Abstract
Inositols, a group of 6-carbon polyols, are highly bioactive molecules derived from diet and endogenous synthesis. Inositols and their derivatives are involved in glucose and lipid metabolism and participate in insulin-signaling, with perturbations in inositol processing being associated with conditions involving insulin resistance, dysglycemia and dyslipidemia such as polycystic ovary syndrome and diabetes. Pregnancy is similarly characterized by substantial and complex changes in glycemic and lipidomic regulation as part of maternal adaptation and is also associated with physiological alterations in inositol processing. Disruptions in maternal adaptation are postulated to have a critical pathophysiological role in pregnancy complications such as gestational diabetes and pre-eclampsia. Inositol supplementation has shown promise as an intervention for the alleviation of symptoms in conditions of insulin resistance and for gestational diabetes prevention. However, the mechanisms behind these affects are not fully understood. In this review, we explore the role of inositols in conditions of insulin dysregulation and in pregnancy, and identify priority areas for research. We particularly examine the role and function of inositols within the maternal-placental-fetal axis in both uncomplicated and pathological pregnancies. We also discuss how inositols may mediate maternal-placental-fetal cross-talk, and regulate fetal growth and development, and suggest that inositols play a vital role in promoting healthy pregnancy.
Introduction
Inositols are 6-carbon polyols present in all living cells (Noventa et al. Citation2016). Humans synthesize approximately 4 g of inositol a day in the kidney (Clements 1979), but inositols are also abundant in fruits, grains and nuts, with a typical North American diet providing about 1 g of inositol a day (Holub Citation1986). Inositol and inositol derivatives often act as both insulin mimics and second messengers (Asplin, Galasko, and Larner Citation1993; Greene et al. Citation1987; Huang et al. Citation1993; Suzuki et al. Citation1994; Larner, Brautigan, and Thorner Citation2010; Cheang et al. Citation2008; Unfer et al. Citation2012; Facchinetti et al. Citation2015; Croze and Soulage Citation2013) and their complex interactions with both glucose and lipid metabolism are well demonstrated across tissue types and in many species (Larner, Brautigan, and Thorner Citation2010; Müller et al. Citation1998; Romero and Larner Citation1993; Saltiel Citation1990; Hansen Citation2015; Yamazaki, Zawalich, and Zawalich Citation2010; Nielson and Rutter Citation2018; Kunjara et al. Citation1999; Tabrizi et al. Citation2018). In humans, perturbations in inositol synthesis, metabolism and excretion are associated with metabolic conditions such as polycystic ovary syndrome (PCOS), diabetes mellitus and metabolic syndrome (Asplin, Galasko, and Larner Citation1993; Larner, Brautigan, and Thorner Citation2010; Croze and Soulage Citation2013; Heimark, McAllister, and Larner Citation2014; Ceriello et al. Citation1988; Croze, Géloën, and Soulage Citation2015; Sun et al. Citation2002), as well as perinatal disorders including gestational diabetes (GDM) (Crawford et al. Citation2015; D’Anna and Santamaria Citation2018), pre-eclampsia (D’Oria et al. Citation2017) and intrauterine growth restriction (IUGR) (Dessì and Fanos Citation2013). Alterations are most prominent in nervous and reproductive tissue, but the extent and foci of the inositol-related pathophysiology in insulin resistant conditions and pregnancy complications remains unclear (Asplin, Galasko, and Larner Citation1993; Daughaday and Larner Citation1954; Kennington et al. Citation1990).
Several small clinical trials suggest that inositol supplementation could be a useful intervention or preventive measure for the insulin-related and metabolic abnormalities in PCOS, GDM and type 2 diabetes (Noventa et al. Citation2016; Croze and Soulage Citation2013; Crawford et al. Citation2015; Papaleo et al. Citation2007; D’anna et al. Citation2012; Gateva, Unfer, and Kamenov Citation2018; Colazingari et al. Citation2013; Bizzarri and Carlomagno Citation2014; Unfer and Porcaro Citation2014; Muscogiuri et al. Citation2016; Lubin et al. Citation2016; Brown, Crawford Tineke, and Alsweiler Citation2015; Farren et al. Citation2017; Malvasi et al. Citation2014; Pintaudi, Di Vieste, and Bonomo Citation2016; D'Anna et al., Citation2013; Matarrelli et al. Citation2013; Werner et al. Citation2016; DʼAnna et al. Citation2015; D'Anna et al., Citation2013; Cogram et al. Citation2002; Cavalli et al. Citation2011). Inositol supplementation appears to decrease glycemia and improve insulin sensitivity, but effects vary depending on the inositol isomer used, the dose and the population studied (D’anna et al. Citation2012; DʼAnna et al. Citation2015; D'Anna et al., Citation2013; Kim et al. Citation2005; Santamaria et al. Citation2012; Giordano et al. Citation2011; Artini et al. Citation2013; Genazzani et al. Citation2012; Santamaria, Di Benedetto, et al. Citation2016; Corrado et al. Citation2011; Fraticelli et al. Citation2018). Other small clinical trials have also suggested that inositol supplementation might reduce plasma triglycerides and total and LDL-cholesterol levels among patients with diabetes mellitus, hyperinsulinemia, metabolic syndrome and PCOS (Tabrizi et al. Citation2018), thereby highlighting a natural compound that could address the increased risk of atherosclerotic and cardiovascular diseases associated with these conditions.
Current evidence suggests that each inositol isomer and derivative affects metabolism in distinct ways (Thomas, Mills, and Potter Citation2016). Furthermore, these compounds appear to be regulated differently in different tissues and in different populations even within apparently similar clinical phenotypes (). In this review we will examine how inositol isomers and derivatives regulate glucose and lipid metabolism, and discuss how disruptions in inositol synthesis, metabolism or excretion could be involved in conditions of insulin dysregulation.
Pregnancy is characterized by substantial and complex changes in glycemic and lipidomic regulation, brought about by signals released by the fetal-placental unit (Di Cianni et al. Citation2003). The placenta is situated at the maternal-fetal interface, where it ensures appropriate fetal nutrition throughout gestation, by regulating maternal-fetal cross talk and nutritional transfer (Gallo, Barrett, and Nitert Citation2017). Dysregulation of these processes in pregnancy complications such as GDM and pre-eclampsia cause disordered fetal growth and threaten both maternal and fetal health (Gallo, Barrett, and Nitert Citation2017; Uhl et al. Citation2015; Herrera and Ortega-Senovilla Citation2018; Larqué et al. Citation2014; Philipps et al. Citation2011). Impact on offspring is often long term because the in-utero environment shapes life-long health (Developmental Origin of Health and Disease (Barker Citation2004)).
The fetal-placental unit is rich in inositol, and inositol concentrations in fetal and neonatal circulations are much higher than that in adults (Brusati et al. Citation2005; Toh et al. Citation1987; Santamaria, Di Benedetto, et al. Citation2016; Campling and Nixon Citation1954; Pereira et al. Citation1990; Islam, Selvam, et al. Citation2019; Pillai et al. Citation2020; Battaglia et al. Citation1961). Inositols are thus postulated to be important in maintaining normal pregnancy physiology and inositol dysregulation could be a key pathological element in many pregnancy pathologies. In this review we will therefore investigate how inositols could act as critical signals in maternal-placental-fetal communications and how they might regulate fetal nutritional supply, growth, adiposity and tissue programming.
Review criteria
Overall, this review aimed to examine the current literature on the biology of inositols in conditions of insulin dysregulation, with a focus on their role within the maternal-placental-fetal axis. This review was conducted in the Department of Obstetrics and Gynecology at the National University of Singapore and includes studies published in English up until March 2020. Articles were identified manually using Pubmed, Science Direct and Google Scholar using the key words described in Supplementary Table S1 and by following relevant references in articles of interest. Human and animal studies, clinical, randomized controlled trials (RCTs), cross-sectional and prospective studies, in-vitro studies, cell studies, genetic studies, reviews and meta-analyses were all included.
Inositol biology
Inositol stereoisomers and their derivatives
Inositol distribution and content in tissues and fluids
Myo-inositol is the most abundant and well-studied inositol, but there are seven other natural inositol stereo-isomers: D-chiro‐inositol, L-chiro-inositol, epi‐inositol, allo‐inositol, muco‐inositol, neo‐inositol and scyllo-inositol, and one synthetic stereo-isomer: cis-inositol (Thomas, Mills, and Potter Citation2016). Only myo-inositol, D-chiro-inositol, L-chiro-inositol, neo-inositol and scyllo‐inositol have so far been found in mammals (Thomas, Mills, and Potter Citation2016; Stewart, Sherman, and Harris Citation1970). Reported inositol concentrations in human and mammalian adult and fetal tissues, and in the circulation are summarized in .
Table 1A. Unspecified inositols (mmol/ kg wet tissue) analyzed by methods that do not differentiate between inositol isomers.
Many older methods were not able to quantify different inositol isomers separately ( and ), whilst newer techniques such as gas chromatography (GC), high performance liquid chromatography (HPLC) and nuclear magnetic resonance (NMR) have enabled the quantification of some individual isomers ().
Table 1B. Specified inositols in tissues.
Table 1C. Unspecified inositols in fluids (µmol/L) by methods that do not differentiate between inositol isomers.
(Garcia-Perez and Burg Citation1990)Inositols isolated and weighed Adult Boar Seminal: 110,000-167,000
Table 1D. Specified isomers in fluids (µmol/L).
Inositol is ubiquitous in the body, but content is particularly high in the central nervous system (CNS), kidney, and male and female reproductive organs, suggesting an important role for inositol in these tissues (). Several studies suggest that inositols are critical for nervous system function (Fisher, Novak, and Agranoff Citation2002; Thurston et al. Citation1989) and inositol is known to play a key role in dopamine, serotonin, noradrenaline and acetylcholine neurotransmission (Shaldubina et al. Citation2007). Indeed, brain inositol dysregulation has been associated with Down syndrome (Berry et al. Citation1999), bipolar disorder (Calker and Belmaker, Citation2000), schizophrenia (Shimon et al. Citation1998), aging (Stokes, Gillon, and Hawthorne Citation1983), epilepsy (Pascente et al. Citation2016) and Alzheimer's disease (Stokes and Hawthorne Citation1987). Inositols were also found to be crucial for testicular and ovarian function, with dysregulation associated with male and female infertility (Steegers-Theunissen, Groenen, and Beemster Citation2002; Condorelli et al. Citation2017; Unfer et al. Citation2017; Papaleo et al. Citation2009).
