Abstract
In this review, we summarize the relationship of PCT with pathogens, evaluate the clinical utility of PCT in the diagnosis of clinical diseases, condition monitoring and evaluation, and guiding medical decision-making, and explore current knowledge on the mechanisms by which pathogens cause changes in PCT levels. The lipopolysaccharides of the microorganisms stimulate cytokine production in host cells, which in turn stimulates production of serum PCT. Pathogens have different virulence mechanisms that lead to variable host inflammatory responses, and differences in the specific signal transduction pathways result in variable serum PCT concentrations. The mechanisms of signal transduction have not been fully elucidated. Further studies are necessary to ascertain the PCT fluctuation range of each pathogen. PCT levels are helpful in distinguishing between certain pathogens, in deciding if antibiotics are indicated, and in monitoring response to antibiotics.
Overview of procalcitonin
Procalcitonin (PCT) is composed of 116 amino acids and has a molecular weight of 14 kDa [Citation1]. It is coded for by the calcitonin I (CALC-I) gene on chromosome 11 and it is the precursor of calcitonin [Citation1], which Le Moullec et al. first described as a biologically active substance in 1984 [Citation2]. PCT is produced primarily in the C cells of the thyroid gland. First, pre-procalcitonin is synthesized by the thyroid C cells and endopeptidases cut the 25-amino acid signal sequence to convert it to PCT [Citation3]. Then, PCT is converted to calcitonin by prohormone converting enzyme. Calcitonin is a 32-amino acid hormone that regulates serum calcium [Citation3]. Under normal physiologic conditions, the expression of CALC-I outside the thyroid is inhibited in the absence of infection [Citation4]. Therefore, the serum PCT concentration in the normal population is low (<0.05 ng/mL) [Citation3]. Endotoxin, a lipopolysaccharide (LPS) released from bacteria and cytokines that are stimulated by bacterial infection can induce CALC-I expression in extra-thyroidal organs such as the liver, pancreas, kidney, lung, intestine and leukocytes, leading to a sharp increase of PCT levels [Citation1]. PCT levels can rise by 100–1000 fold and may rise to greater than 100 ng/mL), especially in patients with severe bacterial infections or sepsis [Citation5–7]. In the systemic circulation, the half-life of PCT is 25–30 h, which is quite long [Citation8]. The serum PCT level decreases with control of the infection. Therefore, PCT is a biomarker that can indicate the severity of bacterial infections and it plays an important role in the rapid diagnosis of infectious diseases, monitoring, and guiding clinical decision-making. In contrast, cytokines released after a viral infection down-regulate PCT production, which leads to little or no increase in serum PCT levels [Citation3]. PCT levels are minimally affected by virus-induced systemic inflammatory response syndrome (SIRS) [Citation4]. For example, a slight increase (<0.5 ng/mL) in PCT levels is commonly found in acute respiratory syndrome coronavirus 2-(SARS-CoV-2-) positive patients as compared with negative or untested patients [Citation5], making PCT a valuable biomarker to distinguish this viral infection from bacterial infections [Citation4]. Furthermore, noninfectious inflammatory reactions, autoimmune diseases and allergic disorders do not or only mildly increase serum PCT levels, which means that PCT may help to distinguish these diseases from bacterial infections [Citation9]. A case report has shown that patients with anaphylactic shock may have a significantly higher PCT level that could be misdiagnosed as septic shock [Citation10].
PCT levels are influenced by many factors, and high serum PCT level are associated with severe renal impairment and congestive heart failure, systemic inflammatory reactions such as inhalation injury, pancreatitis, burn injuries, severe trauma, surgery and circulatory shock, and cancers such as small cell lung cancer, bronchial cancer, and medullary cell carcinoma of the thyroid gland [Citation5,Citation11]. On the other hand, localized infections such as mediastinitis, empyema, abscesses, and atypical pneumonia can lead to false reductions in serum PCT [Citation5,Citation11]. Immunosuppressive therapy does not affect PCT levels [Citation4]. In the past decade, numerous studies have aimed to explain the clinical utility of PCT (). However, there are few reviews that discuss the connection between changes in PCT levels and specific pathogenic microorganisms. In this review, we will describe the associations between PCT and specific pathogenic organisms, discuss the pathogenetic mechanisms of the variation in PCT levels by different microorganisms, and show that monitoring PCT levels may help physicians to determine the type of specific pathogenic microorganisms.
Figure 1. The word cloud model of procalcitonin. We conducted literature research in PubMed with the keywords “procalcitonin” (title search), and drew the word cloud with WordArt (http://wordart.com) based on 1000 articles containing procalcitonin in titles from 2017 to 2021. The word cloud displays the size of a word proportionally to the number of times the word appears in a section of text.
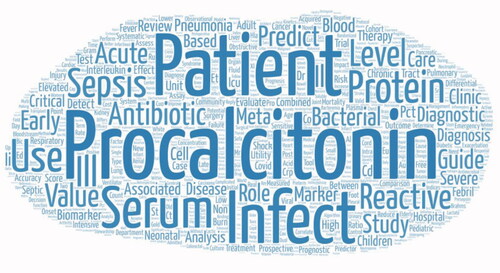
The clinical utility of procalcitonin
In 1993, Assicot et al. evaluated the role of PCT in distinguishing bacterial and non-bacterial infections in children [Citation12]. Although microbial culture remains the gold standard for identification of bacterial infections, it has many limitations. First, it can be cumbersome to obtain high-yield samples from sites such as the lungs and abdominal cavity. Second, the results from microbial cultures may take a long time, delaying the diagnosis and susceptibility test results regarding the antimicrobial agents to use. Third, blood cultures are not very sensitive. False-negative results of microbial blood cultures are common, leading to missed diagnosis and sometimes to the use of broad-spectrum antibiotics for a longer time than should be necessary. In addition, other factors such as antibiotic treatment affecting the detection rate of bacteria, and difficulties in multi-bacterial culture and in distinguishing normal flora and pathogenic bacteria make microbial culture unsuitable for rapid diagnosis in many cases [Citation4,Citation13]. In contrast, PCT is a simple serum biomarker that can distinguish bacterial infections from non-bacterial infections and in some situations can indicate the type of pathogenic microorganisms with good specificity and sensitivity [Citation13–15]. Its value is manifested mainly in the rapid diagnosis of clinical diseases, condition monitoring and evaluation, and guiding medical decision-making [Citation4].
Rapid diagnosis of clinical diseases
Under normal circumstances, the serum PCT concentration is low, about 0.01–0.05 ng/mL [Citation13]. PCT levels rise significantly within 2–6 h after bacterial infections, peak in 12 h [Citation16], and drop by 50% in 24 h or longer even in the presence of host immune response and antibiotic treatment [Citation17–20]. A number of meta-analyses have evaluated the diagnostic value of serum PCT. For example, a meta-analysis of 12 studies confirmed that serum PCT achieved significantly higher sensitivity (88% vs. 75%) and specificity (81% vs. 67%) in detecting bacterial infections compared to C-reactive protein (CRP) [Citation21]. Another study showed that PCT was equally effective in detecting bacterial infections in patients with systemic autoimmune diseases, and its levels were not affected by corticosteroids or immunosuppressive agents; in general, PCT was quite specific for detecting infectious complications, with an area under the summary receiver operating characteristic curve of 0.91 (95% confidence interval [95%CI] 88–93) for PCT [Citation22]. Increased PCT levels may also be caused by noninfectious diseases and by other microbial infections [Citation23]; however, the PCT levels under noninfectious conditions increase only slightly [Citation24]. Detailed summaries on the diagnostic efficacy of PCT for different pathogens are described in .
Table 1. Overview of common pathogens and their associations with procalcitonin.
Diagnosing infection is of great significance for cancer patients, transplant recipients, and other immune-deficient groups. The immune response in cancer patients is slower than in controls, resulting in a slow increase in the PCT levels from baseline, which is already minimally higher in these patients [Citation14]. Several studies in patients with non-neutropenic solid tumor and fever have shown that baseline PCT is less valuable in distinguishing infectious from noninfectious etiologies in febrile patients [Citation54]. However, changes in PCT levels may help to distinguish infection-related and tumor-related fever [Citation14]. For example, in septic cases, the PCT levels during follow-up on days 4–7 of antimicrobial treatment were significantly lower than the baseline, while they remained unchanged in tumor fever [Citation14]. Procalcitonin is also of diagnostic value in hematological patients with febrile neutropenia; 0.56 ng/mL (specificity 76.8%) was determined to be the optimal cutoff value for distinguishing Gram-negative (G–) bloodstream infection (BSI) from other factors that cause fever [Citation34]. In patients with malignant tumors, extensive chemotherapy suppresses the immune system [Citation13], and PCT could help to rule out blood infections in such patients. For patients with suspected sepsis, a cutoff value of 1.17 ng/mL was used to exclude blood infections with high diagnostic efficiency (area under the curve (AUC) = 0.741, 95%CI 68.2–79.4, sensitivity 66.67%, specificity 80.08%, positive predictive value (PPV) 17.34%, negative predictive value (NPV) 97.46%) [Citation13].
A study by Sato et al. showed that bacteremic liver transplant patients had significantly higher PCT levels than non-bacteremic patients. They concluded that PCT could effectively distinguish between bacteremic patients and non-infected patients with immune rejection after transplantation [Citation55]. In addition to liver transplantation, PCT levels were found to distinguish pneumonia and allograft rejection in lung transplant patients [Citation56]. A study by Leli et al. confirmed that PCT had good diagnostic accuracy in predicting bacteremia in patients with suspected sepsis (AUC = 0.876, p = 0.12), and was better than CRP (AUC = 0.602, p < 0.05) [Citation57]. In addition, low PCT levels (<0.4 ng/mL) have been shown to rule out bacteremia [Citation58].
Sepsis has a high mortality rate and is the most common cause of death in the non-coronary intensive care unit (ICU) [Citation59]. Therefore, its early diagnosis is usually essential for successful treatment [Citation60]. PCT can be used to distinguish between sepsis and non-sepsis, and its levels also correlate with the severity of sepsis [Citation61]. Wacker et al. shown that PCT was useful in diagnosing critically ill patients with sepsis (AUC 0.85, 95%CI 81–88), sensitivity 0.77 (95%CI 72–81), a specificity 0.79 (95%CI 74–84) [Citation62]. Furthermore, Cong et al. concluded that, compared to non-severely ill patients, the diagnostic value of PCT was even better in severely ill patients [Citation63]. PCT is a good biomarker for infectious diseases in critically ill patients with sepsis, severe sepsis and septic shock. Compared with CRP, PCT showed better accuracy in diagnosing sepsis in severely ill adults and after surgery or trauma (0.78 vs. 0.71, p = 0.02) [Citation64].
