historical perspective. Biochimie. 2013;95(5):970–975.
- Degnan PH, Taga ME, Goodman AL. Vitamin B12 as a modulator of gut microbial ecology. Cell Metab. 2014;20(5):769–778.
- Renz P. Biosynthesis of the 5,6-dimethylbenzimidazole moiety of cobalamin and of the other bases found in natural corrinoids. In: Banerjee R, editor. Chemistry and biochemistry of B12. New York: John Wiley & Sons, Inc.; 1999. p. 557–575.
- Hardlei TF, Obeid R, Herrmann W, et al. Cobalamin analogues in humans: a study on maternal and cord blood. PLoS One. 2013;8(4):e61194.
- Brandt LJ, Bernstein LH, Wagle A. Production of vitamin B 12 analogues in patients with small-bowel bacterial overgrowth. Ann Intern Med. 1977;87(5):546–551.
- Nyberg W. The influence of Diphyllobothrium latum on the vitamin B12-intrinsic factor complex. 2. In vitro studies. Acta Med Scand. 1960;167:189–192.
- Giannella RA, Broitman SA, Zamcheck N. Competition between bacteria and intrinsic factor for vitamin B 12: implications for vitamin B 12 malabsorption in intestinal bacterial overgrowth. Gastroenterology. 1972;62(2):255–260.
- Shelton AN, Seth EC, Mok KC, et al. Uneven distribution of cobamide biosynthesis and dependence in bacteria predicted by comparative genomics. Isme J. 2019;13(3):789–804.
- Allen RH, Stabler SP. Identification and quantitation of cobalamin and cobalamin analogues in human feces. Am J Clin Nutr. 2008;87(5):1324–1335.
- Roth JR, Lawrence JG, Bobik TA. Cobalamin (coenzyme B12): synthesis and biological significance. Annu Rev Microbiol. 1996;50:137–181.
- Putnam EE, Goodman AL. B vitamin acquisition by gut commensal bacteria. PLoS Pathog. 2020;16(1):e1008208.
- Visconti A, Le Roy CI, Rosa F, et al. Interplay between the human gut microbiome and host metabolism. Nat Commun. 2019;10(1):4505.
- Pawlak R, Parrott SJ, Raj S, et al. How prevalent is vitamin B(12) deficiency among vegetarians? Nutr Rev. 2013;71(2):110–117.
- Carmel R. Subclinical cobalamin deficiency. Curr Opin Gastroenterol. 2012;28(2):151–158.
- Rowley CA, Kendall MM. To B12 or not to B12: five questions on the role of cobalamin in host-microbial interactions. PLoS Pathog. 2019;15(1):e1007479.
- Kolhouse JF, Kondo H, Allen NC, et al. Cobalamin analogues are present in human plasma and can mask cobalamin deficiency because current radioisotope dilution assays are not specific for true cobalamin. N Engl J Med. 1978;299(15):785–792.
- Coates ME, Davies MK, Harrison GF. Antivitamin B12 activity for the growing chick and developing chick embryo of some analogs of cyanocobalamin. Arch Biochem Biophys. 1960;87:93–99.
- Siddons RC, Spence JA, Dayan AD. Experimental vitamin B12 deficiency in the baboon. Adv Neurol. 1975; 10:239–252.
- Kanazawa S, Herbert V. Total corrinoid, cobalamin (vitamin B12), and cobalamin analogue levels may be normal in serum despite cobalamin in liver depletion in patients with alcoholism. Lab Invest. 1985;53(1):108–110.
- Carmel R. Biomarkers of cobalamin (vitamin B-12) status in the epidemiologic setting: a critical overview of context, applications, and performance characteristics of cobalamin, methylmalonic acid, and holotranscobalamin II. Am J Clin Nutr. 2011;94(1):348S–358S.
- Sun AL, Ni YH, Li XB, et al. Urinary methylmalonic acid as an indicator of early vitamin B12 deficiency and its role in polyneuropathy in type 2 diabetes. J Diabetes Res. 2014;2014:921616.
- Wasson K. Introduction: the blind men and the elephant: what “elephanomics” can teach “muromics”. Ilar J. 2006;47(2):91–93.
- Carmel R. Pernicious anemia. The expected findings of very low serum cobalamin levels, anemia, and macrocytosis are often lacking. Arch Intern Med. 1988;148(8):1712–1714.
- Lindenbaum J, Healton EB, Savage DG, et al. Neuropsychiatric disorders caused by cobalamin deficiency in the absence of anemia or macrocytosis. N Engl J Med. 1988;318(26):1720–1728.
- Hooper M, Hudson P, Porter F, et al. Patient journeys: diagnosis and treatment of pernicious anaemia. Br J Nurs. 2014;23(7):376–381.
- Harrington DJ. Investigation of megaloblastic anaemia: cobalamin, folate and metabolite status. Dacie and Lewis Practical Haematology. 12th ed. London: Elsevier; 2016.
- Oo TH. Diagnostic difficulties in pernicious anemia. Discov Med. 2019;28(155):247–253.