Isomeric composition of inositols in circulation, urine and tissues
The amount and isomeric composition of inositol in any cell, and the consequent biological effects, are influenced by systemic and local factors. Systemic inositol concentrations are determined by inositol intake, bioavailability and excretion. Locally, cellular levels are regulated by cellular uptake and efflux, isomeric conversion, synthesis, catabolism and metabolism into inositol-containing derivatives (Holub Citation1986). Myo-inositol comprises ∼98% of adult human plasma inositol and ∼71% of urinary inositol (Kalra, Kalra, and Sharma Citation2016). D-chiro-inositol is commonly quoted as the second most abundant inositol with plasma D-chiro-inositol being 3% relative to plasma myo-inositol, and urinary D-chiro-inositol being 21- 29% relative to urinary myo-inositol (Campbell et al. Citation2004; Stull et al. Citation2008). However, this may not be the case, since most methods used cannot distinguish between D-chiro-inositol and L-chiro-inositol, and do not quantify scyllo-inositol or neo-inositol. There is also concern that the use of acid in some analytical methods may cause isomerization ex-vivo, leading to an artificial change in the ratio of different inositol isomers (Taguchi et al. Citation1997). Therefore, in many studies inositols may have been missed, mis-identified or mis-analyzed.
Only a handful of studies have described the relative abundance of other inositol isomers. In Sprague Dawley rats, L-chiro-inositol was found in similar concentrations to D-chiro-inositol in muscle phospholipids (skeletal and smooth) and heart phospholipids, but D-chiro-inositol was higher than L-chiro-inositol in fat, liver, brain and kidney phospholipids (Kennington Citation1992; Larner Citation2002). Neo-inositol meanwhile was found in the brain, heart, kidney, testis, and spleen of the rat, but was not detected in rat liver (Sherman, Goodwin, and Gunnell Citation1971). Scyllo-inositol and epi-inositol quantified in human plasma were about 100 times less abundant than myo-inositol (Groenen, Merkus, et al. Citation2003), while scyllo-inositol in the human brain was about five times less abundant than myo-inositol (Michaelis et al., Citation1993; Seaquist and Gruetter Citation1998). In rabbit tissue, the myo-inositol to scyllo-inositol ratio was very high in the spleen, pancreas, testis (45:1, 30:1, 28:1, respectively) and in the brain (19:1 – 25:1 depending on region), but much lower in the sciatic nerve, vagus nerve and lens (12:1, 6.6:1, 10:1, respectively) with similar results seen in rats (Sherman, Stewart, Kurien, et al. Citation1968). This may suggest a more important role for scyllo-inositol in the peripheral nervous system compared with other tissues. Overall, further studies are required to clarify the inositol composition in biological systems.
Different actions of inositol isomers
The shape of inositol isomers affects their ability to interact with enzymes giving them distinct bioactivities (Thomas, Mills, and Potter Citation2016) and altering how these molecules are transported across membranes (). However, all inositol isomers have the same effects when shape does not affect function, such as in osmotic regulation (Thurston et al. Citation1989; Garcia-Perez and Burg Citation1990; Häussinger Citation1998). Inositol isomers are also differently disrupted in various pathologies (). The pharmacological effects of inositol likely depend on the inositol isomer, the dose, and the population studied. For example, daily inositol supplementation resulted in decreased insulin resistance as assessed by the Homeostatic Model Assessment of Insulin Resistance (HOMA-IR) in postmenopausal women with metabolic syndrome [4000 mg myo-inositol (Santamaria et al. Citation2012; Giordano et al. Citation2011)], in non-pregnant women with PCOS [2000 mg myo-inositol (Artini et al. Citation2013; Genazzani et al. Citation2012)], in obese and overweight pregnant women [4000 mg (DʼAnna et al. Citation2015; Santamaria, Di Benedetto, et al. Citation2016) myo-inositol], and in women with GDM with no insulin therapy [4000 mg myo-inositol (Corrado et al. Citation2011; Fraticelli et al. Citation2018)]. However, no change in insulin resistance as assessed by HOMA-IR was observed among pregnant women with GDM treated with D-chiro-inositol [500 mg D-chiro-inositol or a combination of 1100 myo-inositol/27.6 mg D-chiro-inositol (Fraticelli et al. Citation2018)]. However, differences in the statistical power of these studies, and in the methods used, make comparisons difficult.
Table 2. Inositol transport proteins facilitating cellular influx of inositol.
Table 3. Case-control studies of inositol concentrations in non-pregnant populations with diabetes compared with controls.
Table 4. Animal studies of inositol levels in non-pregnant diabetes models.
Table 5. Case-control studies of IPGs in non-pregnant diabetes compared with non-diabetic controls.
Table 6. IPGs in pregnant women with and without diabetes.
Table 7. P-IPG in pre-eclampsia (PE) compared to controls with an uncomplicated pregnancy.
So far, only trials of supplements containing myo-inositol (without other inositols) suggested efficacy in reducing risk of GDM (Zheng et al. Citation2015). Treatment with D-chiro-inositol [500 mg] alone showed no effect on GDM prevention, while combined myo-inositol [1100 mg] and D-chiro-inositol [27.6 mg] treatment has shown no consistent positive effect (Farren et al. Citation2017; Celentano et al. Citation2020).
Unlike in GDM, both myo-inositol and D-chiro-inositol treatments were able to significantly improve endocrine and metabolic parameters in non-pregnant overweight PCOS patients (Formuso, Stracquadanio, and Ciotta Citation2015) (vide-infra). Meanwhile, scyllo-inositol and epi-inositol show promise for neurological function. Scyllo-inositol showed effectiveness in-vitro and in-vivo as potential Alzheimer's disease treatment, decreasing neuronal toxicity, cognitive deficits and the aggregation of amyloid β peptides and increasing long-term potentiation (Ma et al., Citation2012; Ma et al., Citation2012). In rats, scyllo-inositol also showed anti-convulsive effects and maybe useful in treating epilepsy (Nozadze et al. Citation2011), while epi-inositol reduced anxiety (Bersudsky et al. Citation1999; Einat and Belmaker Citation2001). Some inositol isomers may also influence the effects of other inositols (Strieleman et al., Citation1992; Wentzel et al. Citation2001; Salman et al. Citation1999; Strieleman and Metzger Citation1993; Strieleman et al., Citation1992). Scyllo-inositol, for example, in the developing rat conceptus, inhibited myo-inositol uptake and the synthesis myo-inositol derived phosphoinositide and phosphoinositide-phosphate (PIP) (Strieleman et al., Citation1992; Wentzel et al. Citation2001; Salman et al. Citation1999; Strieleman and Metzger Citation1993; Strieleman et al., Citation1992), and these changes were associated with increased structural abnormalities and deceased crown rump length and somite number (Strieleman et al., Citation1992).
Inositol derivatization
Inositols can be derivatized with lipids, phosphates, sugars, proteins and other compounds to form a wide range of bioactive molecules ( and ). A lack of suitable analytical techniques means that little is known about the relative abundance of different derivatives. One gas chromatography study compared water soluble myo-inositol (aqueous extract, probably mainly underivatized) to lipid-bound inositol (organic extraction followed by acidic hydrolysis) (Wells, Pittman, and Wells Citation1965). This study demonstrated that in the brain and kidney most inositol was found in the water-soluble form (83 and 87% respectively), while in the liver most inositol was lipid-bound (94%) (Wells, Pittman, and Wells Citation1965). Meanwhile, an enzymatic assay study which compared water-soluble and total acid hydrolyzable inositol in human placenta found 77% of the inositol in the water-soluble form (Islam, Selvam, et al. Citation2019).
Figure 1. Synthesis, catabolism, excretion, isomerization and derivatization of inositols.
*The synthesis of methylated inositol derivatives has so far only been found in plants and whether this process also occurs in mammals is controversial.
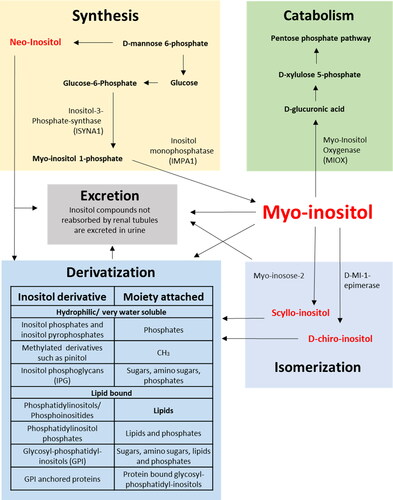
Figure 2. Inositol metabolism leads to the formation of diverse bioactive derivatives many of which interact with insulin signaling pathways. Inositol derivatives containing lipids are shown in gold while those that do not are shown in green. Other compounds are shown in black. Blue arrows and descriptions show selected signaling or metabolic processes affected by inositol or inositol derivatives. Abbreviations: Arachidonic acid (AA), Diacylglycerol (DAG), Glycosyl-phosphatidyl-inositols (GPI), Inositol phosphoglycans (IPGs), Lysophosphatidic acid (LPA), lyso-phosphotidyl inositol (LPI), Phosphoinositide phosphates (PIPs).
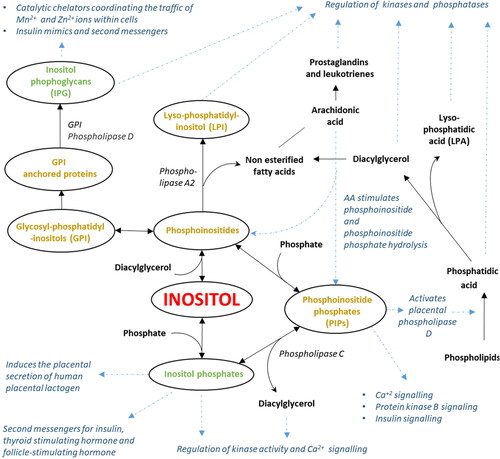
It should be noted that although most quantified water soluble inositol will originate from underivatized inositol, some will be due to the cleavage of other water-soluble inositol derivatives during processing. The degree to which this occurs is not known, but the amount of cleavage will depend on the methods used, with the harsher methods used to prepare samples for gas chromatography likely to cause more cleavage than other methods. The degree of cleavage will also depend on the inositol derivative involved. For example, the phosphate-ester bond in inositol phosphates will be more sensitive to cleavage than the ether bond of pinitol (D-chiro-inositol-O-methyl ether) or the glycosidic bonds of inositol glycans and inositol phosphoglycans (IPGs). Thus, the amount of inositol quantified will depend on extraction, processing and quantification methods used and results cannot easily be compared between studies.