Altogether, serum PCT has high sensitivity, good specificity, few influencing factors, a wide range of applicability and high efficiency in diagnosing patients with sepsis (). Many studies have confirmed that the clinical use of PCT has several advantages, such as rapid diagnosis of infection and accurate risk classification, thereby allowing optimized treatment that benefits patients and reduces costs [Citation65–69]. These advantages are reflected not only in outpatients [Citation68,Citation69] but also in the emergency department [Citation65–67]. Although the specific cutoff values of the same disease in different studies may differ due to the heterogeneity of study designs and other factors, it is clinically feasible to judge roughly the type of disease based on the degree of elevation of PCT and provide general directions for clinical decision-making to achieve early symptomatic treatment [Citation14].
Disease monitoring and evaluation
Several studies have demonstrated that PCT follows a “dose-response” gradient that correlates with the severity of sepsis [Citation4]. In general, PCT increases slightly in patients with local bacterial infection but increases significantly among those with invasive infection causing SIRS, sepsis, and septic shock [Citation4]. In a study on the acute inflammatory response of patients with Pseudomonas aeruginosa in the ICU, PCT was shown to be an important marker of the intensity of the inflammatory response and the severity of the P. aeruginosa infection. The serum PCT levels were more elevated than CRP and cytokines, rose earlier and remained high longer [Citation70]. For special cases such as leukopenic patients, serum PCT levels could be used to predict death. In a prospective study in hematology patients with febrile neutropenia, the cutoff value of PCT at admission of 0.46 ng/mL predicted the risk of septic shock and death [Citation71].
The use of PCT for prognosis is controversial [Citation72]. A study on children with sepsis shown that PCT could not distinguish whether children with sepsis were infectious due to the overlap of the PCT distribution in the children with and without shock, and it was not possible to predict the future survival of children with septic shock [Citation4]. However, another study confirmed that elevated PCT in children with septic shock had prognostic value [Citation73]. Elevated PCT levels at admission were related to severity of organ failure and mortality among the children. A decline in PCT after 24 h had a good prognostic significance [Citation73]. A prospective study also revealed similar findings although with the caveat that, while a single serum PCT value had no obvious prognostic value, monitoring PCT kinetics could predict mortality [Citation70]. In addition, PCT had prognostic value in immunodeficient cancer patients. Debiane et al. found that dynamic monitoring of PCT for 4–7 days effectively predicted the mortality in the next two months of immunodeficient cancer patients [Citation14]. A more in-depth and effective evaluation of PCT is needed, with attention paid to the homogeneity of the study design and appropriate expansion of the sample size.
Guiding medical decision-making
Bacterial infection is not always the causative factor of sepsis. In adult patients, bacterial infection accounts for about 50% of sepsis, while in children, bacterial and fungal infections account for 43% of sepsis [Citation4]. Thus, the use of antibiotics in 30–50% of hospitalized patients is unnecessary [Citation11]. Overuse of antibiotics has serious consequences, among them the emergence of antibiotic-resistant strains, side effects caused by adverse drug reactions and antibiotic-associated colitis [Citation74]. Therefore, it is important to seek effective biomarkers to guide the rational use of antibiotics in clinical practice [Citation74].
A PCT-guided antibiotic algorithm can shorten the duration of antibiotic exposure without endangering patient safety, especially in respiratory disease patients with lower respiratory tract infection symptoms and community-acquired pneumonia (CAP) [Citation75]. The meta-analysis of Schuetz et al. showed that PCT improved the management of antibiotics in patients with acute respiratory infections and had a major impact on the reduction of antibiotic exposure and the risk of antibiotic resistance [Citation76]. However, they also stated that, depending on the clinical environment, there are differences in the application of PCT between trials. Therefore, the clinical environment and patient risks should also be considered in clinical practice [Citation76].
Continuous monitoring of PCT can assess the progression of sepsis and the 28-day risk of death and reduce mortality [Citation11]. For example, a study of sepsis in children showed that continuous PCT monitoring could help guide the duration of antibiotics and achieve safe antibiotic de-escalation [Citation4]. Studies on cancer patients confirmed that monitoring PCT to guide antibiotic therapy in sepsis could shorten the duration of antibiotic therapy while avoiding the complications and side effects of prolonged antibiotic use and reducing mortality [Citation77,Citation78]. Other studies have confirmed that PCT plays a role in the antimicrobial stewardship of febrile cancer patients by decreasing the duration of antimicrobial therapy and the amount of antibiotics given in the course of the treatment [Citation14,Citation79].
PCT-guided antibiotic treatment is used mainly in two situations. First, for patients with stable and low-risk respiratory infections, a PCT level < 0.25 ng/mL can guide the withdrawal of antibiotics [Citation11]. Second, for critically ill patients with suspected sepsis, antibiotics could not be stopped initially, but a PCT level < 0.5 ng/mL or a drop of ≥80% from the peak level could guide discontinuation in patients who have stabilized [Citation4].
PCT-guided antibiotic stewardship has a number of advantages. First, it reduces the risk of development of drug-resistant bacteria. Second, it reduces hospital length of stay, therapeutic costs, and drug waste without any side effects [Citation80,Citation81]. Third, it allows clinicians to formulate individualized antibiotic treatment plans and adjust the antibiotic dosage over time based on objective and reliable PCT values [Citation75]. However, PCT-guided antibiotic stewardship also has limitations, such as false positive and false negative results [Citation11]. As well, despite many investigations, the value of PCT in guiding the use of other drugs is unclear. In the future, the value of PCT in guiding the use of anti-inflammatory and chemotherapeutic drugs should be explored.
Procalcitonin and pathogenic microorganisms
When pathogenic microorganisms infect the human body, serum PCT is stimulated by LPS and cytokines, and the degree of increase in PCT depends on the type of microorganisms [Citation79]. An increase in PCT does not affect the level of calcitonin [Citation82]. In virus-induced SIRS, the change in PCT level is not statistically significant or it increases only slightly [Citation4], while in severe bacterial infections with systemic symptoms, the PCT level increase significantly (> 100 ng/mL) [Citation5]. Moreover, the increase in PCT level caused by different types of bacteria is distinct. A study by Thomas-Rüddel et al. study showed that the PCT concentration in patients with G– bacteremia (26 ng/mL) was significantly higher than in patients with Gram-positive (G+) bacteremia (7.1 ng/mL) [Citation83]. PCT levels of Escherichia coli and other Enterobacteriaceae in blood cultures were three times that of other pathogens; however, some G+ bacteria, such as Streptococcus pneumoniae, could cause a significant PCT increase comparable to G– bacteria [Citation83]. Also, this group found that the infection sites of pathogenic microorganisms were a reason for different elevations of serum PCT levels. Patients with urinary system infection had the highest PCT, followed by those with abdominal infections (levels were twice the normal value), and patients with respiratory tract infections had the least increase in PCT. Higher levels of PCT caused by abdominal infections may be related to higher bacterial loads, because the lymph in the abdominal cavity flows directly into the systemic circulation, while the venous blood in the abdominal organs flows to the liver [Citation83]. Another study showed that patients with positive blood cultures had the highest PCT level (8.2 ± 24.1 ng/mL), followed by patients with positive urine cultures (4.6 ± 16 ng/mL) and patients with positive endotracheal aspirates, who had the lowest level (1.93 ± 11.4 ng/mL) [Citation74]; these findings were consistent with results from other studies [Citation11,Citation84,Citation85].
Bacteria
When bacteria invade the human body, the serum PCT level is significantly increased. The bacteria induce the release of PCT mainly in two ways: in the direct way, PCT is stimulated by LPS or other toxic metabolites of the microorganisms; in the indirect way, the specific pathogen stimulates the body to produce inflammatory mediators, including interleukin (IL)-1beta, IL-6, IL-8 and tumor necrosis factor (TNF)-alpha [Citation16,Citation86,Citation87], which induce target cells to produce PCT (). Pathogens have different virulence mechanisms that lead to other host inflammatory responses [Citation88], and differences in the specific signaling of these pathogens lead to different serum PCT concentrations [Citation83,Citation89].
Figure 2. The specific mechanism of PCT variation caused by different pathogenic microorganisms. (a) Staphylococcus aureus activates TLR2 and TLR4 on monocytes and granulocytes through LTA to stimulate the production of cytokines, including TNF-alpha, and these cytokines ultimately promote the release of PCT. (b) The surface molecule LPS of Escherichia coli acts on macrophages and neutrophils, and promotes the production of IL-1, IL-6, TNF-alpha and other cytokines through the TLR4/NF-κB pathway. These inflammatory mediators such as bacterial endotoxin and interleukin can stimulate the secretion of PCT. (c) Group A Streptococcus activates the TLR2 and TLR4 receptors of macrophages, which activates the NF-κB pathway and stimulates the production of IL-1, IL-6, IL-8, TNF-alpha and other cytokines. (d) Streptococcus pneumoniae induces macrophage NF-κB translocation by activating TLR4, supplemented by cell wall components to activate TLR2, thereby stimulating the secretion of TNF-alpha inflammatory cytokines to promote the release of PCT. (e) Pathogens have different virulence mechanisms, leading to different host inflammatory responses, and differences in these pathogens’ specific signaling lead to different serum PCT concentrations, according to the data from . (f) When a bacterial infection occurs, the release of LPS from bacteria and the cytokines stimulated by it can induce the expression of CALC-I in the extra-thyroid organs and cells such as liver, pancreas, kidney, lung, intestine and within leukocytes, leading to a sharp increase of PCT levels.