- Beck WS. Cobalamin and the nervous system. N Engl J Med. 1988;318(26):1752–1754.
- Healton EB, Savage DG, Brust JC, et al. Neurologic aspects of cobalamin deficiency. Medicine (Baltimore). 1991;70(4):229–245.
- Scott J, Weir D. Folate/vitamin B12 inter-relationships. Essays Biochem. 1994;28:63–72.
- Carmel R. Subtle and atypical cobalamin deficiency states. Am J Hematol. 1990;34(2):108–114.
- Carmel R. Current concepts in cobalamin deficiency. Annu Rev Med. 2000;51:357–375.
- Herbert V. The 1986 Herman award lecture. Nutrition science as a continually unfolding story: the folate and vitamin B-12 paradigm. Am J Clin Nutr. 1987;46(3):387–402.
- Chen MF, McIntyre PA, Wagner HN. Jr. A radiometric microbiologic method for vitamin B12 assay. J Nucl Med. 1977;18(4):388–393.
- Watanabe F, Katsura H, Takenaka S, et al. Pseudovitamin B(12) is the predominant cobamide of an algal health food, spirulina tablets. J Agric Food Chem. 1999;47(11):4736–4741.
- Ispir E, Serdar MA, Ozgurtas T, et al. Comparison of four automated serum vitamin B12 assays. Clin Chem Lab Med. 2015;53(8):1205–1213.
- Carmel R, Agrawal YP. Failures of cobalamin assays in pernicious anemia. N Engl J Med. 2012;367(4):385–386.
- Iltar U, Göçer M, Kurtoğlu E. False elevations of vitamin B12 levels due to assay errors in a patient with pernicious anemia. Blood Res. 2019;54(2):149–151.
- Oberley MJ, Yang DT. Laboratory testing for cobalamin deficiency in megaloblastic anemia. Am J Hematol. 2013;88(6):522–526.
- CD Creative Diagnostics. Vitamin B-12 ELISA Kit (DEIA-H002). Shirley, NY 11967, USA; 2019. Available from: https://www.creative-diagnostics.com/
- Aviva Systems Biology. Vitamin B12 ELISA Kit (OKEH02574). San Diego, CA 92111, USA; 2020. Available from: https://www.avivasysbio.com
- MyBioSource. VB12 ELISA kit: : Human Vitamin B12 ELISA Kit. San Diego, CA 92195-3308 USA; 2019. Available from: https://www.mybiosource.com/
- Hannibal L, Lysne V, Bjorke-Monsen AL. Biomarkers and algorithms for the diagnosis of vitamin B12 deficiency. Front Mol Biosci. 2016;3:27.
- Eussen SJ, de Groot LC, Clarke R, et al. Oral cyanocobalamin supplementation in older people with vitamin B12 deficiency: a dose-finding trial. Arch Intern Med. 2005;165(10):1167–1172.
- Lindenbaum J, Rosenberg IH, Wilson PW, et al. Prevalence of cobalamin deficiency in the Framingham elderly population. Am J Clin Nutr. 1994;60(1):2–11.
- Selhub J, Morris MS, Jacques PF. In vitamin B12 deficiency, higher serum folate is associated with increased total homocysteine and methylmalonic acid concentrations. Proc Natl Acad Sci USA. 2007;104(50):19995–20000.
- Bailey RL, Carmel R, Green R, et al. Monitoring of vitamin B-12 nutritional status in the United States by using plasma methylmalonic acid and serum vitamin B-12. Am J Clin Nutr. 2011;94(2):552–561.
- Bailey RL, Durazo-Arvizu RA, Carmel R, et al. Modeling a methylmalonic acid-derived change point for serum vitamin B-12 for adults in NHANES. Am J Clin Nutr. 2013;98(2):460–467.
- Sobczyńska-Malefora AC, Harrington DJ. The application of holotranscobalamin and methylmalonic acid in hospital patients and total vitamin B12 in primary care patients to assess low vitamin B12 status. J Hematol Thromb. 2015;1(2):8.
- Sobczyńska-Malefora A, Gorska R, Pelisser M, et al. An audit of holotranscobalamin (“Active” B12) and methylmalonic acid assays for the assessment of vitamin B12 status: application in a mixed patient population. Clin Biochem. 2014;47(1–2):82–86.
- Golding PH. Holotranscobalamin (HoloTC, Active-B12) and Herbert's model for the development of vitamin B12 deficiency: a review and alternative hypothesis. Springerplus. 2016;5(1):668.
- Jarquin Campos A, Risch L, Nydegger U, et al. Diagnostic accuracy of holotranscobalamin, vitamin B12, methylmalonic acid, and homocysteine in detecting B12 deficiency in a large, mixed patient population. Dis Markers. 2020;2020:7468506.
- Herrmann W, Obeid R. Utility and limitations of biochemical markers of vitamin B12 deficiency. Eur J Clin Invest. 2013;43(3):231–237.
- Hamilton MS, Lee A, Bonser J, et al. Ability of holotranscobalamin assay (Active B12) to detect severe cobalamin deficiency evidenced by high methylmalonic acid in the presence of high titre intrinsic factor antibody and false normal B12 results. 50th Annual Scientific Meeting of the British-Society-for-Haematology: British Journal of Haematology; 2010;149(Suppl 1):26.