Labeled myo-inositol and D-chiro-inositol injected into rats generally remained as free underivatized inositols (94% and 83.4% respectively), with substantially les s incorporated into inositol phosphates (1.8% and 16.4%), or phospholipids (3.2% and 0.2%), when assessed in all tissues combined after 72 h, suggesting inositol derivatization is isomer dependent (Pak et al. Citation1998).
Inositol synthesis, iomerism and catabolism
Myo-inositol synthesis
Myo-inositol can be endogenously synthesized from glucose by inositol-3-phosphate synthase (ISYNA1) and inositol-1-monophosphatase (IMPA1) (Noventa et al. Citation2016; Holub Citation1986) (). These enzymes are relatively specific to inositol metabolism, although IMPA1 can also hydrolyze galactose 1-phosphate (an intermediate of galactose metabolism) to form galactose (Parthasarathy, Parthasarathy, and Vadnal Citation1997). ISYNA1 is found in all eukaryotes, while IMPA1 is found in all Animalia (Zerbino et al. Citation2018). Although both are ubiquitously expressed in mammalian tissues, ISYNA1 is particularly highly expressed in human placenta and testis, while IMPA1 is especially highly expressed in the testis (Fagerberg et al. Citation2014; Guan, Dai, and Shechter Citation2003). Despite such expression patterns, the kidneys remain the predominant site of myo-inositol synthesis (approximately 4 g/day in humans (Clements Jr Citation1979)), with appreciable amounts also synthesized by the brain [30 µmoles/kg wet weight/h (Stewart, Sherman, and Harris Citation1970)] and testis [316 µmoles/kg wet weight/hr (Stewart, Sherman, and Harris Citation1970; Eisenberg and Bolden Citation1963; Middleton and Setchell Citation1972; Voglmayr and White Citation1971; Hauser and Finelli Citation1963)], consistent with these tissues’ high inositol content ().
Myo-inositol epimerization to D-chiro-inositol
Myo-inositol is thought to be converted into D-chiro-inositol by myo-inositol-D-chiro-inositol-1-epimerase. Epimerization was evaluated in-vivo via the conversion of injected radio-isotope-labeled [3H]-myo-inositol into [3H]-D-chiro-inositol in non-diabetic rats (Pak et al. Citation1992). The relative amount of isotope label found as D-chiro-inositol varied between tissues, ranging from 0.7% in the heart, to 2.2% in liver. Much higher relative amounts of D-chiro-inositol were observed in urine (36%) and blood (60.4%) (Pak et al. Citation1992), which reflect the combined whole body tissue conversion rates. Labeling of other inositol isomers including scyllo-inositol, neo-inositol, epi-inositol, and mucoinositol was less than 0.06%.
Myoinositol epimerase activity appears altered in several conditions of insulin resistance, but the direction of change depends on the condition and tissue studied. Ovarian thecal cells from patients with PCOS showed decreased myo-inositol:chiro-inositol ratios, and increased in-vitro epimerase activity (Heimark, McAllister, and Larner Citation2014; Carlomagno, Unfer, and Roseff Citation2011). In contrast, the muscle, liver, and fat of Goto-Kakizaki diabetic rats showed increased in-vivo myo-inositol:chiro-inositol ratios and decreased in-vivo epimerase activity (Sun et al. Citation2002; Pak et al. Citation1998).
We cannot rule out that differences between these studies could possibly be due to methodological issues, but this does not seem likely. In both cases, in-vivo epimerase activity was quantified by measuring the conversion of myoinositol to chiro-inositol by HPLC. The ovarian study measured the conversion of non-labeled isotopes using an Ag/AgCl electrochemical detector, with peak identity confirmed by GCMS and by comparison to known standards of myo-inositol and D-chiro-inositol. In contrast, the rat study measured the conversion of radiolabeled inositols using a radiolabel detector, a more sensitive technique which automatically adjusts for recovery losses. Peak identity was confirmed by GCMS and by comparison to known standards of myo-inositol, D-chiro-inositol, scyllo-inositol, neo-inositol, epi-inositol, and mucoinositol.
It is more likely that the observed differences are due to the tissues and disorder studied. Treatment with intramuscular insulin increased epimerase activity in the livers of Sprague Dawley rats suggesting that epimerase activity likely depends on insulin availability and insulin sensitivity (Sun et al. Citation2002; Pak et al. Citation1998). The muscle, liver, and fat of Goto-Kakizaki diabetic rats are likely more insulin resistant than controls, which could lead to decreased epimerase activity. In contrast, in PCOS the ovaries, unlike other tissues do not become insulin resistant, but are still exposed to increased insulin leading to increased epimerase activity (Carlomagno, Unfer, and Roseff Citation2011).
Insulin treatment also increased the conversion of total [3H]myo-inositol to [3H]chiro-inositol in a rat fibroblast cell line that expressed the human insulin receptor (Pak et al. Citation1993). However, the increase in radio-label incorporation was mainly observed in the acid hydrolyzed lipid fraction, suggesting either that epimerization occurred in phospholipids rather than free inositol or that the insulin-promoted increase in newly synthesized D-chiro-inositol was rapidly converted into lipids. However, these results may be artefacts brought about by acid treatment and further research is needed.
Controversy about epimerase activity
One study has proposed that the function of the myoinositol epimerase in the previously described rat, ovarian and fibroblast studies had been misidentified, due to the mis-identification of the inositols involved (Lin, Ma, Gopalan, et al. Citation2009). This hypothesis was based on research which suggested that mice cannot convert myo-inositol into D-chiro-inositol and instead rely on dietary D-chiro-inositol (Lin, Ma, Gopalan, et al. Citation2009). D-chiro-inositol was found to fall rapidly in the plasma, stools and urine of mice fed a myo-inositol containing, but pinitol and D-chiro-inositol deficient diet (Lin, Ma, Gopalan, et al. Citation2009). Moreover, mice fed the deficient diet who were fed deuterium-labeled water or injected intraperitoneally with [2H6]-myoinositol produced urine that contained deuterium-labeled myo-inositol, but no deuterium labeled D-chiro-inositol.
However, such evidence alone is not strong enough to suggest that the myoinositol epimerase has been mis-identified. Instead these results could also be explained by low endogenous D-chiro-inositol synthesis compared to dietary intake, the sensitivity limits of stable isotope based analysis, differences in mouse inositol metabolism compared to other species, or to the metabolic consequences a pinitol and D-chiro-inositol-deficient diet for 15 weeks prior to the measurement of the outcome of interest. However, further research on the activity of this epimerase in different tissues and conditions is needed before strong conclusions can be drawn.
The synthesis of other inositol isomers
Myo-inositol is converted into scyllo-inositol by myo‐inosose‐2 (Sherman, Stewart, Kurien, et al. Citation1968; Sherman, Stewart, Kurien, et al. Citation1968; Hipps, Holland, and Sherman Citation1977; Hipps, Ackermann, and Sherman Citation1982). Myo‐inosose‐2 is expressed in a variety of rat and rabbit tissues, but is particularly high in brain, testis and kidneys (Sherman, Stewart, Kurien, et al. Citation1968; Sherman, Stewart, Kurien, et al. Citation1968), suggesting an important role for scyllo-inositol in these tissues. However, whether this is also the case in humans remains to be established. Neo-inositol is synthesized from D-mannose 6-phosphate, but the process is not well understood (Sherman, Goodwin, and Gunnell Citation1971).
Myo-inositol catabolism and excretion
Myo-inositol is catabolized by myo-inositol oxygenase (MIOX) into D-glucuronic acid (Charalampous Citation1959; Arner et al. Citation2001). D-glucuronic acid can then be converted into D-xylulose 5-phosphate, which enters the pentose phosphate pathway (Charalampous Citation1959; Arner et al. Citation2001). The kidney is the primary organ for myo-inositol catabolism (Holub Citation1986), with proximal tubular epithelial cells being the main site of MIOX expression (Arner et al. Citation2006). The kidneys play a major role in regulating inositol excretion with about 95% of filtered inositol being reabsorbed in isolated perfused dog kidney (Troyer et al. Citation1986). Patients with chronic renal failure demonstrate a systemic build-up of inositol which resolves following renal transplantation, showing that healthy kidney function is required for inositol excretion (Clements Jr Citation1979). Nervous tissue can also catabolize myo-inositol and MIOX is highly expressed in the sciatic nerve, pigmented epithelium and lens epithelium suggesting that local inositol content needs to be closely regulated for optimal nervous function (Arner et al. Citation2006).
Inositol transport proteins
Inositol is hydrophilic and requires transporters to cross membranes. Intracellular inositol concentrations are therefore dependent on the expression and activity of transporters. The high levels of inositol in the brain and the female reproductive organs are thought to be due to high inositol import, rather than high local inositol synthesis (Thomas, Mills, and Potter Citation2016; Berry et al. Citation1999; Lewin et al. Citation1982; Di Daniel et al. Citation2009; Bourgeois, Coady, and Lapointe Citation2005; Michaelis et al., Citation1993; Frej, Otto, and Williams Citation2017). Sodium/Myo-Inositol Transporters (SMIT) are expressed in multiple tissues among Animalia (Zerbino et al. Citation2018; Schneider Citation2015), while proton (H+)/Myo-Inositol symporters (HMIT) are found in all living organisms and are closely related to the GLUT family of sugar transporters (Schneider Citation2015; Mueckler and Thorens Citation2013). SMIT and HMIT are symporters, which require a sodium or proton gradient respectively to pump inositol into cells. The affinity of different inositol isomers to each transporter is summarized in .
Regulation of inositol transport is important for maintaining cell osmolality. SMIT-1 is upregulated by intracellular hypertonicity and downregulated by hypotonicity (Schneider Citation2015). Inositol transport is also affected by volume sensitive organic osmolyte anion channels, but the underlying mechanisms are poorly understood (Strange et al. Citation1994; Isaacks et al. Citation1999; Jackson and Strange Citation1993). Other inositol transporters are found in plants, fungi and yeasts, but these have not yet been described in animals and humans (Schneider Citation2015).