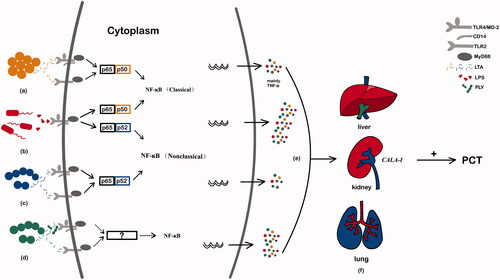
With Gram stains, bacteria can be divided into two groups: G+ and G– bacteria. The main difference between them is the structural components of the cell wall. LPS is found in G– bacteria, and lipoteichoic acid (LTA) is found in G+ bacteria. LPS and LTA, as pathogen-associated molecular patterns (PAMPs), are recognized by the innate immune system through pattern-recognition receptors [Citation33], which can initiate signaling pathways and induce the release of pro-inflammatory cytokines. Pattern-recognition receptors include the members of the Toll-like receptor (TLR) and C-type lectin receptor (CLR) families [Citation8]. Studies have shown that G+ and G– bacteria activate different TLR signaling pathways, which produce various pro-inflammatory cytokines and stimulate different levels of PCT release [Citation90,Citation91]. G– bacteria activate mainly the TLR4/myeloid differentiation factor-2 (MD-2) complex and its co-receptor, membrane-bound CD14 (mCD14) on leukocytes, through LPS (shed or membrane-bound), which is recognized by cells of the innate immune system. In contrast, G+ bacteria activate TLR2 by contacting with LTA, soluble extracellular toxins, and membrane peptidoglycan and activate TLR4 to a more limited extent [Citation92]. Activation of TLRs by PAMPs triggers intracellular signaling cascades, which converge through common adaptor molecules onto three transcription factors: NF-kappaB (NF-κB), activation protein-1 and interferon response factor-1. These three factors all lead to the up-regulation of the genes of pro-inflammatory cytokines, TNF-alpha, IL-1 and interferon (IFN) and result in varying degrees of change in PCT levels [Citation93]. Both TLR2 and TLR4 are members of the IL-1 receptor superfamily and activate IL-1 receptor-related kinases, so the intracellular signaling pathways are generally similar [Citation92]. However, TLR4 appears to be unique, because the intracellular signals mediated by it can occur through both the myeloid differentiation factor 88 (MyD88, a TLR-related adaptor protein)-dependent and -independent pathways [Citation92]. In addition, although the activation of NF-κB and mitogen-activated protein kinase (MAPK) family members caused by TLR2 and TLR4 on dendritic cells is similar, the TLR2 and TLR4 signals are different as they result in transcription of significantly different cytokines and chemokine genes [Citation94]. Therefore, the dominant activation of TLR4 by G– bacteria and the dominant activation of TLR2 by G+ bacteria induce different inflammatory cytokines and different gene expression patterns of leukocytes in vitro [Citation83]. The activation of TLR4 causes strong release of inflammatory cytokines, including TNF-alpha, IL-1, and IL-6, which promote gene transcription and lead to increased PCT secretion by tissues outside the thyroid gland and a sudden increase in serum PCT levels. On the other hand, the activation of TLR2 has a relatively weak stimulating effect on inducing cytokine release, and this effect is indirect, leading to an insignificant increase in PCT levels [Citation37]. These results are consistent with previous studies. For example, a recent study has shown that G– bacterial infection caused higher IL-1, IL-6, and IL-10 levels than G+ bacterial infection, causing a stronger inflammatory response [Citation16]. A study by Thomas-Rüddel et al. shown that elevated IL-6 and IL-8 in the serum of patients with G– bacteremia caused a higher elevation of PCT than G+ bacteremia [Citation83]. In addition, studies have pointed out that the pathogenic substance of G+ cocci is exotoxin, and the main component of exotoxin is a protein that is extremely unstable and easily damaged. This is one reason why the serum PCT level caused by G+ bacterial infection is lower than that of G– bacteria [Citation6]. However, basic research on the specific mechanisms of different pathogen infections leading to different PCT levels is still lacking.
Gram-positive bacteria
Staphylococcus aureus
Staphylococcus aureus is a common cause of bacteremia, infective endocarditis (IE), osteoarticular, skin and soft tissue infections, pleuropulmonary, and device-related infections [Citation95]. It activates TLR2 and TLR4 on monocytes and granulocytes through LTA to stimulate the production of cytokines, including TNF-alpha. These cytokines ultimately promote the release of PCT [Citation56,Citation96]. Although both TLR2 and TLR4 are involved in the identification process of pathogens, the production of IL-1beta and IL-6 during S. aureus infection is mostly dependent on TLR2 [Citation56]. When S. aureus infects the human body, it induces the p65-p50 heterodimer NF-κB classical pathway, activating the inflammatory signal transcription factor, NF-κB. Signals emanating from pattern-recognition receptors and receptors of members of the TNF and IL-1 cytokine families activate the transcription factor, NF-κB, which is the main regulator of innate immunity, adaptive immunity, and inflammatory response. Different types of NF-κB dimers target other genes and are transcribed into different proteins that play a role in innate immunity. In fact, S. aureus induces a stronger p65 nuclear translocation (stronger than Group A Streptococcus [GAS]), and the activation of nuclear factor p65 leads mainly to the production of pro-inflammatory factors that stimulate the release of TNF-alpha, IL-1, and IFN, so S. aureus leads to a strong inflammatory response [Citation56]. Several studies have shown that TNF-alpha is the main mediator for S. aureus to stimulate monocytes and granulocytes to produce PCT. When TNF-alpha is neutralized by anti-TNF-alpha, S. aureus cannot stimulate intracellular synthesis of PCT, and the serum PCT does not increase [Citation97]. Balog et al. confirmed that S. aureus, as a TNF-alpha inducer, increased the expression of PCT in human peripheral blood monocytes and granulocytes [Citation97]. In conclusion, when S. aureus invades the body, LTA, a component of S. aureus, promotes the release of TNF-alpha from human monocytes and granulocytes, thereby causing an increase in serum PCT [Citation97].
Serum PCT has the potential to identify endocarditis caused by S. aureus. When S. aureus invades the human body, the increase in PCT is lower than that of G– bacteria such as E. coli [Citation56,Citation98,Citation99]. The destruction of the heart’s endothelium leads to bacterial infections that may be due to direct invasion or inflammation (e.g. injected particulate matter and secondary onset of heart disease), and eventually result in the formation of sterile vegetations, and IE stems from their colonization [Citation95]. The PCT levels of IE patients caused by different pathogenic bacterial infections differ significantly. Among them, the PCT value of S. aureus bacteremia (n = 7) is significantly higher than that of IE patients with other types of bacteremia (n = 8) (13.1 vs. 0.435, p = 0.0299) [Citation100]. S. aureus and Streptococcus viridans are both common pathogenic causes of IE. The PCT value of patients with S. aureus bacteremia is very high, and the PCT value of patients with S. viridans bacteremia is very low [Citation100]. The study by Jereb et al. confirmed that among IE patients, patients infected by S. aureus had the highest PCT levels [Citation101]. Further studies have shown that PCT > 0.5 ng/mL was the best predictor of adverse outcomes, and that it increased the suspicion of S. aureus as a pathogen. Then, more attention should be paid to the complications of IE and more stringent medical follow-up is necessary, while a PCT level < 0.5 ng/mL indicates low risk and means that staphylococcal infection is unlikely to be present [Citation102]. Thus, PCT has the potential to identify the patients with IE caused by S. aureus infection. Tascini et al. have also shown that in patients admitted for IE, high levels of PCT indicated an increased likelihood of S. aureus infection (AUC = 0.727, 95%CI 64.5–81.0) [Citation26]. Patients with inflammatory biomarkers above the optimal cutoffs (WBC ≥12,800/mm3, CRP ≥ 130 mg/L, PCT ≥1.7 ng/mL) were almost 20 times more likely to be infected with S. aureus than patients with all biomarkers below these cutoffs [Citation26]. In addition, William et al. have evaluated the performance of PCT in distinguishing Staphylococcus aureus bacteremia in blood cultures containing clusters of G+ cocci and found that the optimal cutoff level of PCT was 0.5 ng/mL (AUC = 0.65, 95%CI 52–78, sensitivity 63%, specificity 64%) [Citation25]. However, some studies have shown no significant difference in plasma PCT levels among patients with sepsis caused by different pathogens. For example, a study by Feezor et al. on pro-inflammatory cytokine profiles of patients with G– and G+ bacterial infections showed no significant difference in serum PCT levels between patients with G– and G+ sepsis. In addition, TNF-alpha, IL-1 receptor antagonists, IL-8, IL-10, IL-18 binding protein, and protein C showed no significant differences [Citation92]. Because the total number of patients in this study was relatively small (n = 52), it had limitations, and further studies are needed to explain these findings.
Coagulase-negative Staphylococcus
Coagulase-negative Staphylococcus (CoNS) is a skin symbiotic bacterium that can maintain a stable environment in the human body. It is also the main pathogen causing nosocomial infections. The most common pathogenic species, Staphylococcus epidermidis, Staphylococcus haemolyticus, Staphylococcus saprophyticus, Streptococcus capitis and Staphylococcus lutonus [Citation103], often cause prosthetic valve endocarditis, urinary tract and central nervous system infections, surgical or implanted medical device infections and bacteremia [Citation104]. The main source of endogenous infection is the colonization of CoNS in different parts of the host skin and mucosa, while the route of transmission is through medical and/or nursing procedures [Citation104]. Invasive procedures in the ICU spread the infection of S. epidermidis. S. epidermidis forms a protective layer of mucopolysaccharide on silicon to protect it from the immune system and drugs [Citation105]. Research by Białecka et al. has shown that intradermal injection of S. epidermidis in animal models does not cause local inflammation [Citation106]. S. epidermidis is low in virulence; most strains do not produce exotoxin and they evade host defenses by forming a biofilm instead of attacking the host by producing toxins. Rewa et al. believed that the low virulence may explain why PCT and other inflammatory markers of patients with S. epidermidis bacteremia do not increase [Citation107].
CoNS is the most common pathogen associated with neonatal nosocomial infection in the neonatal ICU [Citation108], and such infections have high morbidity (17–25%) and mortality rates (17–72%) [Citation105]; they also cause high hospital admission rates and prolonged hospitalization in the neonatal ICU [Citation108]. PCT levels may help to distinguish between CoNS and G– bacilli infections [Citation105]. In a study on neonatal hospital infections, PCT was the only parameter measured that increased in sepsis caused by G+ bacteria. At the same time, CRP, WBC, and the immature-to-total neutrophil ratio could not be used to identify G+ infections [Citation105]. Chiesa et al. also found that, compared with G– bacteria, CoNS caused a lower degree of PCT elevation in patients with sepsis [Citation109]. These studies all confirmed the role of PCT in the diagnosis of neonatal nosocomial coagulase-negative staphylococcal infection. In addition, PCT may help to differentiate S. aureus from CoNS in clustered G+ bacteremia. The PCT level of patients with S. aureus bacteremia was significantly higher than those with CoNS isolated from blood cultures (p = 0.29) [Citation25].