- Morkbak AL, Hvas AM, Milman N, et al. Holotranscobalamin remains unchanged during pregnancy. Longitudinal changes of cobalamins and their binding proteins during pregnancy and postpartum. Haematologica. 2007;92(12):1711–1712.
- Keller P, Rufener J, Schild C, et al. False low holotranscobalamin levels in a patient with a novel TCN2 mutation. Clin Chem Lab Med. 2016;54(11):1739–1743.
- Sobczyńska-Malefora A, Pangilinan F, Plant GT, et al. Association of a transcobalamin II genetic variant with falsely low results for the holotranscobalamin immunoassay. Eur J Clin Invest. 2016;46(5):434–439.
- McCaddon A,C, Carr DF, Hudson P, et al. Transcobalamin receptor polymorphisms in the MRC Cognitive Function and Aging Study (MRC CFAS). 9th International Conference On Homocysteine and One Carbon Metabolism. J Inherit Metab Dis. 2013;36(Suppl 1):1–51.
- Flatley JE, Garner CM, Al-Turki M, et al. Determinants of urinary methylmalonic acid concentration in an elderly population in the United Kingdom. Am J Clin Nutr. 2012;95(3):686–693.
- Supakul S, Chabrun F, Genebrier S, et al. Diagnostic Performances of Urinary Methylmalonic Acid/Creatinine Ratio in Vitamin B12 Deficiency. J Clin Med. 2020;9(8):2335.
- Sobczyńska-Malefora A. Methods for assessment of folate (vitamin B9). In: Harrington DJ, editor. Laboratory assessment of vitamin status. London (UK): Academic Press; 2019. p. 219–264.
- Sobczyńska-Malefora A, Ramachandran R, Cregeen D, et al. An infant and mother with severe B12 deficiency: vitamin B12 status assessment should be determined in pregnant women with anaemia. Eur J Clin Nutr. 2017;71(8):1013–1015.
- Fedosov SN. Biochemical markers of vitamin B12 deficiency combined in one diagnostic parameter: the age-dependence and association with cognitive function and blood hemoglobin. Clin Chim Acta. 2013; 422(42247):47–53.
- Fedosov SN, Brito A, Miller JW, et al. Combined indicator of vitamin B12 status: modification for missing biomarkers and folate status and recommendations for revised cut-points. Clin Chem Lab Med. 2015;53(8):1215–1225.
- Sobczyńska-Malefora AG, Ahmadi M, Harrington KR, et al. The pregnancy related reference ranges for markers of vitamin B12 status. Nova Scotia: FASEB; 2018.
- Sebastiani G, Herranz Barbero A, Borrás-Novell C, et al. The effects of vegetarian and vegan diet during pregnancy on the health of mothers and offspring. Nutrients. 2019;11(3):557.
- Stabler SP, Allen RH. Vitamin B12 deficiency as a worldwide problem. Annu Rev Nutr. 2004;24(24299):299–326.
- Antony AC. Vegetarianism and vitamin B-12 (cobalamin) deficiency. Am J Clin Nutr. 2003;78(1):3–6.
- Watanabe F, Yabuta Y, Bito T, et al. Vitamin B12-containing plant food sources for vegetarians. Nutrients. 2014;6(5):1861–1873.
- Watanabe F. Vitamin B12 sources and bioavailability. Exp Biol Med (Maywood). 2007;232(10):1266–1274.
- Yajnik CS, Deshpande SS, Lubree HG, et al. Vitamin B12 deficiency and hyperhomocysteinemia in rural and urban Indians. J Assoc Physicians India. 2006;54:775–782.
- Herrmann W, Schorr H, Obeid R, et al. Vitamin B-12 status, particularly holotranscobalamin II and methylmalonic acid concentrations, and hyperhomocysteinemia in vegetarians. Am J Clin Nutr. 2003;78(1):131–136.
- Bito T, Bito M, Asai Y, et al. Characterization and quantitation of vitamin B12 compounds in various Chlorella supplements. J Agric Food Chem. 2016;64(45):8516–8524.
- Helliwell KE, Lawrence AD, Holzer A, et al. Cyanobacteria and eukaryotic algae use different chemical variants of Vitamin B12. Curr Biol. 2016;26(8):999–1008.
- Visentin CE, Masih SP, Plumptre L, et al. Low serum Vitamin B-12 concentrations are prevalent in a cohort of pregnant Canadian women. J Nutr. 2016;146(5):1035–1042.
- Murphy MM, Molloy AM, Ueland PM, et al. Longitudinal study of the effect of pregnancy on maternal and fetal cobalamin status in healthy women and their offspring. J Nutr. 2007;137(8):1863–1867.
- Finkelstein JL, Guillet R, Pressman EK, et al. Vitamin B(12) status in pregnant adolescents and their infants. Nutrients. 2019;11(2):397.