Competition between inositol isomers and other saccharides for inositol transporters affects the relative uptake of each inositol isomer. For example, the uptake of myo-inositol by SMIT-1 is inhibited by scyllo-inositol in isolated bovine cardiac sarcolemmal vesicles (Rubin and Hale Citation1993), and by scyllo-inositol and a range of other saccharides in human embryonic HEK293 kidney cell-line (Fenili Citation2010). This raises the possibility that a divergence from a normal ratio of inositol isomers and glucose in the circulation or renal filtrate could impact local inositol uptake and supply.
Inositol transport in pathological conditions
Disrupted inositol transport has been observed in multiple pathological conditions, mostly involving the central nervous system. For example, particular single nucleotide polymorphisms within HMIT are associated with Parkinson’s disease (Satake et al. Citation2009; Gao et al. Citation2012). Meanwhile, the SMIT-1 gene, found on chromosome 21 (Berry et al. Citation1995) and likely over-expressed in Down syndrome (trisomy 21) (Schneider Citation2015; Berry et al. Citation1995; Fruen and Lester Citation1991; Guo et al. Citation1997), may contribute to inositol elevation in brain, cerebrospinal fluid, and amniotic fluid (Berry et al. Citation1999; Lamar et al. Citation2011; Santamaria et al. Citation2014) and an increased risk of Alzheimer's disease (Lamar et al. Citation2011).
Depressive symptoms are associated with decreased myo‐inositol in the frontal cortex of the brain (Willmroth et al. Citation2007; Frey et al. Citation1998; Moore et al. Citation1999; Moore et al. Citation2000; Silverstone, McGrath, and Kim Citation2005; Coupland et al. Citation2005), while increased inositol in the cingulate cortex was observed in children with mania (Davanzo et al. Citation2003; Davanzo et al. Citation2001). Neutrophils in bipolar I disorder, but not bipolar II disorder, expressed higher SMIT mRNA than controls (Willmroth et al. Citation2007). These findings suggest specific regional changes in inositol transport rather than general physiological deficiency in mood disorders (Willmroth et al. Citation2007; Frey et al. Citation1998; Moore et al. Citation1999; Moore et al. Citation2000; Silverstone, McGrath, and Kim Citation2005). Mood stabilizers lithium, valproate and carbamazepine inhibit SMIT activity in astrocyte cultures, and decrease brain inositol levels in bipolar disorder, suggesting a possible mode of action for these medications (Calker and Belmaker, Citation2000; Silverstone, McGrath, and Kim Citation2005; Harwood Citation2005; Lubrich and van Calker Citation1999; Calker and Belmaker, Citation2000; Wolfson et al. Citation2000).
In the diabetic rat model of acute stage streptozocin-induced hyperglycemia SMIT expression increased in the hippocampus, while SMIT-1 knockout mice display reduced inositol in the frontal cortex and hippocampus (Yamashita et al. Citation1998). During human pregnancy, protein expression of SMIT2 and HMIT in the placenta were downregulated with increasing maternal glycemia and this is thought to contribute to lower placental inositol in GDM compared to controls (Pillai et al. Citation2020). Such changes may be directly attributable to glucose since glucose treatment of placental explants in-vitro downregulated SMIT2 mRNA expression (Pillai et al. Citation2020). Understanding the regulation of transporter activity is therefore important for understanding inositol-related pathology and potential side-effects of inositol supplements outside the target tissue.
Oral intake and absorption of inositols
Inositol hexaphosphate (phytate) is an important dietary source of myo-inositol found in cereals and legumes (Schlemmer et al. Citation2009). High fiber diets are rich in phytates and it has been suggested that the health benefits of such diets may be due to inositol (Prynne et al. Citation2010). Phytate is broken down by intestinal microbes to release underivatized inositol, which is then taken up by inositol transporters in the small intestine (Grases et al. Citation2001; Steer and Gibson Citation2002). Experiments in rats have suggested that SMIT-2 in particular maybe involved in intestinal inositol uptake (Aouameur et al. Citation2007). Some studies hypothesized that mammals may also absorb phytate directly, but more recent work in HeLa cells suggests that most physiological phytate is synthesized in-situ (Letcher, Schell, and Irvine Citation2008). Phytate was classically thought to decrease the dietary bioavailability of zinc, iron and copper ions, but recent studies suggest that phytate does not usually affect mineral status in humans (Grases et al. Citation2001). Dietary phytate confers protection against kidney stones, colon, lung and mammary cancer, and has both anti-oxidant and hypocholesterolaemic effects (Grases et al. Citation2001). However, it is unclear whether these effects are due to phytate itself or due to increased inositol availability (Grases et al. Citation2001). Dietary inositol may also originate from lipid-bound inositols, pinitol or 6-β-galactinol (Holub Citation1986; Croze and Soulage Citation2013). Lipid-bound inositols are particularly important, making up 56% of the inositol in a typical American diet (Holub Citation1986; Croze and Soulage Citation2013).
Inositol derivatives
Phosphoinositides (PI)
Inositols can be derivatized with phosphoglycerol and either one or two fatty acids to form lyso-phosphoinositides (LPI; also known as lyso-phosphatidylinositols) and phosphoinositides (PI; also known as phosphatidylinositol) respectively (Traynor-Kaplan et al. Citation2017).
PIs are generally found at relatively low concentrations making up only 2–12% of the total phospholipids in mammalian tissues (White Citation1973; D'Souza and Epand Citation2014), but these lipids are vitally important in maintaining cell architecture, membrane function and dynamics (Di Paolo and De Camilli Citation2006; Shewan, Eastburn, and Mostov Citation2011).
PI species are enriched in poly-unsaturated fatty acids (PUFA), particularly at the SN2 position and 70% of all PI species contain both arachidonic acid (an important omega-6 PUFA) and oleic acid (Traynor-Kaplan et al. Citation2017; D'Souza and Epand Citation2014). PI metabolism therefore regulates the cellular storage and availability of PUFA and the synthesis of PUFA derived signaling molecules such as eicosanoids (Traynor-Kaplan et al. Citation2017; D'Souza and Epand Citation2014). Myo-inositol, D-chiro-inositol, scyllo‐inositol and muco-inositol have all been found in PI in mammals, but the relative abundance of each isomer in such lipids and the biological importance of this variation remains unknown (Fenili et al. Citation2007; Freedman et al. Citation2014; Behuria et al. Citation2018).
Phosphoinositide phosphates (PIP) and inositol phosphates
Phosphoinositide phosphates (PIPs) and inositol phosphates are influential and highly energetic signaling compounds that regulate diverse processes (Croze and Soulage Citation2013; Balla Citation2013; Bitsanis et al. Citation2005). The availability of these transient compounds is rapidly and tightly controlled by the activity of kinases, phosphatases and lipases (illustrated for PIP1 and PIP2 in ), enabling cells to rapidly respond to constantly changing environments (Farese Citation1983).
Figure 3. The availability of phosphoinositides (PI), phosphoinositide phosphates (PIP) and inositol phosphates, and therefore the activity of many signaling processes, is tightly regulated by metabolism. Phosphates may be selectively and specifically added to PI by kinases, or removed by phosphatases, to produce PIP such as PIP1 and PIP2. These PIPs are cleaved by lipases to form inositol phosphates and diacylglycerol (DAG).
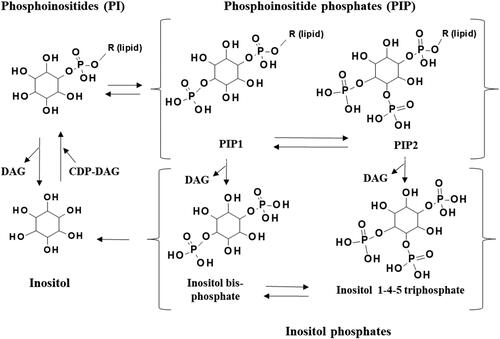
PIPs are produced when the inositol component of PI and LPI lipids is phosphorylated by kinases (Hansen Citation2015; Czech Citation2000). Multiple phosphates may be selectively and specifically added in a variety of configurations and the phosphate moieties of PIPs can be further phosphorylated to form high energy pyro-phosphates (Hansen Citation2015; Czech Citation2000). PIPs can be hydrolyzed by phospholipase C (PLC) to produce a wide range of inositol phosphates, pyro-phosphates and diacylglycerols (DAG), (Hansen Citation2015; Czech Citation2000). Inositol phosphates can also be produced by direct phosphorylation of inositol (as evident in slime molds and plants) (Letcher, Schell, and Irvine Citation2008), or from the further phosphorylation of existing inositol triphosphates (in all organisms) (Letcher, Schell, and Irvine Citation2008; Shears Citation2015). The relative amount of PIPs and inositol phosphates compared to PI in biological systems is difficult to quantify, because these molecules occur at very low concentrations and are very susceptible to degradation during processing (Wenk et al. Citation2003; Fukami and Takenawa Citation1989; Pettitt et al. Citation2006).
PIPs and inositol phosphates act as powerful and versatile signals that mediate processes as varied as cell proliferation, apoptosis, metabolism and motility (Hansen Citation2015; Balla Citation2013; Berridge and Irvine Citation1989). Individual PIPs and inositol phosphates, have distinct bioactivities, but most provide phosphate-ester based energetic currency for kinases (Shears Citation2015) and many regulate Ca2+ signaling pathways (Hansen Citation2015; Czech Citation2000). Cleavage of PIP2 for example forms inositol 1,4,5- trisphosphate (IP3), which stimulates protein kinase C, inducing a cascade of kinase regulated changes (Van Sande et al. Citation2006; Sayers and Hanyaloglu Citation2018; Berridge Citation2009). Interestingly, scyllo‐inositol phosphates were found to act as agonists for myo-inositol-IP3 receptors, suggesting that inositol phosphates containing different isomers might have different, or even antagonistic activities (Wilcox et al. Citation1998; Brautigan et al. Citation2005; Lampe and Potter Citation1993). Many PIPs and inositol phosphates act as second messengers in hormone signaling pathways (Yamazaki, Zawalich, and Zawalich Citation2010; Blazer-Yost and Nofziger Citation2005; Manna and Jain Citation2013). For instance, the binding of insulin to tyrosine kinase receptors activates phosphoinositide 3 kinase (PI3K), that phosphorylates PIP2 to form PIP3 which activates protein kinase B (PKB) causing a cascade of kinase regulated effects (Lizcano and Alessi Citation2002).