Whether PCT can distinguish between blood sample contamination and true bacteremia among CoNS patients remains controversial. The study by Shomali et al. showed that PCT could not distinguish between blood sample contamination (median 0.24 ng/mL) and true bacteremia (median 0.26 ng/mL) (p = 0.51) [Citation25]. In contrast, another study showed that PCT had diagnostic value in distinguishing blood contamination and BSI caused by CoNS. The PCT concentrations of blood culture of BSI patients at −1, 0, and +1 days was significantly higher than those of patients with blood contamination (p < 0.05), and PCT had a high sensitivity and high specificity at a cutoff of 1 ng/mL (sensitivity 86% and 100% on day −1 and 0, specificity 60% and 80% on day −1 and 0) in the diagnosis of BSI caused by CoNS, which could help to distinguish BSI caused by blood contamination and CoNS [Citation28]. In fact, the positive blood culture of CoNS was still considered to be sample contamination. However, while positive cultures of full-term newborns and older infants may be due to sample contamination, in neonates with very low weight, positive cultures are frequently caused by bacteremia [Citation105]. In addition, PCT < 0.5 ng/mL could not rule out bacteremia because bacteremia caused by CoNS might not increase serum PCT levels [Citation27]. For example, an observational study on PCT and CoNS infections in the ICU demonstrated that, as the most common microorganism in catheter-associated bloodstream infections, the elevated level of serum PCT during CoNS infection was lower and similar to that of culture-negative patients (1.0 U/L vs. 0.9 U/L [p = 0.80]), and much lower than the level in the known pathogen group (4.6 U/L) [Citation107] [note U/L here is the same as ng/mL (editorial comment)]. Therefore, although PCT is an important auxiliary indicator for diagnosing bacterial sepsis caused by CoNS, microbial culture remains the gold standard [Citation108].
Streptococcus pneumoniae
Streptococcus pneumoniae is the main pathogen causing bacterial pneumonia, otitis media, meningitis, and sepsis, and its infection is an important health problem in the world [Citation110]. The main virulence factors of S. pneumoniae are its capsule and hemolysin, which help S. pneumoniae to infect the body and are necessary for immune evasion against the host complement system. Hemolysin is important in obtaining nutrients from host cells and the alveolar cavity, thereby promoting the host inflammatory response and increasing the possibility of transmission [Citation110]. Hemolysin, a cytoplasmic protein that is a strong but thermally unstable macrophage agonist [Citation97], induces macrophage NF-κB translocation by activating TLR4, and is supplemented by cell wall components that activate TLR2, which stimulates the secretion by TNF-alpha of inflammatory cytokines that promote the release of PCT [Citation111]. The importance of TLR2 in identifying G+ bacteria through cell wall and cell membrane components such as peptidoglycan, LTA and lipoprotein has been demonstrated [Citation92]. Although the cell wall components of S. pneumoniae are recognized by TLR2, the inflammatory response induced by hemolysin does not depend on this receptor but is similar to that of LPS, and the hemolysin-induced NF-κB translocation in macrophages requires TLR4 [Citation111]. Moreover, the similarity of the sepsis syndrome caused by S. pneumoniae and G– bacteria may be due to the involvement of the same signal-transduction receptor, TLR4, in combination with TLR2-mediated stimulation by cell-wall components of lysed pneumococci, which further challenges the generally accepted conclusion that the main ligand of TLR4 is a product of G– bacteria [Citation111].
S. pneumoniae is the most common pathogen of CAP [Citation112]. Studies have shown that in patients with severe CAP, the increase in PCT level is higher in those infected by S. pneumoniae than by atypical pathogens such as Legionella [Citation30]. One study demonstrated that 8 out of 9 cases of atypical pathogenic pneumonia (Mycoplasma pneumoniae (MP), Chlamydia psittaci, Chlamydia pneumoniae, Pseudomonas carinii, respiratory syncytial virus (RSV)) had PCT < 0.5 ng/mL, and 6 out of 27 cases of typical pathogenic pneumonia (S. pneumoniae, Haemophilus influenzae, S. aureus, Streptococcus pyogenes) had PCT <0.5 ng/mL (p = 0.03) [Citation113]. Another study had similar findings: the PCT level (mean ± standard error of the mean, 6.76 ± 1.74 ng/mL) in patients with Legionella pneumonia on admission was significantly lower than that of patients with S. pneumoniae pneumonia (20.94 ± 3.99 ng/mL) (p < 0.01) [Citation114]. S. pneumoniae is one of the most common pathogens of bacterial meningitis in adults [Citation115]. The meta-analysis of Henry et al. demonstrated that in children with suspected meningitis, serum PCT testing could quickly distinguish between bacterial meningitis and viral meningitis (AUC = 0.97, sensitivity 0.96, specificity 0.89) [Citation116]. A retrospective study showed that PCT ≤ 1.27 ng/mL could independently differentiate tuberculous meningitis from bacterial meningitis (sensitivity 96.2%, specificity 62.9%, PPV 49.0%, NPV 97.8%) [Citation117]. However, there was no significant difference between the level of PCT in patients with tuberculous meningitis and viral meningitis, so PCT could not distinguish between them [Citation117].
Group A Streptococcus
Streptococcus pyogenes (GAS) can cause various human diseases from superficial skin infections to fatal syndromes. GAS usually persists in the body through immune escape and can cause repeated infections [Citation56]. It activates the TLR2 and TLR4 receptors of macrophages, activates the NF-κB pathway, and stimulates the production of IL-1, IL-6, IL-8, TNF-alpha, and other cytokines [Citation56]. These inflammatory cytokines promote gene transcription and cause a sudden increase in serum PCT levels from tissues outside the thyroid gland [Citation37]. Oberhoffer et al. showed that LPS and sepsis-related cytokines increased the expression of PCT in human peripheral blood mononuclear cells [Citation118]. However, research is lacking on a direct connection between GAS infection and elevated serum PCT. Compared with S. aureus and E. coli, GAS induces less p65 nuclear translocation in macrophages. It activates the non-classical NF-κB activation pathway, p65-p52, which leads to a lower activity of NF-κB and lower expression of pro-inflammatory factors in macrophages than in those infected by S. aureus and E. coli. These factors may lead to a weakened inflammatory response [Citation56].
A PCT threshold for the identification of streptococcal infection has not been demonstrated. However, among patients with a diagnosis of erysipelas or deep vein thrombosis, PCT >0.25 ng/mL can be used to identify erysipelas, which is caused mainly by streptococcus (AUC = 0.76, sensitivity 37.1%, specificity 90.0%) [Citation29]. In the future, the diagnostic threshold of PCT for streptococcal infection should be determined.
Other gram-positive bacteria
Although Streptococcus sophia (common in wound infections and bacteremia), Streptococcus bovis (common in sepsis and IE), and Streptococcus mutans (common in caries) can be seen in patients, there are few studies on PCT and other G+ bacteria, including Enterococcus faecalis, Enterococcus faecium, Streptococcus pyogenes, Streptococcus esculentus, Listeria monocytogenes, Streptococcus paraanguillae, Streptococcus agalactiae, Streptococcus mutans, Streptococcus bovis, Streptococcus sanguis, and Propionibacterium acnes. We conducted a literature search in PubMed with the keywords "procalcitonin" (title/abstract search) and the name of each strain mentioned above (title/abstract search) for original research and review articles but found no relevant literature. However, a recent case report on the identification of E. faecalis by gene sequencing in patients with urinary tract infection mentioned PCT [Citation119].
Gram-negative bacteria
Escherichia coli
Escherichia coli is usually a harmless gastrointestinal symbiotic bacterium, but it still has the ability to cause disease. Its pathogenic substances include fimbriae (adhesin that mediates the initial attachment to the host intestinal epithelial cells), endotoxin, and enterotoxin [Citation120]. Pathogenic E. coli can cause severe diarrhea and extraintestinal diseases that can invade from the gastrointestinal tract to extraintestinal sites (e.g. urinary tract, blood and central nervous system) [Citation120]. The LPS surface molecule of E. coli acts on macrophages and neutrophils and promotes the production of IL-1, IL-6, TNF-alpha and other cytokines through the TLR4/NF-κB pathway. These inflammatory mediators, bacterial endotoxin and interleukin can stimulate the secretion of PCT by neuroendocrine cells of the spleen, liver, lung, and kidney [Citation6,Citation98]. LPS is the key factor in the difference in PCT expression between E. coli and S. aureus bacteremia. One study demonstrated that, after E. coli LPS acted ex vivo on differentiated adipocytes for 6 h, the secretion of PCT in the culture supernatant increased 13.5-fold [Citation121]. Elson et al. showed that E. coli infection of the human body strongly stimulated cells expressing TLR4/MD-2 and induced elevated levels of PCT [Citation122]. LPS binds to TLR4, activates the NF-κB pathway, induces p65, and stimulates the release of more pro-inflammatory factors that promote the production of PCT. They, therefore, can induce the human body to release a large amount of PCT into the blood.
Compared with S. aureus, which induces the p65-p50 heterodimer to activate NF-κB, E. coli induces both the p65-p50 and p65-p52 heterodimers to activate NF-κB in macrophages [Citation56]. Because p65 activation is related to pro-inflammatory factors such as TNF-alpha and IL-1, E. coli produces more cytokines [Citation56], leading to a greater increase in PCT with E. coli than with S. aureus. In addition, studies have shown that E. coli stimulation of the body to produce higher levels of PCT is also related to IL-6 [Citation35]. Yan et al. showed that E. coli stimulated human umbilical vein endothelial cells to produce more IL-6 than P. aeruginosa (104 cells/mL vs. 106 cells/mL) and therefore induced higher levels of PCT [Citation35].
Serum PCT can help to distinguish E. coli sepsis from other bacterial sepsis [Citation33]. There are significant differences in PCT levels between different pathogenic bacteria groups, with E. coli, Streptococcus spp. and other Enterobacteriaceae having the highest PCT concentrations [Citation30]. The PCT levels of bacteremia patients with G– bacilli (19.50 ng/mL) such as E. coli (32.5 ng/mL) was significantly higher than that of G+ bacilli (8.45 ng/mL) and G+ cocci (9.21 ng/mL) (p < 0.01) [Citation123]. Li et al. found that the optimal cutoff value to identify E. coli sepsis was 2.32 ng/mL (AUC = 0.778, 95%CI 71.1–84.5, sensitivity 76.2%, specificity 65.4%, PPV 36.6%, NPV 91.5%) [Citation33]. Therefore, a higher PCT concentration may indicate E. coli infection, but K. pneumoniae infection cannot be ruled out. Li et al. also showed that the optimal cutoff value for K. pneumoniae was 2.81 ng/mL (AUC = 0.859, 95%CI 80.0–91.7, sensitivity 89.3%, specificity 69.4%, PPV 25.5%, NPV 98.3%) [Citation33].
Other gram-negative bacteria
The virulence of P. aeruginosa depends on the various exotoxins (exotoxin A, exoenzyme S, elastase, etc.) it produces and the LPS in its outer membrane. Its pathogenic mechanism depends partly on exotoxin or endotoxin, but more importantly on the interaction between bacterial cells and nonspecific immune host cells [Citation124]. Studies have shown that PCT levels are not different between multidrug-resistant P. aeruginosa and non-drug-resistant P. aeruginosa, and monocytes may be a possible source of PCT release in inflammation [Citation124]. A study on the sepsis mouse model of burn wounds infected with P. aeruginosa indicated that the endotoxin produced at the site of the infection could induce the release of PCT [Citation17]. A prospective single-center study showed that P. aeruginosa was an important factor for infection in patients with chronic kidney disease and that a PCT level ≥ 0.85 ng/mL could help in the early identification of infections in these patients [Citation125].