- Plumptre L, Tammen SA, Sohn KJ, et al. Maternal and cord blood folate concentrations are inversely associated with fetal DNA hydroxymethylation, but not DNA methylation, in a cohort of pregnant Canadian women. J Nutr. 2020;150(2):202–211.
- Layden AJ, O'Brien KO, Pressman EK, et al. Vitamin B12 and placental expression of transcobalamin in pregnant adolescents. Placenta. 2016;45:1–7.
- Frery N, Huel G, Leroy M, et al. Vitamin B12 among parturients and their newborns and its relationship with birthweight. Eur J Obstet Gynecol Reprod Biol. 1992;45(3):155–163.
- Ronnenberg AG, Goldman MB, Chen D, et al. Preconception homocysteine and B vitamin status and birth outcomes in Chinese women. Am J Clin Nutr. 2002;76(6):1385–1391.
- Duggan C, Srinivasan K, Thomas T, et al. Vitamin B-12 supplementation during pregnancy and early lactation increases maternal, breast milk, and infant measures of vitamin B-12 status. J Nutr. 2014;144(5):758–764.
- Siddiqua TJ, Ahmad SM, Ahsan KB, et al. Vitamin B12 supplementation during pregnancy and postpartum improves B12 status of both mothers and infants but vaccine response in mothers only: a randomized clinical trial in Bangladesh. Eur J Nutr. 2016;55(1):281–293.
- Candito M, Magnaldo S, Bayle J, et al. Clinical B12 deficiency in one case of recurrent spontaneous pregnancy loss. Clin Chem Lab Med. 2003;41(8):1026–1027.
- Hibbard ED, Spencer WJ. Low serum B12 levels and latent Addisonian anaemia in pregnancy. J Obstet Gynaecol Br Commonw. 1970;77(1):52–57.
- Ball EW, Giles C. Folic acid and vitamin B12 levels in pregnancy and their relation to megaloblastic anaemia. J Clin Pathol. 1964;17(2):165–174.
- Metz J, McGrath K, Bennett M, et al. Biochemical indices of vitamin B12 nutrition in pregnant patients with subnormal serum vitamin B12 levels. Am J Hematol. 1995;48(4):251–255.
- Pardo J, Peled Y, Bar J, et al. Evaluation of low serum vitamin B(12) in the non-anaemic pregnant patient. Hum Reprod. 2000;15(1):224–226.
- Walker MC, Smith GN, Perkins SL, et al. Changes in homocysteine levels during normal pregnancy. Am J Obstet Gynecol. 1999;180(3):660–664.
- Koebnick C, Heins UA, Dagnelie PC, et al. Longitudinal concentrations of vitamin B(12) and vitamin B(12)-binding proteins during uncomplicated pregnancy. Clin Chem. 2002;48(6):928–933.
- Milman N, Bergholt T, Byg KE, et al. Reference intervals for haematological variables during normal pregnancy and postpartum in 434 healthy Danish women. Eur J Haematol. 2007;79(1):39–46.
- Schroder TH, Tan A, Mattman A, et al. Reference intervals for serum total vitamin B12 and holotranscobalamin concentrations and their change points with methylmalonic acid concentration to assess vitamin B12 status during early and mid-pregnancy. Clin Chem Lab Med. 2019;57(11):1790–1798.
- Johnson LR. Functional development of the stomach. Annu Rev Physiol. 1985;47:199–215.
- Adkins Y, Lonnerdal B. Mechanisms of vitamin B(12) absorption in breast-fed infants. J Pediatr Gastroenterol Nutr. 2002;35(2):192–198.
- Obeid R, Murphy M, Sole-Navais P, et al. Cobalamin status from pregnancy to early childhood: lessons from global experience. Adv Nutr. 2017;8(6):971–979.
- Honzik T, Adamovicova M, Smolka V, et al. Clinical presentation and metabolic consequences in 40 breastfed infants with nutritional vitamin B12 deficiency-what have we learned? Eur J Paediatr Neurol. 2010;14(6):488–495.
- Hay G, Johnston C, Whitelaw A, et al. Folate and cobalamin status in relation to breastfeeding and weaning in healthy infants. Am J Clin Nutr. 2008;88(1):105–114.
- Chandyo RK, Ulak M, Kvestad I, et al. Cobalamin and folate status among breastfed infants in Bhaktapur, Nepal. Nutrients. 2018;10(5):639.
- Bjorke-Monsen AL, Torsvik I, Saetran H, et al. Common metabolic profile in infants indicating impaired cobalamin status responds to cobalamin supplementation. Pediatrics. 2008;122(1):83–91.
- Torsvik IK, Ueland PM, Markestad T, et al. Motor development related to duration of exclusive breastfeeding, B vitamin status and B12 supplementation in infants with a birth weight between 2000-3000 g, results from a randomized intervention trial. BMC Pediatr. 2015;15(1):15218.
- Torsvik I, Ueland PM, Markestad T, et al. Cobalamin supplementation improves motor development and regurgitations in infants: results from a randomized intervention study. Am J Clin Nutr. 2013;98(5):1233–1240.