Inositol phosphates may show contrasting effects on insulin pathways as part of homeostatic regulation. For example, diphosphoinositol pentakisphosphate (IP7) increases insulin secretion, but impairs insulin signaling (Rajasekaran et al. Citation2018; Barker and Berggren Citation2013; Nagamatsu and Ohara-Imaizumi Citation2007). In pancreatic β-cells, glucose increases the synthesis of IP7 by hexakisphosphate kinase1(IP6K1) from phytate (IP6). Increased IP7 then increases insulin secretion enabling IP7 to regulate first phase insulin release (Rajasekaran et al. Citation2018; Barker and Berggren Citation2013; Nagamatsu and Ohara-Imaizumi Citation2007). However, IP7 itself appears to suppress PKB activity, resulting in reduced insulin signaling (Kim et al. Citation2019). Indeed a reduction in the amount of IP7 via the knockdown of IP6K1 resulted in an increase in PKB signaling in many in-vitro experiments, while a total knockout of IP6K1 in mice was associated with reduced insulin resistance and a phenotype resistant to high-fat diet-induced obesity and diabetes (Kim et al. Citation2019; Mackenzie and Elliott Citation2014). This creates an in-built autocrine homeostatic mechanism where the direct effects of IP7 on suppressing PKB counter-balances those of IP7-induced insulin secretion which would induce PKB activation and ensures responses are timely, transient and highly sensitive to changes in the environment.
Glycosylphosphatidylinositols (GPIs)
Glycans can be attached to PIs containing myo-inositol or D‐chiro‐inositol to form glycosylphosphatidylinositols (GPIs) (Balla Citation2013; Tsai, Liu, and Seeberger Citation2012; Pak and Larner Citation1992). GPIs impact diverse cellular processes and can interact with intracellular targets, or be transported across the cell membrane by transporters such as Glut2 to act as a “hormone” at a distance (Mariggiò et al. Citation2006). GPIs can be attached to proteins which enables these proteins to be anchored into membranes (Paulick and Bertozzi Citation2008).
GPIs and GPI-anchored proteins are particularly important in signal transduction and vesicular trafficking (Paulick and Bertozzi Citation2008). At a cellular level, membrane GPIs in lipid rafts and caveolae are important for lipid raft function, potocytosis and the regulation of K+ and Ca2+ transport (Reeves, Thomas, and Smart Citation2012; Noble, Zhang, and Wray Citation2006), while cleavage of GPI-anchored proteins by phospholipases releases a range of signaling proteins (Low Citation2000). GPIs are also involved in many key physiological functions of the body, as well as pathophysiological processes. GPIs in sperm are essential for egg fertilization, while GPIs synthesized by macrophages modulate immune cell function (Kondoh et al. Citation2005; Patrussi et al. Citation2013). During infection, GPIs produced by protozoan parasites such as Plasmodium and Trypanosoma, induce the pathological production of cytokines, chemokines and nitric oxide (NO) by the host eliciting clinical symptoms such as hypoglycemia, acidosis or anemia (Debierre-Grockiego and Schwarz Citation2010). Several GPIs are also currently under investigation as malaria vaccine candidates (Malik et al. Citation2020). The generation of abnormal GPI-anchored proteins has also been observed in prion disease (Simons and Ehehalt Citation2002).
Inositol phosphoglycans (IPGs) and inositol glycans
The lipid moiety of GPIs may be cleaved off by phospholipase C or D (PLC, PLD) to form inositol phosphoglycans (IPGs) and inositol glycans (Huang et al. Citation1993; Larner, Brautigan, and Thorner Citation2010; Croze and Soulage Citation2013; Kunjara et al. Citation1999; Nestler and Unfer Citation2015). Historically it has been difficult to differentiate between IPGs and inositol glycans, and these classes are often not distinguished in published work. IPGs and inositol glycans commonly act as insulin second messengers, but they can also act as insulin mimics independently from insulin, whilst also having non-insulin related effects (Larner, Brautigan, and Thorner Citation2010; Müller et al. Citation1998). These molecules are thought to regulate enzymes by acting as catalytic chelators, coordinating the traffic of Mn2+ and Zn2+ within cells, enabling them to control kinase activity and a cascade of downstream effects (McLean et al. Citation2008).
As well as having local effects, IPGs and inositol glycans may be released into the circulation as signals to other tissues (McLean et al. Citation2008; Alvarez et al. Citation1991; Turner, Chakraborty, and d'Alarcao Citation2005). The origin of IPGs and inositol glycans in the circulation is still unclear, but the process appears to be regulated by insulin (Scioscia Citation2017; Scioscia et al. Citation2013; Kunjara, Greenbaum, et al. Citation2000). Glucose consumption was associated with a spike in circulating IPG (Baillargeon et al. Citation2006), while IPG release into muscle could be induced by the administration of insulin during euglycemic-hyperinsulinemic-clamp studies (Kennington et al. Citation1990). Furthermore, IPG release by cultured placental trophoblast microvillous membranes could be induced by the addition of insulin to culture media (Scioscia et al. Citation2006; Scioscia et al. Citation2008).
Insulin activates phospholipase C and D, so it has been suggested that increases in IPG and inositol glycans are due to increased cleavage of GPI, enabling IPGs to act as an insulin second messenger (Kennington et al. Citation1990; Pak et al. Citation1993; Goel and Azev Citation2009; Nascimento et al. Citation2006). However, IPG availability will likely also be regulated by GPI availability, IPG transport and IPG metabolism and little is known about how these processes are altered by insulin. Experiments in primary rat hepatocytes demonstrated that radiolabeled IPGs can be transported into cells through an energy-dependent IPG transporter, but nothing else is known about the transport of these molecules (Alvarez et al. Citation1991). IPGs may also affect cellular function by interacting with external cell surface receptors, with fluorescently-labeled IPG stimulating lipogenesis in a rat adipocyte cell line, despite an inability to enter the cell (Turner, Chakraborty, and d'Alarcao Citation2005).
P-IPGs and A-IPGs
The structure of most IPGs and inositol glycans is unknown (Larner, Brautigan, and Thorner Citation2010; Müller et al. Citation1998) so these molecules are classified based on their biochemical properties and our ability to separate them into two fractions. The first fraction (P-IPGs) elutes at pH 2.0 and activates pyruvate dehydrogenase (an enzyme that links the glycolysis metabolic pathway to the citric acid cycle via the production of acetyl-CoA), while the second fraction (A-IPGs) elutes at pH 1.3 and inhibits protein kinase A (an enzyme that modulates glucose and lipid metabolism) (Larner, Brautigan, and Thorner Citation2010). These IPG fractions likely contain both IPGs and inositol glycans, but little is known about the relative amounts of each. Generally, the levels of P-IPG and A-IPG have been quantified by testing these fractions using enzymatic assays (e.g. for pyruvate dehydrogenase activation activity), or by measuring the amount of inositol in these fractions by techniques such as Gas Chromatography Mass Spectrometry (GCMS) (Paine et al. Citation2006). An antibody-mediated assay is also available, but only for a pregnancy-specific P-IPG, and this test only works in urine and not plasma or serum samples (Paine et al. Citation2003). Hence, the development of more discriminatory tests are sorely needed to better characterize IPGs and inositol glycans.
P-IPGs and A-IPGs were classically thought to contain D-chiro-inositol and myo-inositol respectively, but current evidence suggests that both classes contain a range of inositol and inositol phosphate isomers bound to a range of glycans and phosphoglycans, and that many of these compounds have different effects even when they are from the same fraction (Asplin, Galasko, and Larner Citation1993; Elased et al. Citation2001). P-IPG fractions from human hemodialysate have a D-chiro-inositol:myo-inositol ratio of 1.19, whilst those from skeletal muscle extracts have a ratio of 9.42 (Asplin, Galasko, and Larner Citation1993) suggesting that IPG composition varies between biological fluids and tissue types.
The composition and structures of some IPGs have been investigated more closely. For instance, one compound named INS-2 was isolated from the P-IPG fraction of bovine liver and was found to contain galactosamine, pinitol and Mn2+ (Larner, Brautigan, and Thorner Citation2010; Larner et al. Citation2003)). The structure of this molecule was subsequently confirmed by the synthesis of an analogue with identical biological properties (Larner, Brautigan, and Thorner Citation2010; Larner et al. Citation2003). Methylated inositol derivatives such as pinitol, sequoyitol and bornesitol are well known plant secondary metabolites (Owczarczyk-Saczonek et al. Citation2018), but the origin of mammalian pinitol is uncertain and could be dietary or endogenously synthesized (Lin, Ma, Gopalan, et al. Citation2009).
Another compound extracted from a malarial parasite P-IPG fraction was found to contain myo-inositol, phosphorus, galactosamine, glucosamine, and glucose (Elased et al. Citation2001). Meanwhile the makeup of A-IPGs is particularly unclear with some appearing to contain myo-inositol, glucosamine, galactose and ethanolamine (Larner, Brautigan, and Thorner Citation2010).
IPGs influence glucose and lipid metabolism
IPGs alter glucose and lipid metabolism in similar ways to insulin, leading these molecules to be classified as insulin mimics. For example, both P-IPG and A-IPG fractions and INS-2 (a synthetic P-IPG) stimulated the incorporation of labeled glucose into glycogen in rat diaphragm muscles, hepatoma cells, erythroleukemia cells and rat primary adipocytes (Huang et al. Citation1993; Larner et al. Citation2003; Lazar et al. Citation1994; Lawrence, Guinovart, and Larner Citation1977). Furthermore, P-IPG fractions purified from human plasma or bovine liver and synthetic INS-2 stimulated glucose oxidation and lipogenesis in a variety of model systems (Larner, Brautigan, and Thorner Citation2010; Kunjara, Greenbaum, et al. Citation2000; Elased et al. Citation2001; Larner et al. Citation2003; Galasko et al. Citation1996). Moreover, the A-IPG fraction tested in a variety of assays also increased lipid synthesis by decreasing the amount of fatty acids directed toward β oxidation by activating acetyl-CoA carboxylase (ACC) (Huang et al. Citation1993; Kunjara et al. Citation1999) and lowered lipolysis by activating cAMP-phosphodiesterase (Larner, Brautigan, and Thorner Citation2010; Kunjara et al. Citation1999; Scioscia Citation2017; Caro et al. Citation1997; Scioscia, Gumaa, and Rademacher Citation2009; Witters and Kemp Citation1992).