Acinetobacter baumannii is a conditional pathogen of hospital-acquired pneumonia. However, there have been no reliable serum markers to distinguish A. baumannii infection from colonization. A study in 2018 pointed out that the dynamic secretion of a potential biomarker, sTREM-1, was increased in A. baumannii infection in the lungs and that the serum sTREM-1 concentration was positively correlated with the serum PCT level [Citation126]. However, research is lacking on a connection between PCT and A. baumannii.
There is also little research on the relationship between PCT and Enterobacter cloacae, Serratia, Klebsiella oxytoca, Salmonella typhi, Enterobacter aerogenes, Citrobacter, Stenomonas maltophilia, Bacteroides fragilis and other common G– bacteria. A recent study on PCT, sTREM-1, and CRP in diagnosing enteric fever caused by Salmonella typhi showed that the sensitivity and specificity of PCT for the diagnosis were 97.7% and 82.5%, respectively [Citation127], but the mechanism that causes the PCT increase is still unknown.
Fungi
Although there is little difference in the increase of serum PCT concentration when fungal and G+ sepsis occurs, the mechanisms that cause the rise of PCT differ. Unlike bacteria, the fungal pattern-recognition receptors are CLR instead of TLR [Citation128]. Activation of the CLR/Syk-CARD9 signaling pathway induces the secretion of IL-1beta, IL-10, and IL-6 and other cytokines. It promotes the release of PCT but causes a more modest increase in the level of PCT than bacterial infections [Citation33,Citation83]. In addition, the level of serum IFN-gamma in patients with fungal infection was significantly increased [Citation129], which could inhibit the secretion of PCT [Citation121]; thus, the concentration of PCT in fungal sepsis could be regulated by the level of IFN-gamma [Citation33]. There is no significant increase in serum PCT during fungal infections. There has been little research on the relationship between Candida albicans, Aspergillus, and other common strains and PCT.
Low concentrations of PCT in critically ill patients may indicate a fungal infection. The PCT level can be used to distinguish G– sepsis from fungal sepsis with high sensitivity and specificity. In one study, the optimal cutoff value was 3.11 ng/mL (sensitivity 63.9%, specificity 93.3%) [Citation33]. Leli et al. used a PCT cutoff value of 1.6 ng/mL (sensitivity 77%, specificity 96%) to distinguish G– bacterial infections from fungal infections [Citation31]. Several studies have proposed cutoff values of PCT for the identification of fungal sepsis [Citation32,Citation38–41,Citation130]. In critically ill septic patients outside the ICU, PCT ≥2.5 ng/mL could identify Candida spp. (AUC = 0.76, 95%CI 68–84, sensitivity 78.3%, specificity 72%, PPV 15.1%, NPV 98.3%) [Citation38]. In ICU patients, the diagnostic role of PCT is still applicable. Cortegiani et al. showed that a cutoff of ≤6.08 ng/mL for PCT could distinguish Candida spp. from bacteria in blood cultures (AUC = 0.93, 95%CI 89–96, sensitivity 86.8%, specificity 87.4%, PPV 63.9%, NPV 96.3%) [Citation41]. A prospective observational study reported that the PCT cutoff value to distinguish Candida sepsis from bacterial sepsis was 2 ng/mL (AUC = 0.97, sensitivity 92%, specificity 93%, PPV 94%, NPV 94%) [Citation39]. Evidence also suggests that PCT levels higher than 5.5 ng/mL have high diagnostic power for candidemia-related sepsis (AUC = 0.96, PPV 65.2%, NPV 100%) [Citation40]. However, a study by Li et al. showed that the difference between the serum PCT levels of patients with G+ sepsis (0.48 ng/mL [interquartile range 0.15–2.16 ng/mL]) and fungal sepsis (0.60 ng/mL [interquartile range 0.14 – 2.06 ng/mL]) was not statistically significant (p = 0.602) [Citation33]. A systematic review showed that PCT could not be used as an independent indicator to distinguish candidemia and bacterial bacteremia, but when PCT was used in combination with other infection biomarkers (such as beta-D-glucan), it could improve the diagnostic performance of candidemia [Citation79].
Viruses
When a virus infects the body, the serum PCT level remains unchanged or increases slightly. Studies have shown that the concentration of IFN-gamma increases during viral infection, and PCT synthesis is inhibited by IFN-gamma [Citation131]. The inhibitory effect of IFN-gamma on IL-1beta-induced PCT secretion may explain why the rise in the serum PCT concentration in viral infections is lower than in bacterial infection [Citation121]. Studies have also shown that the lack of change in PCT levels in viral infections may be caused by the virus stimulating macrophages to produce IFN-gamma and inhibiting the release of TNF-alpha in the immune response [Citation59,Citation132].
Because serum PCT concentrations are basically unchanged during viral infection and are significantly increased during bacterial infection, they are used mostly for the differential diagnosis of bacterial coinfection in patients with viral infections, and most of the relevant studies have been on patients with respiratory viral infections. For example, the cutoff value for differentiating pneumonia caused by mixed bacterial and 2009 H1N1 influenza infection from influenza infection alone was 1.5 ng/mL (AUC = 0.698, 95%CI 52.3–87.3, sensitivity 56%, specificity 84%) [Citation44]. Other studies have reported different cutoff values for this purpose. Pfister et al. confirmed that a cutoff value of 0.5 ng/mL could diagnose bacterial pneumonia (AUC = 0.72, 95%CI 64–80, sensitivity 80.5%, NPV 73.2%) [Citation43]. A multicenter study found that a PCT value of ≥ 0.8 ng/mL combined with clinical judgment was strongly associated with bacterial coinfection (AUC = 0.90, 95%CI 78–100, sensitivity 91%, specificity 68%) [Citation45]. Another study showed that the PCT cutoff value to exclude community acquired respiratory coinfection in patients with viral pneumonia caused by influenza A (H1N1) was 0.29 ng/mL (AUC = 0.716, 95%CI 67–75, sensitivity 88.2%, specificity 33.2%, PPV 25.0%, NPV 91.8%) [Citation42].
A study showed that the optimal cutoff value of PCT for children with severe RSV pneumonia and with severe RSV pneumonia combined with bacterial infection was >2.25 ng/mL (AUC = 0.749, 95%CI 57.1–92.7, sensitivity 55%, specificity 92%, PPV 55%, NPV 92%) [Citation46]. Another recent study demonstrated that PCT could also increase during simple viral infection, and in proportion to the severity of the disease, and was not inhibited by IFN signals [Citation87]; it also pointed out that PCT was more suitable to assess the severity of the disease than to differentially diagnose the presence of bacterial coinfections in patients with viral infections [Citation87].
Studies have shown that the concentration of PCT at admission could predict disease severity, secondary bacterial infection and mortality of patients with hemorrhagic fever with renal syndrome caused by Hantaan virus. One study showed that the PCT level in severely ill patients (n = 72) was significantly higher than in mildly ill patients (n = 74, p < 0.001), and was independently related to disease severity (odds ratio 2.544, 95% CI 1.330–4.868, p = 0.005) [Citation133].
Mycoplasma, Chlamydia, Spirochetes and Rickettsia
At present, research is lacking on the relationship between PCT and Chlamydia and Spirochetes. Research on Mycoplasma and Rickettsia has focused mainly on evaluating the value of PCT and the diagnostic value of the combined detection by PCT and CRP. A decrease in PCT (especially PCT < 0.25 ng/mL) was statistically related to MP infection, and the combination of age > 5 years (AUC = 0.77), pre-fever and respiratory symptoms > 6 days (AUC = 0.79), PCT < 0.25 ng/mL (AUC = 0.81) could improve the diagnostic performance of high-risk patients with MP-CAP (AUC = 0.90, p = 0.05) [Citation50]. Two recent studies pointed out that the CRP/PCT ratio may be a potential indicator for distinguishing between MP-CAP and non-MP-CAP [Citation49,Citation51]. Olivia et al. believed that CRP/PCT >400 μg/ng helped to predict MP-CAP in adults [Citation51], while Li et al. found that CRP/PCT >350 μg/ng showed excellent diagnostic performance (AUC = 0.90, 95%CI 83–95, p < 0.001, sensitivity 76%, specificity 100%) [Citation49], so the CRP/PCT ratio may be a potential indicator to distinguish MP-CAP from non-MP-CAP. In terms of Rickettsia, a report on tsutsugamushi disease caused by Orientia tsutsugamushi infection gave the optimal cutoff value of 1.3 ng/mL (sensitivity 87.7%, specificity 78.0%) for the identification of acute tsutsugamushi, indicating that serum PCT (≤1.3 ng/mL) could be used as an auxiliary test for the diagnosis of acute tsutsugamushi [Citation52].
Parasites
Research on PCT in parasites has focused on Plasmodium. Plasmodium falciparum malaria is a disease with obvious heterogeneity, and its prognosis varies from complete recovery to death [Citation53]. In adult patients with acute Plasmodium falciparum malaria, the plasma PCT level increases. Moreover, the PCT level of patients with severe and non-severe acute disease was significantly increased, and the degree of increase was positively correlated with disease severity markers [Citation134]. Studies have shown that PCT can be used to measure disease severity and mortality risk of patients with Plasmodium falciparum malaria. PCT >25 ng/mL indicated a very high risk of death [Citation53]. A study pointed out that the results of PCT determination at admission were better than CRP, platelet count and bilirubinemia, and could be used for preliminary clinical evaluation of the severity of disease in travelers in non-endemic areas [Citation135]. Compared with other parameters, PCT >5 ng/mL had the strongest association (highest specificity 86%, highest positive predictive factor 67%, high sensitivity 67%) with complex malaria. Only PCT was associated with an increased risk of complicated malaria (odds ratio 8.2, 95%CI 1.2–57.2, p = 0.03) [Citation135]. In addition, broad applicability to various populations makes PCT a useful tool for assessing children with severe malaria and tracking clinical progress, even in non-specialist hospitals in areas where malaria is not endemic [Citation136]. In fact, identifying patients with severe malaria with Plasmodium falciparum in non-malaria endemic areas is a key factor for the best management of these patients, and PCT has diagnostic value in this regard [Citation136].
Discussion
PCT has been extensively and thoroughly studied in the rapid diagnosis of clinical diseases, condition monitoring and evaluation, and guiding medical decision-making.