- Hvas AM, Morkbak AL, Nexo E. Plasma holotranscobalamin compared with plasma cobalamins for assessment of vitamin B12 absorption; optimisation of a non-radioactive vitamin B12 absorption test (CobaSorb). Clin Chim Acta. 2007;376(1–2):150–154.
- Andres E, Affenberger S, Vinzio S, et al. Food-cobalamin malabsorption in elderly patients: clinical manifestations and treatment. Am J Med. 2005;118(10):1154–1159.
- Selhub J, Bagley LC, Miller J, et al. B vitamins, homocysteine, and neurocognitive function in the elderly. Am J Clin Nutr. 2000;71(2):614S–620S.
- Krasinski SD, Russell RM, Samloff IM, et al. Fundic atrophic gastritis in an elderly population. Effect on hemoglobin and several serum nutritional indicators. J Am Geriatr Soc. 1986;34(11):800–806.
- Nilsson-Ehle H, Jagenburg R, Landahl S, et al. Serum cobalamins in the elderly: a longitudinal study of a representative population sample from age 70 to 81. Eur J Haematol. 1991;47(1):10–16.
- Pfeiffer CM, Johnson CL, Jain RB, et al. Trends in blood folate and vitamin B-12 concentrations in the United States, 1988-2004. Am J Clin Nutr. 2007;86(3):718–727.
- Clarke R, Grimley Evans J, Schneede J, et al. Vitamin B12 and folate deficiency in later life. Age Ageing. 2004;33(1):34–41.
- Miller JW, Garrod MG, Allen LH, et al. Metabolic evidence of vitamin B-12 deficiency, including high homocysteine and methylmalonic acid and low holotranscobalamin, is more pronounced in older adults with elevated plasma folate. Am J Clin Nutr. 2009;90(6):1586–1592.
- Khodabandehloo N, Vakili M, Hashemian Z, et al. Determining functional Vitamin B12 deficiency in the elderly. Iran Red Crescent Med J. 2015;17(8):e13138.
- Zhang Y, Hodgson NW, Trivedi MS, et al. Decreased brain levels of Vitamin B12 in aging, autism and schizophrenia. PLoS One. 2016;11(1):e0146797.
- Vogiatzoglou A, Smith AD, Nurk E, et al. Cognitive function in an elderly population: interaction between vitamin B12 status, depression, and apolipoprotein E ε4: the Hordaland Homocysteine Study. Psychosom Med. 2013;75(1):20–29.
- Osborne D, Sobczynska-Malefora A. Autoimmune mechanisms in pernicious anaemia & thyroid disease. Autoimmun Rev. 2015;14(9):763–768.
- Toh BH, van Driel IR, Gleeson PA. Pernicious anemia. N Engl J Med. 1997;337(20):1441–1448.
- Remacha AF, Zapico E, Sarda MP, et al. Immune complexes and persistent high levels of serum vitamin B12. Int J Lab Hematol. 2014;36(1):92–97.
- Carmel R, Tatsis B, Baril L. Circulating antibody to transcobalamin II causing retention of vitamin B12 in the blood. Blood. 1977;49(6):987–1000.
- Blaser MJ, Parsonnet J. Parasitism by the ”slow“ bacterium Helicobacter pylori leads to altered gastric homeostasis and neoplasia. J Clin Invest. 1994;94(1):4–8.
- Kaptan K, Beyan C, Ural AU, et al. Helicobacter pylori-is it a novel causative agent in Vitamin B12 deficiency? Arch Intern Med. 2000;160(9):1349–1353.
- Bergman MP, Vandenbroucke-Grauls CM, Appelmelk BJ, et al. The story so far: Helicobacter pylori and gastric autoimmunity. Int Rev Immunol. 2005;24(1–2):63–91.
- Sharma K, Wijarnpreecha K, Merrell N. Diphyllobothrium latum mimicking subacute appendicitis. Gastroenterol Res. 2018;11(3):235–237.
- Ward MG, Kariyawasam VC, Mogan SB, et al. Prevalence and risk factors for functional Vitamin B12 deficiency in patients with Crohn's disease. Inflamm Bowel Dis. 2015;21(12):2839–2847.
- Allen RH, Seetharam B, Podell E, et al. Effect of proteolytic enzymes on the binding of cobalamin to R protein and intrinsic factor. In vitro evidence that a failure to partially degrade R protein is responsible for cobalamin malabsorption in pancreatic insufficiency. J Clin Invest. 1978;61(1):47–54.
- Ehrenpreis ED, Carlson SJ, Boorstein HL, et al. Malabsorption and deficiency of vitamin B12 in HIV-infected patients with chronic diarrhea. Digest Dis Sci. 1994;39(10):2159–2162.
- Smith CD, Herkes SB, Behrns KE, et al. Gastric acid secretion and vitamin B12 absorption after vertical Roux-en-Y gastric bypass for morbid obesity. Ann Surg. 1993;218(1):91–96.
- Green R, Allen LH, Bjorke-Monsen AL, et al. Vitamin B12 deficiency. Nat Rev Dis Primers. 2017;3(1):317040.