P-IPG can also stimulate the activity of several other regulatory molecules within the insulin signaling pathway including Phosphatidyl Inositol 3-Kinase (PI3K), Protein Kinase B (PKB) and Insulin Receptor Substrate (IRS) proteins (Larner, Brautigan, and Thorner Citation2010; Kunjara et al. Citation1999; Scioscia Citation2017; Varela-Nieto, León, and Caro Citation1996; Burton, Scioscia, and Rademacher Citation2011) and thus could potentially enhance insulin actions, as well as act as insulin mimics in their own right. INS-2, for example was just as effective as insulin in stimulating testosterone production by human ovarian thecal cells in-vitro, and the effect of insulin could be blocked by an anti-INS-2 polyclonal antibody suggesting that INS-2 is critical to this signaling pathway (Nestler et al. Citation1998).
IPGs can also show anti-insulin-like effects. For example the A-IPG fraction was found to inhibit adenylate cyclase (which is normally activated by insulin) and protein kinase A (which normally stimulates insulin secretion) in multiple assays (Larner, Brautigan, and Thorner Citation2010; Kunjara et al. Citation1999; Scioscia Citation2017; Caro et al. Citation1997; Scioscia, Gumaa, and Rademacher Citation2009; Villalba, Kelly, and Mato Citation1988; Scioscia, Gumaa, et al. Citation2007). The A-IPG fraction could also decrease glucose oxidation by antagonizing the in-vitro stimulation of the PDH phosphatase by P-IPG (Kunjara et al. Citation1999; Kunjara, Greenbaum, et al. Citation2000). IPGs also modulate the actions of other hormones besides insulin, with A-IPG inhibiting leptin release from cultured non-pregnant rat adipocytes (Kunjara, Greenbaum, et al. Citation2000). IPGs are therefore important regulators of both glucose and lipid metabolism through insulin-dependent and independent mechanisms (Nestler et al. Citation1998).
Synthetic GPIs and IPGs and the relationship between structure and function
Since the isolation and structural elucidation of natural IPGs has been difficult, chemists have produced a range of synthetic GPIs, IPGs and inositol glycans in order to better understand structure-function relationships and identify possible drug candidates (Larner, Brautigan, and Thorner Citation2010; Goel and Azev Citation2009; Suzuki et al. Citation2014). While many of these candidates showed insulin mimetic activity in various assays, the strength of the effect, and whether the effect was on glucose, glycogen or lipid metabolism varied by molecular structure (Larner, Brautigan, and Thorner Citation2010; Goel and Azev Citation2009; Suzuki et al. Citation2014). These effects were reviewed by Goel et.al. (Goel and Azev Citation2009), who found that high insulin-like activity was more often found for compounds containing a cyclic phosphate on the inositol, an unacylated amino group on the second sugar, and a phosphonate, phosphate or sulfate on one or more of the mannose residues (Goel and Azev Citation2009). Hence, it seems likely that natural GPIs and IPGs will play a wide range of roles in glucose and lipid metabolism and insulin signaling. However, these will not be fully understood until the effects of individual IPGs rather than broad class effects can be reliably quantified.
Inositols influence lipid metabolism, transport and storage
Inositol and its derivatives regulate lipid mobilization, transport and storage, but effects vary depending on the organ and model studied. There is some evidence that myo-inositol supplementation reduces circulating triglycerides, and total and LDL cholesterol levels in patients with metabolic diseases such as diabetes, GDM, PCOS and metabolic syndrome (Tabrizi et al. Citation2018). Myo-inositol supplementation appears to alter body wide lipid distribution and, in general, causes more lipids to be stored in adipose tissue and less to be stored ectopically in the liver and other areas, but this varies depending on the model studied as discussed below (Hayashi et al. Citation1978).
Lipid storage and myo-inositol in animal models
Various animal models have been utilized to examine myo-inositol’s effect on lipid storage and body-wide distribution. Myo-inositol deficiency in gerbils caused a decrease in plasma lipids and lipoproteins, but increased lipid storage in large lipid droplets in intestinal mucosal cells leading to the development of intestinal lipodystrophy (Hegsted et al. Citation1973; Chu and Hegsted Citation1980). In many rat experiments, myo-inositol deficiency was associated with increased adipose tissue lipid mobilization and increased hepatic triacylglycerol (TAG) and cholesterol ester (CE) accumulation while myo-inositol supplementation reversed these effects (Hayashi et al. Citation1978; Andersen and Holub, Citation1980; Burton and Wells Citation1976; Best et al. Citation1946; Gavin and McHenry Citation1941). Alterations to lipid metabolism appeared fatty acid specific, and in rats fed inositol-deficient diets liver phospholipids contained less linoleic acid whilst TAGs contained more palmitoleic acid (Andersen and Holub Citation1976; Andersen and Holub, Citation1980). Increased lipid storage in adipose tissue, rather than ectopically in liver, muscle or other organs is known to associate with decreased cardiometabolic adversity, suggesting that this myo-inositol effect may be beneficial (Hayashi et al. Citation1978; Gaggini, Saponaro, and Gastaldelli Citation2015). However, overall increased obesity could itself lead to negative outcomes and even alter responses to myo-inositol.
Differential lipid storage responses to myo-inositol with obesity
The effects of myo-inositol on adipose tissue appear to be opposite in obese rodent models compared to non-obese models. Myo-inositol supplementation of a non-pregnant high fat diet obese adult mouse model was associated with reduced fat accretion in white adipose tissue and a partial normalization of plasma leptin concentrations (Croze, Géloën, and Soulage Citation2015). Similarly, myo-inositol supplementation in obese PCOS patients, was found to significantly decrease body mass index (BMI) and circulating leptin concentrations (Gerli et al. Citation2007). However, it is unclear whether this reduction in adipose tissue fat accretion is protective (from obesity-related pathology), or whether it is harmful (by increasing ectopic lipid accretion). It is not known why obesity alters the effect of myo-inositol on adipose tissue. Adipocytes from obese populations tend to be more insulin resistant and have low liposynthetic capacity and high lipolytic capacity (Zhang and Zhang Citation2010), and thus might be less likely to respond to insulin-dependent inositol effects while being more likely to release free fatty acids. Adipose tissue in obese populations also shows more pro-inflammatory activity (Zhang and Zhang Citation2010), which may be altered by inositols. Indeed other studies have suggested a role for inositol in many immune and inflammatory processes (Krystal Citation2000; Miller, Chamberlain, and Cooke Citation2008). Hence, obesity modifies the responses of adipose tissue to myo-inositol, and understanding how this occurs will enable the development of strategies to enhance the benefits of myo-inositol supplementation in obese populations.
Myo-inositol status and lipid mobilization during pregnancy and lactation
Myo-inositol and lipid mobilization have also been specifically investigated in the context of pregnancy and lactation. Rats given a myo-inositol deficient diet during pregnancy until 49 days after the birth, developed fatty liver only during lactation and these changes were reversed by treatment with inositol or by the cessation of lactation (Burton and Wells Citation1977). This suggests that myo-inositol deficiency is more dangerous during periods of massive lipid mobilization from adipose tissue, such as during lactation (Oben et al. Citation2010; Vernon Citation2005). The livers of these myo-inositol-deficient lactating rats showed increased numbers of intracellular lipid droplets, which were larger and contained more cholesterol-ester and TAG, but less cholesterol and PI (Burton and Wells Citation1977). Interestingly, no difference in lipids was seen in kidney or intestinal tissues, suggesting a liver-specific effect (Burton and Wells Citation1977). Lactating rats supplemented with inositol and given radiolabeled palmitic acid showed decreased radiolabeled liver TAG, but increased radiolabeled serum TAG compared to animals given an inositol-deficient diet (Burton and Wells Citation1979). Together these findings again suggest inositol selectively modulates lipid transfer and storage around the body producing different effects in different organs and different effects depending on the population studied.
Mechanisms of myo-inositol regulation of lipid mobilization and distribution
Myo-inositol-deficient rats showed increased hepatic fatty acid synthetase and acetyl-CoA carboxylase (ACC) activity, which could explain the increase in hepatic lipogenic activity (Beach and Flick Citation1982). Myo-inositol deficiency in yeasts also increased ACC activity, resulting in an accumulation of neutral lipids and this effect was reversed when the deficiency was rectified by myo-inositol treatment (Hayashi, Hasegawa, and Tomita Citation1976). In contrast, mouse adipocyte cells treated with myo-inositol in-vitro showed increased lipid accumulation due to increased fatty acid synthase expression and reduced lipolysis (Kim, Han, and Kim Citation2014). Collectively, these studies suggest that myo-inositol may potentially reduce ectopic fat deposition by mobilizing lipid stores in the liver, which is an ectopic site, and increasing adipose tissue stores, although this needs to be validated in further studies.
In addition, myo-inositol effects may interact with the autonomic nervous system. Plasma adrenalin levels were higher in myo-inositol-deficient rats, suggesting that myo-inositol may affect the autonomic nervous system and hormonal regulation (Hayashi et al. Citation1978). Moreover, administration of sympathetic nervous system blockers to myo-inositol-deficient rats inhibited hepatic lipid deposition and increased serum free fatty acids (Holub Citation1986; Hayashi et al. Citation1978), indicating that sympathetic nervous activity also modulates myo-inositol effects.
Inositol may also impact cholesterol and lipoprotein metabolism (Hayashi et al. Citation1978; Burton and Wells Citation1977). Myo-inositol-deficient rats showed increased plasma hormone-sensitive lipase activity in epididymal adipose tissues, which would increase the local breakdown of circulating lipoproteins and increase lipid uptake (Hayashi et al. Citation1978). Myo-inositol deficient rats also showed reduced plasma lecithin–cholesterol acyltransferase (LCAT) activity suggesting decreased cholesterol ester and lipoprotein formation (Burton and Wells Citation1977; Wells and Hogan Citation1968). Additionally, intravenous injection of PI into rabbits inhibited the transfer of hepatocyte cholesteryl esters into the blood stream and increased hepatic uptake of plasma cholesterol and cholesterol biliary secretion (Burgess et al. Citation2003; Stamler et al. Citation2000). Plasma from rabbits treated with PI also showed lower LCAT activity within 10 minutes of PI injection. Since PI will not be converted into myo-inositol in this short period, it seems likely that PI, rather than unesterified inositol, is responsible for altered cholesterol metabolism. Inositols also appear to regulate the synthesis and hydrolysis of PI (Strieleman et al., Citation1992; Wentzel et al. Citation2001; Salman et al. Citation1999). Scyllo-inositol for example inhibited the incorporation of myo-inositol into PI and the hydrolysis of PIP into myo-inositol phosphates in the developing rat conceptus (McPhee, Downes, and Lowe Citation1991). D-chiro-inositol meanwhile, was found to be a potent inhibitor of mycobacterial PI synthase (Salman et al. Citation1999). Overall, how inositols and their derivatives are involved in lipid metabolism in different species, metabolic conditions, tissue types, and various stages of development is still not well understood and further research is necessary. The effects of inositol supplementation on lipid metabolism in GDM pregnancy will be discussed in section “Inositol and fetal development.”