However, the current coverage of PCT research is not broad enough, although research in 2020 did involve children and patients with malignant tumors [Citation4,Citation13]. Future research objects should include the clinical application of PCT, an important clinical biomarker, in more populations, for example, infants, children, adolescents, adults, the elderly, pregnant women, intensive care patients, immunodeficiency patients, transplant recipients, cancer patients and other groups to evaluate the clinical value of PCT comprehensively. As well, research should be conducted in non-ICU patients to determine if PCT-guided antibiotic therapy is safe and effective for all types of suspected or confirmed infections, and to assess if it is useful in patients on anti-inflammatory and other drugs.
Optimal PCT cutoffs, based on different research methods, for diagnosing a given disease differ. Thus, it would be helpful if research approaches were less heterogeneous, in order to standardize the specific cutoff value of each disease. We believe that such practice would significantly improve the diagnostic efficacy of PCT.
Current research on PCT as a biomarker has mainly compared the utility of PCT with CRP, IL-6, WBC and other indicators of infection. We suggest that further research is needed to explore the diagnostic efficacy of combination of indicators to improve the utility of PCT.
Recent research has focused on explaining the relationship between PCT and infectious diseases caused by different pathogen. However, research on the mechanisms of changes in PCT levels is still lacking, and some studies have pointed out that it is related to signal transduction. It was reported that MyD88, which encodes a cytosolic adapter protein that plays a central role in the innate and adaptive immune response, is not the main reason for the difference in the expression of inflammatory factors induced by S. aureus, GAS, and E. coli [Citation56]. There are other adaptor proteins involved in regulating the expression of the inflammatory factors, and their role in regulating serum PCT levels needs to be explored.
Our review has demonstrated the associations between PCT and pathogenic microorganisms and clinical conditions. In the future, knowledge of the PCT range for each common pathogen could help to guiding the use of empirical clinical medications and the appropriate employment of antibiotics.
Author contributions
All authors contributed to the draft and have seen and approved the final version of the report.
Abbreviations | ||
AUC | = | area under the curve |
BSI | = | bloodstream infection |
CALC-I | = | calcitonin I |
CAP | = | community-acquired pneumonia |
CI | = | confidence interval |
CLR | = | C-type lectin receptor |
CoNS | = | coagulase-negative Staphylococcus |
CRP | = | C-reactive protein |
G– | = | Gram-negative |
G+ | = | Gram-positive |
GAS | = | Group A Streptococcus |
ICU | = | intensive care unit |
IE | = | infective endocarditis |
IL | = | interleukin |
IFN | = | interferon |
LPS | = | lipopolysaccharide |
LTA | = | lipoteichoic acid |
MAPK | = | mitogen-activated protein kinase |
mCD14 | = | membrane-bound CD14 |
MD-2 | = | myeloid differentiation factor-2 |
MP | = | Mycoplasma pneumoniae |
MyD88 | = | myeloid differentiation factor 88 |
NF-κB | = | NF-kappaB |
NPV | = | negative predictive value |
PAMPs | = | pathogen-associated molecular patterns |
PCT | = | procalcitonin |
PPV | = | positive predictive value |
RSV | = | respiratory syncytial virus |
SIRS | = | systemic inflammatory response syndrome |
TLR | = | Toll-like receptor |
TNF | = | tumor necrosis factor |
Disclosure statement
No potential conflict of interest was reported by the author(s).
Additional information
Funding
References
- Aloisio E, Dolci A, Panteghini M. Procalcitonin: between evidence and critical issues. Clin Chim Acta. 2019;496:7–12.
- Le Moullec JM, Jullienne A, Chenais J, et al. The complete sequence of human preprocalcitonin. FEBS Lett. 1984;167(1):93–97.
- Cleland DA, Eranki AP. Procalcitonin. In: StatPearls [internet]. Treasure Island (FL): StatPearls Publishing; 2021 [cited 2021 Aug 11]. Available from: https://www.ncbi.nlm.nih.gov/books/NBK539794
- Downes KJ, Fitzgerald JC, Weiss SL. Utility of procalcitonin as a biomarker for sepsis in children. J Clin Microbiol. 2020;58(7):e01851–19.
- Ponti G, Maccaferri M, Ruini C, et al. Biomarkers associated with COVID-19 disease progression. Crit Rev Clin Lab Sci. 2020;57(6):389–399.
- Gai L, Tong Y, Yan BQ. Research on the diagnostic effect of PCT level in serum on patients with sepsis due to different pathogenic causes. Eur Rev Med Pharmacol Sci. 2018;22:4238–4242.
- Müller B, White JC, Nylén ES, et al. Ubiquitous expression of the calcitonin-i gene in multiple tissues in response to sepsis. J Clin Endocrinol Metab. 2001;86(1):396–404.
- Rau B, Steinbach G, Gansauge F, et al. The potential role of procalcitonin and interleukin 8 in the prediction of infected necrosis in acute pancreatitis. Gut. 1997;41(6):832–840.
- Oczenski W, Fitzgerald RD, Schwarz S. Procalcitonin: a new parameter for the diagnosis of bacterial infection in the peri-operative period. Eur J Anaesthesiol. 1998;15(2):202–209.
- Kim YJ, Kang SW, Lee JH, et al. Marked elevation of procalcitonin level can lead to a misdiagnosis of anaphylactic shock as septic shock. Int J Infect Dis. 2015;37:93–94.
- Rhee C. Using Procalcitonin to guide antibiotic therapy. Open Forum Infect Dis. 2016;4:ofw249.
- Assicot M, Gendrel D, Carsin H, et al. High serum procalcitonin concentrations in patients with sepsis and infection. Lancet. 1993;341(8844):515–518.
- Shokripour M, Omidifar N, Salami K, et al. Diagnostic accuracy of immunologic biomarkers for accurate diagnosis of bloodstream infection in patients with malignancy: Procalcitonin in comparison with C-reactive protein. Can J Infect Dis Med Microbiol. 2020;2020:8362109.
- El Haddad H, Chaftari AM, Hachem R, et al. Biomarkers of sepsis and bloodstream infections: the role of procalcitonin and proadrenomedullin with emphasis in patients with cancer. Clin Infect Dis. 2018;67(6):971–977.
- Watkin RW, Harper LV, Vernallis AB, et al. Pro-inflammatory cytokines IL6, TNF-alpha, IL1beta, procalcitonin, lipopolysaccharide binding protein and C-reactive protein in infective endocarditis. J Infect. 2007;55(3):220–225.
- Lin JC, Chen ZH, Chen XD. Elevated serum procalcitonin predicts Gram-negative bloodstream infections in patients with burns. Burns. 2020;46(1):182–189.
- Nakae H, Inaba H, Endo S. Usefulness of procalcitonin in Pseudomonas burn wound sepsis model. Tohoku J Exp Med. 1999;188(3):271–273.
- Becker KL, Nylén ES, White JC, et al. Clinical review 167: Procalcitonin and the calcitonin gene family of peptides in inflammation, infection, and sepsis: a journey from calcitonin back to its precursors. J Clin Endocrinol Metab. 2004;89(4):1512–1525.
- Lippi G, Sanchis-Gomar F. Procalcitonin in inflammatory bowel disease: drawbacks and opportunities. World J Gastroenterol. 2017;23(47):8283–8290.
- Schuetz P, Albrich W, Mueller B. Procalcitonin for diagnosis of infection and guide to antibiotic decisions: past, present and future. BMC Med. 2011;9:107. https://doi.org/https://doi.org/10.1186/1741-7015-9-107
- Simon L, Gauvin F, Amre DK, et al. Serum procalcitonin and C-reactive protein levels as markers of bacterial infection: a systematic review and meta-analysis. Clin Infect Dis. 2004;39(2):206–217.
- Wu JY, Lee SH, Shen CJ, et al. Use of serum procalcitonin to detect bacterial infection in patients with autoimmune diseases: a systematic review and Meta-analysis. Arthritis Rheum. 2012;64(9):3034–3042.
- Liu HH, Guo JB, Geng Y, et al. Procalcitonin: present and future. Ir J Med Sci. 2015;184(3):597–605.
- Tang JH, Gao DP, Zou PF. Comparison of serum PCT and CRP levels in patients infected by different pathogenic microorganisms: a systematic review and Meta-analysis. Braz J Med Biol Res. 2018;51(7):e6783.
- Shomali W, Hachem R, Chaftari AM, et al. Can procalcitonin differentiate Staphylococcus aureus from coagulase-negative staphylococci in clustered gram-positive bacteremia? Diagn Microbiol Infect Dis. 2013;76(2):158–161.
- Tascini C, Aimo A, Arzilli C, et al. Procalcitonin, white blood cell count and C-reactive protein as predictors of S. aureus infection and mortality in infective endocarditis. Int J Cardiol. 2020;301:190–194.
- Giamarellou H, Giamarellos-Bourboulis EJ, Repoussis P, et al. Potential use of procalcitonin as a diagnostic criterion in febrile neutropenia: experience from a multicentre study. Clin Microbiol Infect. 2004;10(7):628–633.
- Schuetz P, Mueller B, Trampuz A. Serum procalcitonin for discrimination of blood contamination from bloodstream infection due to coagulase-negative staphylococci. Infection. 2007;35(5):352–355.
- Rast AC, Knobel D, Faessler L, et al. Use of procalcitonin, C-reactive protein and white blood cell count to distinguish between lower limb erysipelas and deep vein thrombosis in the emergency department: a prospective observational study. J Dermatol. 2015;42(8):778–785.
- Charles PE, Ladoire S, Aho S, et al. Serum procalcitonin elevation in critically ill patients at the onset of bacteremia caused by either Gram negative or Gram positive bacteria. BMC Infect Dis. 2008;8:38.
- Leli C, Ferranti M, Moretti A, et al. Procalcitonin levels in gram-positive, gram-negative, and fungal bloodstream infections. Dis Markers. 2015;2015:1–8.
- Fu Y, Chen J, Cai B, et al. The use of PCT, CRP, IL-6 and SAA in critically ill patients for an early distinction between candidemia and Gram positive/negative bacteremia. J Infect. 2012;64(4):438–440.
- Li S, Rong H, Guo Q, et al. Serum procalcitonin levels distinguish Gram-negative bacterial sepsis from Gram-positive bacterial and fungal sepsis. J Res Med Sci. 2016;21(1):39.
- Luo X, Chen S, Zhang J, et al. Procalcitonin as a marker of Gram-negative bloodstream infections in hematological patients with febrile neutropenia. Leuk Lymphoma. 2019;60(10):2441–2448.
- Yan ST, Sun LC, Jia HB, et al. Procalcitonin levels in bloodstream infections caused by different sources and species of bacteria. Am J Emerg Med. 2017;35(4):579–583.
- Leng Y, Chen C, Zhang Y, et al. Ability of serum procalcitonin to distinguish focus of infection and pathogen types in patients with bloodstream infection. Ann Transl Med. 2019;7(7):135–135.