- Luciano-Mateo F, Hernández-Aguilera A, Cabre N, et al. Nutrients in energy and one-carbon metabolism: learning from metformin users. Nutrients. 2017;9(2):121.
- Miller JW. Proton pump inhibitors, H2-receptor antagonists, metformin, and Vitamin B-12 deficiency: clinical implications. Adv Nutr. 2018;9(4):511S–518S.
- Zhang Q, Li S, Li L, et al. Metformin treatment and homocysteine: a systematic review and meta-analysis of randomized controlled trials. Nutrients. 2016;8(12):798.
- Pflipsen MC, Oh RC, Saguil A, et al. The prevalence of vitamin B(12) deficiency in patients with type 2 diabetes: a cross-sectional study. J Am Board Fam Med. 2009;22(5):528–534.
- Wile DJ, Toth C. Association of metformin, elevated homocysteine, and methylmalonic acid levels and clinically worsened diabetic peripheral neuropathy. Diabetes Care. 2010;33(1):156–161.
- Leung S, Mattman A, Snyder F, et al. Metformin induces reductions in plasma cobalamin and haptocorrin bound cobalamin levels in elderly diabetic patients. Clin Biochem. 2010;43(9):759–760.
- Obeid R, Jung J, Falk J, et al. Serum vitamin B12 not reflecting vitamin B12 status in patients with type 2 diabetes. Biochimie. 2013;95(5):1056–1061.
- Bauman WA, Shaw S, Jayatilleke E, et al. Increased intake of calcium reverses vitamin B12 malabsorption induced by metformin. Diabetes Care. 2000;23(9):1227–1231.
- Liu X, Romero IL, Litchfield LM, et al. Metformin targets central carbon metabolism and reveals mitochondrial requirements in human cancers. Cell Metab. 2016;24(5):728–739.
- Valuck RJ, Ruscin JM. A case-control study on adverse effects: H2 blocker or proton pump inhibitor use and risk of vitamin B12 deficiency in older adults. J Clin Epidemiol. 2004;57(4):422–428.
- Dharmarajan TS, Kanagala MR, Murakonda P, et al. Do acid-lowering agents affect vitamin B12 status in older adults? J Am Med Dir Assoc. 2008;9(3):162–167.
- den Elzen WP, Groeneveld Y, de Ruijter W, et al. Long-term use of proton pump inhibitors and vitamin B12 status in elderly individuals. Aliment Pharmacol Ther. 2008;27(6):491–497.
- Labenz J, Tillenburg B, Peitz U, et al. Helicobacter pylori augments the pH-increasing effect of omeprazole in patients with duodenal ulcer. Gastroenterology. 1996;110(3):725–732.
- Sagar M, Janczewska I, Ljungdahl A, et al. Effect of CYP2C19 polymorphism on serum levels of vitamin B12 in patients on long-term omeprazole treatment. Aliment Pharmacol Ther. 1999;13(4):453–458.
- Furuta T, Shirai N, Sugimoto M, et al. Influence of CYP2C19 pharmacogenetic polymorphism on proton pump inhibitor-based therapies. Drug Metab Pharmacokinet. 2005;20(3):153–167.
- Saltzman JR, Kemp JA, Golner BB, et al. Effect of hypochlorhydria due to omeprazole treatment or atrophic gastritis on protein-bound vitamin B12 absorption. J Am Coll Nutr. 1994;13(6):584–591.
- Wilson SM, Bivins BN, Russell KA, et al. Oral contraceptive use: impact on folate, vitamin B6, and vitamin B12 status. Nutr Rev. 2011;69(10):572–583.
- Berenson AB, Rahman M. Effect of hormonal contraceptives on vitamin B12 level and the association of the latter with bone mineral density. Contraception. 2012;86(5):481–487.
- Riedel B, Bjørke Monsen AL, Ueland PM, et al. Effects of oral contraceptives and hormone replacement therapy on markers of cobalamin status. Clin Chem. 2005;51(4):778–781.
- Riedel B, Bjørke Monsen AL, Ueland PM, Schneede J. Effects of oral contraceptives and hormone replacement therapy on markers of cobalamin status. Clin Chem. 2005;51(4):778–781.
- Bao L, Li Q, Li Q, et al. Clinical, electrophysiological and radiological features of nitrous oxide-induced neurological disorders. Neuropsychiatr Dis Treat. 2020;16:977–984.
- Li Z, Shanmuganathan A, Ruetz M, et al. Coordination chemistry controls the thiol oxidase activity of the B12-trafficking protein CblC. J Biol Chem. 2017;292(23):9733–9744.
- Yamada K, Gravel RA, Toraya T, et al. Human methionine synthase reductase is a molecular chaperone for human methionine synthase. Proc Natl Acad Sci USA. 2006;103(25):9476–9481.
- Hathout L, El-Saden S. Nitrous oxide-induced B12 deficiency myelopathy: perspectives on the clinical biochemistry of vitamin B12. J Neurol Sci. 2011;301(1–2):1–8.
- Pratt J. Inorganic chemistry of vitamin B12. London (UK): Academic Press; 1972.