The anti-oxidant effects of inositol
Inositols can act as anti-oxidants and reactive oxygen species (ROS) scavengers (Valluru and Van den Ende Citation2011; Hu, Chen, and Lin Citation1995). In Jian carp, myo-inositol was shown to increase enzymatic anti-oxidant capacity by altering the activity of catalase, glutathione peroxidase and glutathione reductase superoxide dismutase and glutathione-S-transferase (Jiang et al. Citation2009). Furthermore one small study also suggested that there is an increase in thiol oxidation and oxidative stress in the follicular fluid of patients with PCOS compared to controls and that this oxidation was reduced by the administration of D-chiro-inositol (De et al. Citation2012; Dona et al. Citation2012). Uncomplicated pregnancy, pre-eclampsia, diabetic pregnancy, GDM and diabetes in non-pregnant populations are also all associated with increased oxidative stress in general (Wentzel et al. Citation2001; Burton and Jauniaux Citation2004; Jenkins et al. Citation2000; Eriksson and Borg Citation1991; Eriksson and Borg Citation1993; Wentzel, Welsh, and Eriksson Citation1999; Trocino et al. Citation1995; Newsholme et al. Citation2007), but further research is needed to determine how this is related to inositol dysregulation.
Inositols are important for male fertility, testicular function and spermatogenesis (Steegers-Theunissen, Groenen, and Beemster Citation2002; Condorelli et al. Citation2017) and a small clinical study showed that myo-inositol supplementation could be used to treat idiopathic male infertility (Calogero et al. Citation2015). The biology behind this is not yet known, but it has been suggested that the anti-oxidant effects of inositol may play an important role (Colone et al. Citation2010). In particular, in-vitro myo-inositol treatment of spermatozoa reduced ROS activity and mitochondrial cristae damage and reduced the number of spermatozoa covered with amorphous fibrous material, which gives an excessive viscosity to the seminal fluid that increases subfertility (Colone et al. Citation2010).
Inositol in diabetes mellitus
Inositol bioavailability is altered in diabetes
Circulating and urinary D-chiro-inositol and myo-inositol levels are altered in adult type 1 and type 2 diabetes populations compared with non-diabetic controls (), with most studies reporting decreased urinary D-chiro-inositol and increased urinary myo-inositol in both types of diabetes. However, two studies on overweight and obese populations found an increase in both urinary D-chiro-inositol and myo-inositol (Ostlund et al. Citation1993; Jung et al. Citation2005). This difference was discussed by Larner et.al. Citation2010, who noted that D-chiro-inositol excretion also increased with increasing obesity and insulin resistance in PCOS. A similar increase is also observed in GDM as discussed in section “Maternal inositols in an uncomplicated pregnancy and lactation.” Taken together, it appears that diabetes, obesity and PCOS may each have different and independent associations with circulating and urinary inositol concentrations.
Obesity alters the relationship between inositols and diabetes
The differences in systemic inositol concentrations between obese and normal weight diabetes populations is also apparent in animal studies (). Studies using rodent models of diabetes associated with obesity such as streptozocin-treated rats and db/db mice, showed increased urinary D-chiro-inositol and myo-inositol compared with non-diabetes controls (Kawa, Przybylski, and Taylor Citation2003). In contrast, those from the non-obese Goto-Kakizaki diabetic rat model showed decreased urinary D-chiro-inositol and increased myo-inositol compared with controls (Suzuki et al. Citation1991). These findings further support the idea that obesity may modify the associations of inositol dysregulation with the diabetic state.
However, most previous human and animal studies did not adequately adjust for BMI or bodyweight as a covariate, nor use weight-matched controls. Many studies also defined their study populations by insulin-dependence which makes it difficult to discern the associations of inositol alterations with different subtypes of diabetes or treatments. Many of the patients were also taking oral hypoglycemic agents, with or without insulin, but these populations were not analyzed separately. Further research will be necessary to determine how inositol bioavailability and action is altered in diabetes in different patient populations, the direction of causality and to determine whether urinary or circulatory inositols could be used as a tool to screen for, monitor treatment and assess progression of diabetes.
Inositol correlations with degree of insulin resistance
Changes in systemic and local inositol levels in diabetes are strongly correlated with disease severity and insulin resistance. For example, in spontaneously diabetic rhesus monkeys, the urinary D-chiro-inositol excretion rate is directly correlated with insulin-mediated glucose disposal rates and glucose tolerance (Ortmeyer, Huang, et al. Citation1993). Urinary D-chiro-inositol was also correlated with increased glycogen synthetase activity in skeletal muscle and adipose tissue, and decreased activity in glycogen phosphorylase in skeletal muscle (Ortmeyer, Huang, et al. Citation1993). This suggests D-chiro-inositol itself could be involved in the regulation of glycogen synthesis, which is known to be impaired in type 2 diabetes (Ashcroft et al. Citation2017; Cline et al. Citation1999). In humans, urinary D-chiro-inositol was also strongly correlated with fasting plasma glucose, glycated hemoglobin and urinary glucose, and the urinary increase in D-chiro-inositol with diabetes was reversed by insulin treatment (Ostlund et al. Citation1993). Although this suggests that increased D-chiro-inositol excretion may cause or be caused by insulin deficiency or by hyperglycemia, it does not exclude the possibility that increased D-chiro-inositol excretion is an adaptive or protective response against diabetes-induced complications or could result from diabetes-related secondary pathology.
Tissue inositol content in diabetes
In humans, how tissue inositol content is changed with diabetes is not known. A few studies (Asplin, Galasko, and Larner Citation1993; Kennington et al. Citation1990) measured the myo-inositol and D-chiro-inositol content in IPG fractions in human muscle tissue (see ), but IPGs represent only a small fraction of the total inositol present in cells and biological systems, and IPG quantification cannot show if diabetes is associated with general tissue inositol deficiency. In animal studies strong evidence for myo-inositol depletion in diabetes is only present for nervous tissue (). Further studies are needed to determine how inositol metabolism is changed in both type 1 and type 2 diabetes and how this is altered by insulin, oral hypoglycemic or lifestyle/dietary treatments. Findings will depend on how well inositol isomers are separately quantified and what inositol derivatives are included in the extraction and analysis methods.
IPGs and diabetes
IPGs are a diverse group of compounds, which can regulate glycemic and lipidomic pathways (Larner, Brautigan, and Thorner Citation2010), and are altered in diabetes (). Urinary and tissue P-IPGs are generally decreased in type 2 diabetes (Asplin, Galasko, and Larner Citation1993; Kunjara et al. Citation1999; Kennington et al. Citation1990), whilst urinary and tissue A-IPGs are increased, but findings varied depending on the population studied and the analysis method (). In one study, the urinary P-IPG:A-IPG ratio was decreased in diabetes and the ratio was negatively correlated with a rise in systolic blood pressure, BMI and hemoglobin-A1c (HbA1c), which are all markers of worsening metabolic syndrome (Kunjara et al. Citation1999). P-IPG has insulin-like effects (Kunjara et al. Citation1999; Scioscia Citation2017; Scioscia, Gumaa, and Rademacher Citation2009), so a decrease in P-IPG may promote diabetogenic effects. A-IPG in contrast, may direct metabolism away from glucose oxidation and toward energy conservation and lipid storage promoting a diabetic phenotype (Kunjara et al. Citation1999; Scioscia Citation2017; Kunjara, Greenbaum, et al. Citation2000; Scioscia, Gumaa, and Rademacher Citation2009). However, P-IPG and A-IPG fractions both contain many different myo-inositol, D-chiro-inositol and pinitol containing IPGs with a range of bioactivities. Until these can be separately analyzed, and their individual effects quantified, it will be difficult to deepen our understanding of the role of these molecules in diabetes.
IPG concentrations display sex differences. Urinary A-IPG is five-fold higher in healthy female urine than healthy male urine samples and there is a decrease in the P-IPG:A-IPG ratio in women compared with men (Kunjara, Greenbaum, et al. Citation2000), yet most studies do not take these sex differences into account. Further studies accounting for sex, type of diabetes, diabetic treatment and BMI are needed to understand how IPGs are altered in different tissues and bodily fluids in type 1 and type 2 diabetes.
Alterations in inositols in diabetes: pathological or adaptive?
Although inositols participate in numerous insulin, glucose and lipid metabolic pathways, (Yamazaki, Zawalich, and Zawalich Citation2010; Manna and Jain Citation2013; Coustan Citation2013) it is not known if these inositol perturbations have an etiological role in any diabetic features, or if they are a consequence of the pathology. These inositol-related changes could even be protective. For example, myo-inositol-derived PIP3 enhances the transport of glucose into cells by stimulating the translocation of GLUT4 to the cell membrane (Paul and Brady Citation2015). One simple perspective is that a decrease in tissue myo-inositol and consequently PIP3 could therefore decrease glucose uptake and promote hyperglycemia, contributing to diabetes pathology. However, a decrease in tissue myo-inositol could also result in lower intracellular glucose levels, protecting specific cells from glucose overload in the context of hyperinsulinemic hyperglycemia. The association between inositol perturbations and diabetes is also likely tissue-specific and influenced by many factors such as type of diabetes, BMI or ethnicity.