- Cabral L, Afreixo V, Meireles R, et al. Evaluation of procalcitonin accuracy for the distinction between Gram-negative and Gram-positive bacterial sepsis in burn Patients. J Burn Care Res. 2019;40(1):112–119.
- Pieralli F, Corbo L, Torrigiani A, et al. Usefulness of procalcitonin in differentiating Candida and bacterial blood stream infections in critically ill septic patients outside the intensive care unit. Intern Emerg Med. 2017;12(5):629–635.
- Martini A, Gottin L, Menestrina N, et al. Procalcitonin levels in surgical patients at risk of candidemia. J Infect. 2010;60(6):425–430.
- Charles PE, Dalle F, Aho S, et al. Serum procalcitonin measurement contribution to the early diagnosis of candidemia in critically ill patients. Intensive Care Med. 2006;32(10):1577–1583.
- Cortegiani A, Russotto V, Montalto F, et al. Procalcitonin as a marker of Candida species detection by blood culture and polymerase chain reaction in septic patients. BMC Anesthesiol. 2014;14:9.
- Rodríguez AH, Avilés-Jurado FX, Díaz E, et al. Procalcitonin (PCT) levels for ruling-out bacterial coinfection in ICU patients with influenza: a CHAID decision-tree analysis. J Infect. 2016;72:143–151.
- Pfister R, Kochanek M, Leygeber T, et al. Procalcitonin for diagnosis of bacterial pneumonia in critically ill patients during 2009 H1N1 influenza pandemic: a prospective cohort study, systematic review and individual patient data Meta-analysis. Crit Care. 2014;18(2):R44.
- Ahn S, Kim WY, Kim SH, et al. Role of procalcitonin and C-reactive protein in differentiation of mixed bacterial infection from 2009 H1N1 viral pneumonia. Influenza Other Respir Viruses. 2011;5(6):398–403.
- Cuquemelle E, Soulis F, Villers D, et al. Can procalcitonin help identify associated bacterial infection in patients with severe influenza pneumonia? A multicentre study. Intensive Care Med. 2011;37(5):796–800.
- Do Q, Dao TM, Nguyen TNT, et al. Procalcitonin identifies bacterial coinfections in vietnamese children with severe respiratory syncytial virus pneumonia. Biomed Res Int. 2020;2020:7915158.
- Yang M, Gao H, Chen J, et al. Bacterial coinfection is associated with severity of avian influenza A (H7N9), and procalcitonin is a useful marker for early diagnosis. Diagn Microbiol Infect Dis. 2016;84(2):165–169.
- Laham JL, Breheny PJ, Gardner BM, et al. Procalcitonin to predict bacterial coinfection in infants with acute bronchiolitis: a preliminary analysis. Pediatr Emerg Care. 2014;30(1):11–15.
- Li F, Kong S, Xie K, et al. High ratio of C-reactive protein/procalcitonin predicts Mycoplasma pneumoniae infection among adults hospitalized with community acquired pneumonia. Scand J Clin Lab Invest. 2021;81(1):65–71.
- Meyer Sauteur PM, Krautter S, Ambroggio L, et al. Improved diagnostics help to identify clinical features and biomarkers that predict Mycoplasma pneumoniae community-acquired pneumonia in children. Clin Infect Dis. 2020;71(7):1645–1654.
- Neeser OL, Vukajlovic T, Felder L, et al. A high C-reactive protein/procalcitonin ratio predicts Mycoplasma pneumoniae infection. Clin Chem Lab Med. 2019;57(10):1638–1646.
- Lee CS, Hwang JH, Lee JM, et al. The clinical usefulness of serum procalcitonin level in patients with scrub typhus. Korean J Intern Med. 2017;32(4):761–763.
- Chiwakata CB, Manegold C, Bönicke L, et al. Procalcitonin as a parameter of disease severity and risk of mortality in patients with Plasmodium falciparum malaria. J Infect Dis. 2001;183(7):1161–1164.
- Shomali W, Hachem R, Chaftari AM, et al. Can procalcitonin distinguish infectious fever from tumor-related fever in non-neutropenic cancer patients? Cancer. 2012;118(23):5823–5829.
- Sato A, Kaido T, Iida T, et al. Bundled strategies against infection after liver transplantation: lessons from multidrug-resistant Pseudomonas aeruginosa. Liver Transpl. 2016;22(4):436–445.
- Wu S, Ma C, Gao X, et al. Group a Streptococcus induces less p65 nuclear translocation and non-classical nuclear factor kappa B activation in macrophages, which possibly leads to a weaker inflammatory response. Int J Infect Dis. 2016;44:50–60.
- Leli C, Ferranti M, Marrano U, et al. Diagnostic accuracy of presepsin (sCD14-ST) and procalcitonin for prediction of bacteraemia and bacterial DNAaemia in patients with suspected sepsis. J Med Microbiol. 2016;65(8):713–719.
- Sivapalan P, Jensen JU. Procalcitonin in acute infections: from the research laboratory to clinical impact-new perspectives of biomarker use. J Lab Precis Med. 2019;4:36–36.
- Prucha M, Bellingan G, Zazula R. Sepsis biomarkers. Clin Chim Acta. 2015;440:97–103.
- Wu CC, Lan HM, Han ST, et al. Comparison of diagnostic accuracy in sepsis between presepsin, procalcitonin, and C-reactive protein: a systematic review and Meta-analysis. Ann Intensive Care. 2017;7:91.
- Tan M, Lu Y, Jiang H, et al. The diagnostic accuracy of procalcitonin and C-reactive protein for sepsis: a systematic review and meta-analysis. J Cell Biochem. 2019;120(4):5852–5859.
- Wacker C, Prkno A, Brunkhorst FM, et al. Procalcitonin as a diagnostic marker for sepsis: a systematic review and meta-analysis. Lancet Infect Dis. 2013;13(5):426–435.
- Cong S, Ma T, Di X, et al. Diagnostic value of neutrophil CD64, procalcitonin, and interleukin-6 in sepsis: a meta-analysis. BMC Infect Dis. 2021;21(1):384.
- Uzzan B, Cohen R, Nicolas P, et al. Procalcitonin as a diagnostic test for sepsis in critically ill adults and after surgery or trauma: a systematic review and meta-analysis. Crit Care Med. 2006;34(7):1996–2003.
- Travaglino F, De Berardinis B, Magrini L, et al. Utility of procalcitonin (PCT) and mid regional pro-adrenomedullin (MR-proADM) in risk stratification of critically ill febrile patients in emergency department (ED). a comparison with APACHE II score. BMC Infect Dis. 2012;12:184.
- Huang DT, Weissfeld LA, Kellum JA, et al. Risk prediction with procalcitonin and clinical rules in community-acquired pneumonia. Ann Emerg Med. 2008;52(1):48–58.
- Julián-Jiménez A, Iqbal-Mirza SZ, de Rafael González E, et al. Predicting bacteremia in patients attended for infections in an emergency department: the 5MPB-Toledo model. Emergencias. 2020;32:81–89.
- Michaelidis CI, Zimmerman RK, Nowalk MP, et al. Cost-effectiveness of procalcitonin-guided antibiotic therapy for outpatient management of acute respiratory tract infections in adults. J Gen Intern Med. 2014;29(4):579–586.
- Pérez-López A, Irwin A, Rodrigo C, et al. Role of C reactive protein and procalcitonin in the diagnosis of lower respiratory tract infection in children in the outpatient setting. BMJ. 2021;373:n1409.
- Gómez-Zorrilla S, Morandeira F, Castro MJ, et al. Acute inflammatory response of patients with Pseudomonas aeruginosa infections: a prospective study. Microb Drug Resist. 2017;23(4):523–530.
- Reyes Mondragón AL, Cantú-Rodríguez OG, Garza-Acosta AC, et al. Performance of serum procalcitonin as a biochemical predictor of death in hematology patients with febrile neutropenia. Blood Cells Mol Dis. 2021;90:102586. https://doi.org/https://doi.org/10.1016/j.bcmd.2021.102586
- Becker KL, Snider R, Nylen ES. Procalcitonin assay in systemic inflammation, infection, and sepsis: clinical utility and limitations. Crit Care Med. 2008;36(3):941–952.
- Hatherill M, Tibby SM, Turner C, et al. Procalcitonin and cytokine levels: relationship to organ failure and mortality in pediatric septic shock. Crit Care Med. 2000;28(7):2591–2594.
- Vujaklija Brajković A, Košuta I, Tomek D, et al. Utility of procalcitonin in a medical intensive care unit in Croatia. Wien Klin Wochenschr. 2021;133(15–16):832–838.
- Nobre V, Harbarth S, Graf JD, et al. Use of procalcitonin to shorten antibiotic treatment duration in septic patients: a randomized trial. Am J Respir Crit Care Med. 2008;177(5):498–505.
- Schuetz P, Wirz Y, Sager R, et al. Procalcitonin to initiate or discontinue antibiotics in acute respiratory tract infections. Cochrane Database Syst Rev. 2017;(10):CD007498.
- de Jong E, van Oers JA, Beishuizen A, et al. Efficacy and safety of procalcitonin guidance in reducing the duration of antibiotic treatment in critically ill patients: a randomised, controlled, open-label trial. Lancet Infect Dis. 2016;16(7):819–827.
- Bloos F, Trips E, Nierhaus A, et al. Effect of sodium selenite administration and procalcitonin-guided therapy on mortality in patients with severe sepsis or septic shock: a randomized clinical trial. JAMA Intern Med. 2016;176(9):1266–1276.
- Cortegiani A, Misseri G, Ippolito M, et al. Procalcitonin levels in candidemia versus bacteremia: a systematic review. Crit Care. 2019;23(1):190.
- Stojanovic I, Schneider JE, Wei L, et al. Economic evaluation of procalcitonin-guided antibiotic therapy in acute respiratory infections: a Chinese hospital system perspective. Clin Chem Lab Med. 2017;55(4):561–570.
- Schuetz P, Balk R, Briel M, et al. Economic evaluation of procalcitonin-guided antibiotic therapy in acute respiratory infections: a US health system perspective. Clin Chem Lab Med. 2015;53(4):583–592.
- Dandona P, Nix D, Wilson MF, et al. Procalcitonin increase after endotoxin injection in normal subjects. J Clin Endocrinol Metab. 1994;79:1605–1608.
- Thomas-Rüddel DO, Poidinger B, Kott M, et al. Influence of pathogen and focus of infection on procalcitonin values in sepsis patients with bacteremia or candidemia. Crit Care. 2018;22(1):128.
- Stolz D, Smyrnios N, Eggimann P, et al. Procalcitonin for reduced antibiotic exposure in ventilator-associated pneumonia: a randomised study. Eur Respir J. 2009;34(6):1364–1375.