- Choi C, Kim T, Park KD, et al. Subacute combined degeneration caused by nitrous oxide intoxication: a report of two cases. Ann Rehabil Med. 2019;43(4):530–534.
- Thompson AG, Leite MI, Lunn MP, et al. Whippits, nitrous oxide and the dangers of legal highs. Pract Neurol. 2015;15(3):207–209.
- Zanardo V, Caroni G, Burlina A. Higher homocysteine concentrations in women undergoing caesarean section under general anesthesia. Thromb Res. 2003;112(1–2):33–36.
- Myles PS, Chan MT, Leslie K, et al. Effect of nitrous oxide on plasma homocysteine and folate in patients undergoing major surgery. Br J Anaesth. 2008;100(6):780–786.
- Wrońska-Nofer T, Nofer JR, Jajte J, et al. Oxidative DNA damage and oxidative stress in subjects occupationally exposed to nitrous oxide (N(2)O). Mutat Res. 2012;731(1–2):58–63.
- NICE. NIfHaCE. Anaemia – B12 and folate deficiency. NICE CKS; 2018.
- Mechanick JI, Apovian C, Brethauer S, et al. Clinical practice guidelines for the perioperative nutrition, metabolic, and nonsurgical support of patients undergoing bariatric procedures – 2019 Update: cosponsored by American Association of Clinical Endocrinologists/American College of Endocrinology, The Obesity Society, American Society for Metabolic & Bariatric SURGERY, OBESITY MEDICINE ASSOCIATION, AND AMERICAN SOCIETY OF ANESTHESIOLOGISTS – EXECUTIVE SUMMARY. Endocr Pract. 2019;25(12):1346–1359.
- Smelt HJ, Pouwels S, Smulders JF. Different supplementation regimes to treat perioperative Vitamin B12 deficiencies in bariatric surgery: a systematic review. Obes Surg. 2017;27(1):254–262.
- Masucci L, Goeree R. Vitamin B12 intramuscular injections versus oral supplements: a budget impact analysis. Ont Health Technol Assess Ser. 2013;13(24):1–24.
- Thakkar K, Billa G. Treatment of vitamin B12 deficiency-methylcobalamine? Cyancobalamine? Hydroxocobalamin?-clearing the confusion. Eur J Clin Nutr. 2015;69(1):1–2.
- Obeid R, Fedosov SN, Nexo E. Cobalamin coenzyme forms are not likely to be superior to cyano- and hydroxyl-cobalamin in prevention or treatment of cobalamin deficiency. Mol Nutr Food Res. 2015;59(7):1364–1372.
- Arendt J, Nexø E. [Treatment response in vitamin B12 deficiency depends on the chosen vitamin B12 preparation]. Ugeskr Laeger. 2011;173(42):2634–2635.
- Glass GB, Lee DH, Hardy WW. Hydroxocobalamin. II. Absorption from the site of injection and uptake by the liver and calf muscle in man. Blood. 1961;18:522.
- Hertz H, Kristensen HP, Hoff-Jorgensen E. Studies on vitamin B12 retention. Comparison of retention following intramuscular injection of cyanocobalamin and hydroxocobalamin. Scand J Haematol. 1964;1:5-15.
- Hertz H, Kristensen HP, Hoff-Jorgensen E. [Retention of vitamin b12. A comparative study of retention after intramuscular injection of cyanocobalamin and hydrooxocobalamin respectively]. Ugeskr Laeger. 1964;126:535–541.
- Jones R. Swallow your pride. Can Fam Physician. 2008;54(7):978–979.
- Sanz-Cuesta T, Escortell-Mayor E, Cura-Gonzalez I, et al. Oral versus intramuscular administration of vitamin B12 for vitamin B12 deficiency in primary care: a pragmatic, randomised, non-inferiority clinical trial (OB12). BMJ Open. 2020;10(8):e033687.
- Kuzminski AM, Del Giacco EJ, Allen RH, et al. Effective treatment of cobalamin deficiency with oral cobalamin. Blood. 1998;92(4):1191–1198.
- Bolaman Z, Kadikoylu G, Yukselen V, et al. Oral versus intramuscular cobalamin treatment in megaloblastic anemia: a single-center, prospective, randomized, open-label study. Clin Ther. 2003;25(12):3124–3134.
- Slot WB, Merkus FW, Van Deventer SJ, et al. Normalization of plasma vitamin B12 concentration by intranasal hydroxocobalamin in vitamin B12-deficient patients. Gastroenterology. 1997;113(2):430–433.
- Sharabi A, Cohen E, Sulkes J, et al. Replacement therapy for vitamin B12 deficiency: comparison between the sublingual and oral route. Br J Clin Pharmacol. 2003;56(6):635–638.
- Del Bo C, Riso P, Gardana C, et al. Effect of two different sublingual dosages of vitamin B(12) on cobalamin nutritional status in vegans and vegetarians with a marginal deficiency: a randomized controlled trial. Clin Nutr. 2019;38(2):575–583.