- Shehabi Y, Sterba M, Garrett PM, et al. Procalcitonin algorithm in critically ill adults with undifferentiated infection or suspected sepsis: a randomized controlled trial. Am J Respir Crit Care Med. 2014;190(10):1102–1110.
- Matwiyoff GN, Prahl JD, Miller RJ, et al. Immune regulation of procalcitonin: a biomarker and mediator of infection. Inflamm Res. 2012;61(5):401–409.
- Gautam S, Cohen AJ, Stahl Y, et al. Severe respiratory viral infection induces procalcitonin in the absence of bacterial pneumonia. Thorax. 2020;75(11):974–981.
- Abe R, Oda S, Sadahiro T, et al. Gram-negative bacteremia induces greater magnitude of inflammatory response than Gram-positive bacteremia. Crit Care. 2010;14(2):R27.
- Menéndez R, Sahuquillo-Arce JM, Reyes S, et al. Cytokine activation patterns and biomarkers are influenced by microorganisms in community-acquired pneumonia. Chest. 2012;141(6):1537–1545.
- Kumar S, Ingle H, Prasad DV, et al. Recognition of bacterial infection by innate immune sensors. Crit Rev Microbiol. 2013;39(3):229–246.
- Gu M, Xu HG. Association of procalcitonin concentrations with pathogenic microorganisms. Clin Chem. 2020;66(10):1353–1356.
- Feezor RJ, Oberholzer C, Baker HV, et al. Molecular characterization of the acute inflammatory response to infections with gram-negative versus gram-positive bacteria. Infect Immun. 2003;71(10):5803–5813.
- Gao H, Evans TW, Finney SJ. Bench-to-bedside review: sepsis, severe sepsis and septic shock – does the nature of the infecting organism matter? Crit Care. 2008;12(3):213.
- Re F, Strominger JL. Toll-like receptor 2 (TLR2) and TLR4 differentially activate human dendritic cells. J Biol Chem. 2001;276(40):37692–37699.
- Tong SY, Davis JS, Eichenberger E, et al. Staphylococcus aureus infections: epidemiology, pathophysiology, clinical manifestations, and management. Clin Microbiol Rev. 2015;28(3):603–661.
- DeLeo FR, Diep BA, Otto M. Host defense and pathogenesis in Staphylococcus aureus infections. Infect Dis Clin North Am. 2009;23(1):17–34.
- Balog A, Ocsovszki I, Mándi Y. Flow cytometric analysis of procalcitonin expression in human monocytes and granulocytes. Immunol Lett. 2002;84(3):199–203.
- Hui-Heng L, Jun-Sheng W, Jin-Zhou L, et al. LPS induced PCT production via TLR-4/NF-кB passway:it is the difference of G–/G+ bacteremia rats. Cytokine. 2021;137:155317.
- Spyropoulos V, Chalkias A, Georgiou G, et al. Initial immune response in Escherichia coli, Staphylococcus aureus, and Candida albicans bacteremia. Inflammation. 2020;43(1):179–190.
- Cuculi F, Toggweiler S, Auer M, et al. Serum procalcitonin has the potential to identify Staphylococcus aureus endocarditis. Eur J Clin Microbiol Infect Dis. 2008;27(11):1145–1149.
- Jereb M, Kotar T, Jurca T, et al. Usefulness of procalcitonin for diagnosis of infective endocarditis. Intern Emerg Med. 2009;4(3):221–226.
- Cornelissen CG, Frechen DA, Schreiner K, et al. Inflammatory parameters and prediction of prognosis in infective endocarditis. BMC Infect Dis. 2013;13:272.
- Argemi X, Hansmann Y, Prola K, et al. Coagulase-negative Staphylococci pathogenomics. Int J Mol Sci. 2019;20(5):1215.
- Becker K, Heilmann C, Peters G. Coagulase-negative staphylococci. Clin Microbiol Rev. 2014;27(4):870–926.
- Kordek A. Concentrations of procalcitonin and C-reactive protein, white blood cell count, and the immature-to-total neutrophil ratio in the blood of neonates with nosocomial infections: Gram-negative bacilli vs coagulase-negative staphylococci. Eur J Clin Microbiol Infect Dis. 2011;30(3):455–457.
- Białecka A, Mak M, Biedroń R, et al. Different pro-inflammatory and immunogenic potentials of Propionibacterium acnes and Staphylococcus epidermidis: implications for chronic inflammatory acne. Arch Immunol Ther Exp. 2005;53(1):79–85.
- Rewa O, Muscedere J, Reynolds S, et al. Coagulase-negative Staphylococcus, catheter-related, bloodstream infections and their association with acute phase markers of inflammation in the intensive care unit: an observational study. Can J Infect Dis Med Microbiol. 2012;23(4):204–208.
- Verboon-Maciolek MA, Thijsen SF, Hemels MA, et al. Inflammatory mediators for the diagnosis and treatment of sepsis in early infancy. Pediatr Res. 2006;59(3):457–461.
- Chiesa C, Pacifico L, Rossi N, et al. Procalcitonin as a marker of nosocomial infections in the neonatal intensive care unit. Intensive Care Med. 2000;26(S2):S175–S177.
- Engholm DH, Kilian M, Goodsell DS, et al. A visual review of the human pathogen Streptococcus pneumoniae. FEMS Microbiol Rev. 2017;41(6):854–879.
- Malley R, Henneke P, Morse SC, et al. Recognition of pneumolysin by Toll-like receptor 4 confers resistance to pneumococcal infection. Proc Natl Acad Sci USA. 2003;100(4):1966–1971.
- Lopardo G, Basombrío A, Clara L, et al. Neumonía adquirida de la comunidad en adultos. Recomendaciones sobre su atención [Guidelines for management of community-acquired pneumonia in adults]. Medicina. 2015;75:245–257.
- Hedlund J, Hansson LO. Procalcitonin and C-reactive protein levels in community-acquired pneumonia: correlation with etiology and prognosis. Infection. 2000;28(2):68–73.
- Bellmann-Weiler R, Ausserwinkler M, Kurz K, et al. Clinical potential of C-reactive protein and procalcitonin serum concentrations to guide differential diagnosis and clinical management of pneumococcal and Legionella pneumonia. J Clin Microbiol. 2010;48(5):1915–1917.
- McGill F, Heyderman RS, Panagiotou S, et al. Acute bacterial meningitis in adults. Lancet. 2016;388(10063):3036–3047.
- Henry BM, Roy J, Ramakrishnan PK, et al. Procalcitonin as a serum biomarker for differentiation of bacterial meningitis from viral meningitis in children: evidence from a Meta-Analysis. Clin Pediatr. 2016;55(8):749–764.
- Kim J, Kim SE, Park BS, et al. Procalcitonin as a diagnostic and prognostic factor for tuberculosis meningitis. J Clin Neurol. 2016;12(3):332–339.
- Oberhoffer M, Stonans I, Russwurm S, et al. Procalcitonin expression in human peripheral blood mononuclear cells and its modulation by lipopolysaccharides and sepsis-related cytokines in vitro. J Lab Clin Med. 1999;134(1):49–55.
- Li M, Yang F, Lu Y, et al. Identification of Enterococcus faecalis in a patient with urinary-tract infection based on metagenomic next-generation sequencing: a case report. BMC Infect Dis. 2020;20(1):467.
- Croxen MA, Law RJ, Scholz R, et al. Recent advances in understanding enteric pathogenic Escherichia coli. Clin Microbiol Rev. 2013;26(4):822–880.
- Linscheid P, Seboek D, Nylen ES, et al. In vitro and in vivo calcitonin I gene expression in parenchymal cells: a novel product of human adipose tissue. Endocrinology. 2003;144(12):5578–5584.
- Elson G, Dunn-Siegrist I, Daubeuf B, et al. Contribution of toll-like receptors to the innate immune response to Gram-negative and Gram-positive bacteria. Blood. 2007;109(4):1574–1583.
- Ogasawara S, Saito N, Hirano R, et al. Clinical relevance of procalcitonin values in bacteremia. J Infect Chemother. 2020;26(10):1048–1053.
- Giamarellos-Bourboulis EJ, Plachouras D, Tzivra A, et al. Stimulation of innate immunity by susceptible and multidrug-resistant Pseudomonas aeruginosa: an in vitro and in vivo study. Clin Exp Immunol. 2004;135(2):240–246.
- Contou D, d’Ythurbide G, Messika J, et al. Description and predictive factors of infection in patients with chronic kidney disease admitted to the critical care unit. J Infect. 2014;68(2):105–115.
- Hu C, Jiang J, Li Z, et al. Expression pattern of soluble triggering receptor expressed on myeloid cells-1 in mice with Acinetobacter baumannii colonization and infection in the lung. J Thorac Dis. 2018;10(3):1614–1621.
- Zaki MES, Abed S, Elegezy M, et al. Can triggering receptors expressed on myeloid cells be used for diagnosis of Salmonella typhi infection? Endocr Metab Immune Disord Drug Targets. 2020;20(9):1488–1493.
- Plato A, Hardison SE, Brown GD. Pattern recognition receptors in antifungal immunity. Semin Immunopathol. 2015;37(2):97–106.
- Sood BG, Shankaran S, Schelonka RL, et al. Cytokine profiles of preterm neonates with fungal and bacterial sepsis. Pediatr Res. 2012;72(2):212–220.
- Brodská H, Malíčková K, Adámková V, et al. Significantly higher procalcitonin levels could differentiate Gram-negative sepsis from Gram-positive and fungal sepsis. Clin Exp Med. 2013;13(3):165–170.
- Lippi G, Plebani M. Procalcitonin in patients with severe coronavirus disease 2019 (COVID-19): a meta-analysis. Clin Chim Acta. 2020;505:190–191.
- Han J, Gatheral T, Williams C. Procalcitonin for patient stratification and identification of bacterial co-infection in COVID-19. Clin Med. 2020;20(3):e47.
- Fan X, Deng H, Sang J, et al. High serum procalcitonin concentrations in patients with hemorrhagic fever with renal syndrome caused by hantaan virus. Front Cell Infect Microbiol. 2018;8:129.
- Braun N, Marfo Y, Von Gärtner C, et al. CTLA-4 positive T cells in contrast to procalcitonin plasma levels discriminate between severe and uncomplicated Plasmodium falciparum malaria in Ghanaian children. Trop Med Int Health. 2003;8(11):1018–1024.
- Righi E, Merelli M, Arzese A, et al. Determination of PCT on admission is a useful tool for the assessment of disease severity in travelers with imported Plasmodium falciparum malaria. Acta Parasitol. 2016;61:412–418.
- Carannante N, Rossi M, Fraganza F, et al. A high PCT level correlates with disease severity in Plasmodium falciparum malaria in children. New Microbiol. 2017;40(1):72–74.