- Dary O. Establishing safe and potentially efficacious fortification contents for folic acid and vitamin B12. Food Nutr Bull. 2008;29(2 Suppl):S214–S224.
- Kumaran K, Yajnik P, Lubree H, et al. The Pune Rural Intervention in Young Adolescents (PRIYA) study: design and methods of a randomised controlled trial. BMC Nutr. 2017;3:41.
- Naik S, Mahalle N, Greibe E, et al. Cyano-B12 or whey powder with endogenous hydroxo-B12 for supplementation in B12 deficient lactovegetarians. Nutrients. 2019;11(10):2382.
- Smith AD, Refsum H. Homocysteine, B Vitamins, and cognitive impairment. Annu Rev Nutr. 2016;36:211–239.
- Patel KR, Sobczynska-Malefora A. The adverse effects of an excessive folic acid intake. Eur J Clin Nutr. 2017;71(2):159–163.
- Andrès E, Serraj K, Zhu J, et al. The pathophysiology of elevated vitamin B12 in clinical practice. QJM. 2013;106(6):505–515.
- Arendt JF, Nexo E. Unexpected high plasma cobalamin: proposal for a diagnostic strategy. Clin Chem Lab Med. 2013;51(3):489–496.
- Murphy G, Dawsey SM, Engels EA, et al. Cancer risk after pernicious anemia in the US elderly population. Clin Gastroenterol Hepatol. 2015;13(13):2282–2289.
- Kokkola A, Sjöblom SM, Haapiainen R, et al. The risk of gastric carcinoma and carcinoid tumours in patients with pernicious anaemia. A prospective follow-up study. Scand J Gastroenterol. 1998;33(1):88–92.
- Mahmud N, Stashek K, Katona BW, et al. The incidence of neoplasia in patients with autoimmune metaplastic atrophic gastritis: a renewed call for surveillance. Ann Gastroenterol. 2019;32(1):67–72.
- Arendt JF, Pedersen L, Nexo E, et al. Elevated plasma vitamin B12 levels as a marker for cancer: a population-based cohort study. J Natl Cancer Inst. 2013;105(23):1799–1805.
- Fanidi A, Carreras-Torres R, Larose TL, et al. Is high vitamin B12 status a cause of lung cancer? Int J Cancer. 2019;145(6):1499–1503.
- Moore E, Mander A, Ames D, et al. Cognitive impairment and vitamin B12: a review. Int Psychogeriatr. 2012;24(4):541–556.
- van Tiggelen CJM. Dementia: association with zinc deficiency and cerebral vitamin B12 Deficiency. J Orthomolec Psychatr. 1984;13(2):97–104.
- Cole MG, Prchal JF. Low serum vitamin B12 in Alzheimer-type dementia. Age Ageing. 1984;13(2):101–105.
- McCaddon A, Kelly CL. Familial Alzheimer's disease and vitamin B12 deficiency. Age Ageing. 1994;23(4):334–337.
- McCaddon A. Vitamin B12 in neurology and ageing; clinical and genetic aspects. Biochimie. 2013;95(5):1066–1076.
- Smith AD, Smith SM, de Jager CA, et al. Homocysteine-lowering by B vitamins slows the rate of accelerated brain atrophy in mild cognitive impairment: a randomized controlled trial. PLoS One. 2010;5(9):e12244.
- de Jager CA, Oulhaj A, Jacoby R, et al. Cognitive and clinical outcomes of homocysteine-lowering B-vitamin treatment in mild cognitive impairment: a randomized controlled trial. Int J Geriatr Psychiatry. 2012;27(6):592–600.
- Smith AD, Refsum H, Bottiglieri T, et al. Homocysteine and dementia: an International Consensus Statement. J Alzheimers Dis. 2018;62(2):561–570.
- Reynolds EH, Linnell JC, Faludy JE. Multiple sclerosis associated with vitamin B12 deficiency. Arch Neurol. 1991;48(8):808–811.
- Goodkin DE, Jacobsen DW, Galvez N, et al. Serum cobalamin deficiency is uncommon in multiple sclerosis. Arch Neurol. 1994;51(11):1110–1114.
- Reynolds EH, Bottiglieri T, Laundy M, et al. Vitamin B12 metabolism in multiple sclerosis. Arch Neurol. 1992;49(6):649–652.
- Nijst TQ, Wevers RA, Schoonderwaldt HC, et al. Vitamin B12 and folate concentrations in serum and cerebrospinal fluid of neurological patients with special reference to multiple sclerosis and dementia. J Neurol Neurosurg Psychiatry. 1990;53(11):951–954.
- Reynolds EH. Multiple sclerosis and vitamin B12 metabolism. J Neurol Neurosurg Psychiatry. 1992;55(5):339–340.
- Kira J, Tobimatsu S, Goto I. Vitamin B12 metabolism and massive-dose methyl vitamin B12 therapy in Japanese patients with multiple sclerosis. Intern Med. 1994;33(2):82–86.
- Boucher JL. The cause of multiple sclerosis is autoimmune attack of adenosyltransferase thereby limiting adenosylcobalamin production. Med Hypotheses. 2017;109:29–37.