Inositols as treatment for diabetes in humans
In general, inositol supplementation appears to reduce glycemia and increase insulin sensitivity, suggesting that inositol supplementation could suppress diabetic features even in the absence of inositol deficiency. A systematic review and meta-analysis that included 20 RCTs with a total of 1239 subjects with different types of insulin resistant conditions, which studied the effects of inositol on glucose homeostasis, showed that inositol treatment decreased fasting plasma glucose (mean difference [MD] − 0.44 mmol/l, 95% CI −0.65, −0.23), 2 h plasma glucose after a 75 g oral glucose load (MD −0.69 mmol/l, 95% CI −1.14, −0.23), the incidence of abnormal glucose tolerance (relative risk [RR] 0.28, 95% CI 0.12, 0.66), fasting insulin (MD −38.49 pmol/l, 95% CI −52.63, −24.36) and the Homeostatic Model Assessment of Insulin Resistance (HOMA-IR) (MD −1.96, 95% CI −2.62, −1.30) (Miñambres et al. Citation2019). However, this review included an extremely heterogeneous set of studies and did not analyze the effects of inositol in diabetes separately from the effects of other insulin resistant conditions such as PCOS. Furthermore, this review did not adequately differentiate between the use of different types of inositol interventions, although most utilized myo-inositol.
Trials including D-chiro-inositol supplementation are scarce. One small trial involving twenty men with type 2 diabetes showed that the administration of both myo-inositol and D-chiro-inositol in combination for three months, lowered fasting blood glucose and HbA1c levels compared to pretrial baseline (Pintaudi, Di Vieste, and Bonomo Citation2016). A separate 24 weeks’ long RCT involving 26 overweight patients with type 1 diabetes tested the effect of D-chiro-inositol (1 g, plus 400 mcg folic acid daily) compared to a folic acid control (Maurizi et al. Citation2017). A significant reduction in HbA1c level was seen in the treated group compared with control, but no significant reduction in BMI or insulin requirements was observed (Maurizi et al. Citation2017).
Several studies of patients with type 2 diabetes (Kim et al. Citation2005; Kang et al. Citation2006; Kim et al. Citation2012; Kim et al. Citation2007) and non-diabetic adults (Hernández-Mijares et al. Citation2013), suggested that pinitol (3-O-methyl-D-chiro-inositol) can also improve glycemic control. However, small studies in non-diabetic older subjects (66 ± 8 years) (Campbell et al. Citation2004) and non-diabetic obese subjects (Davis et al. Citation2000), suggested that pinitol treatment had no impact on fasting glucose, insulin-mediated glucose disposal, or plasma lipids. Therefore, whilst these initial small studies show promise and whilst there has been no mention of serious adverse effects, larger randomized controlled double-blind trials are required to determine whether treatment with any inositol isomer or inositol derivative could be used to reduce diabetes risk, or to complement current established treatments for diabetes and other conditions of insulin dysregulation.
Inositols as treatment for diabetes in animal models and possible mechanisms of action
D-chiro-inositol treatment lowered postprandial glycemia in streptozocin-induced hyperglycemic rats, wild-type rats and rhesus monkeys with varying degrees of spontaneous insulin resistance (Ortmeyer, Huang, et al. Citation1993; Hansen and Bodkin Citation1986). Furthermore, in obese insulin-resistant monkeys, myo-inositol or D-chiro-inositol supplementation decreased postprandial plasma glucose concentrations, while myo-inositol also decreased urinary glucose (Ortmeyer Citation1996). Meanwhile, the supplementation of streptozotocin and high fat diet induced diabetic rats with myo-inositol decreased fasting glycemia, HOMA-IR and plasma insulin compared with diabetic controls (Antony et al. Citation2017).
Supplementation of diabetic rats with myo-inositol has also been associated with an improvement in several other diabetic-associated changes including glomerular filtration rate, serum urea, creatinine and arachidonic acid, urinary albumin, IgG excretion, endoneural blood flow and motor nerve conduction velocity (Antony et al. Citation2017; Carlomagno and Unfer Citation2011; Pugliese et al. Citation1990; Coppey et al. Citation2002; Solomonia et al. Citation2010; Mayer and Tomlinson Citation1983; Yue et al. Citation1989). In obese insulin-resistant monkeys, neither D-chiro-inositol nor myo-inositol altered post-prandial circulating insulin levels, suggesting that inositol may have an effect on downstream insulin pathways (Ortmeyer Citation1996).
In streptozotocin and high fat diet induced diabetic rats, myo-inositol treatment increased the adipose tissue expression of PPARγ and significantly upregulated GLUT4 and insulin receptor signaling molecules, leading to the suggestion that myo-inositol acts as a PPARγ agonist (Antony et al. Citation2017).
Injected intravenously, both A-IPG and P-IPG fractions from various natural sources (Elased et al. Citation2001; Larner et al. Citation2003) and synthetic INS-2 (Larner et al. Citation2003) could also dose-dependently reduce hyperglycemia in streptozocin-induced diabetic rats (Larner et al. Citation2003), db/db mice, and ob/ob mice (Elased et al. Citation2001), suggesting that IPGs may be partly responsible for the insulin like effects of inositol treatment.
Myo-inositol may also decrease glycemia by reducing dietary glucose absorption in rats (Chukwuma, Ibrahim, and Islam Citation2016). Myo-inositol was found to inhibit duodenal glucose absorption, reduce post-prandial glucose excursions, delay gastric emptying and accelerate intestinal transit in both normal and diabetic (fructose-fed and streptozocin-injected) rats (Chukwuma, Ibrahim, and Islam Citation2016). These effects were observed both in ex-vivo studies where isolated rat jejunum were treated with increasing concentrations of myo-inositol and in-vivo in rats given oral myo-inositol co-administrated with glucose (Chukwuma, Ibrahim, and Islam Citation2016).
Proposed mechanisms underlying inositol dysregulation in diabetes
Myo-inositol depletion by increased excretion
It has been suggested that systemic myo-inositol deficiency could result from increased urinary inositol excretion due to increased competition with glucose for transporters during renal tubular reabsorption of the glomerular filtrate (Daughaday and Larner Citation1954; Kennington et al. Citation1990; Baillargeon et al. Citation2006), resulting in reduced inositol reabsorption (Asplin, Galasko, and Larner Citation1993; Thomas, Mills, and Potter Citation2016; Bourgeois, Coady, and Lapointe Citation2005). However, hyperglycemia is not always associated with increased urinary inositol excretion, nor with systematic inositol deficiency or universally decreased tissue inositol (), and there is variation between isomers, suggesting alterations in circulating inositols in diabetes are due to more complex processes (Loy et al. Citation1990).
Strong evidence for myo-inositol depletion in diabetes is so far only evident for nervous tissue (). Myo-inositol is consistently depleted in the nervous tissue of diabetic rats and rabbits across studies (Greene et al. Citation1987; Loy et al. Citation1990; Palmano, Whiting, and Hawthorne Citation1977; Zhu and Eichberg Citation1990; Greene, De Jesus, and Winegrad Citation1975; Greene and Mackway Citation1986), while human retinal pigment epithelial cells incubated in high glucose showed decreased myo-inositol content and decreased PI synthesis (Thomas et al. Citation1994; Thomas et al. Citation1993; Del Monte et al. Citation1991; Nakamura et al. Citation1992). However, since inositol synthesis (Stewart, Sherman, and Harris Citation1970), catabolism (Arner et al. Citation2006) and transport (Berry et al. Citation1999) processes are particularly active in nervous tissue, this tissue is unlikely to be representative of changes occurring in other tissues in diabetes.
Altered transporter protein activity
Tissue inositol content is regulated by inositol transporter proteins with varying affinities for different inositol isomers. These transporters’ different isomeric specificity () may partly explain the different D-chiro-inositol and myo-inositol profiles in diabetes. Meanwhile, at a systemic level, altered intestinal or kidney transport could lead to altered inositol absorption and excretion, and hence, systemic inositol concentrations. Skeletal muscle SMIT-2 activity is upregulated by insulin, as evidenced by an 18-fold increase in D-chiro-inositol transport in insulin-treated rat skeletal muscle cells that were transiently transfected with human SMIT-2 (Lin, Ma, Gopalan, et al. Citation2009). In contrast, tumor necrosis factor α (TNFα), a critical factor in the pathophysiology of diabetes mellitus (Navarro-González et al. Citation2009), caused a decrease in SMIT-1 mRNA expression and activity in adipocytes (Yorek, Dunlap, and Lowe Citation1998). Differential alterations in locally regulated inositol transporter activity could therefore lead to varying disruptions in inositol content in tissues.
Dysregulated inositol metabolism
Inositol concentrations in diabetes are locally controlled by the relative balance of inositol synthesis, epimerization, derivatization and catabolism. Glucose increased ISYNA1 expression in HepG2 (human liver-derived) cell line, suggesting that diabetes may increase hepatic myo-inositol synthesis (Guan, Dai, and Shechter Citation2003). Furthermore, myo-inositol catabolism appears to be promoted by upregulation of MIOX in human and porcine kidney cells (HK-2 or LLC-PK1) treated with high glucose (Zhan et al. Citation2015; Xie et al. Citation2010), and by increased MIOX expression in the kidneys of mice fed a high fat diet (Tominaga et al. Citation2016). The production of many IPGs could also be increased in diabetes, since the hydrolysis of membrane proteins, including GPI-anchored proteins from which IPGs are released, by phospholipase C is activated by transforming growth factor (TGF)‐β, a factor which is over-expressed in diabetes (Ziyadeh Citation2004; Vivien et al. Citation1993).
Myo-inositol to D-chiro-inositol epimerase is insulin sensitive with evidence of diminished conversion in-vivo in the insulin resistant Goto-Kakizaki (GK) rat model of type 2 diabetes, resulting in increased myo-inositol, but decreased D-chiro-inositol in insulin sensitive tissues such as the liver, fat, muscle and blood, but not in the non-insulin-sensitive spleen, kidney, intestine, heart and brain (Sun et al. Citation2002; Pak et al. Citation1998). It has been proposed that a deficit of D-chiro-inositol could impair the synthesis of IPGs containing D-chiro-inositol, explaining the observed D-chiro-inositol-IPG deficiency in skeletal muscle, hemodialysate and urine of patients with type 2 diabetes (Asplin, Galasko, and Larner Citation1993). However, it is unclear whether D-chiro-inositol availability is the rate limiting factor in D-chiro-inositol IPG synthesis.
PI/PIP/Inositol-phosphate signaling pathways, which regulate many insulin-dependent processes, are also dysregulated in diabetes. SHIP-2 for example, inhibits peripheral insulin signaling, by catalyzing the conversion of PIP3 to PIP2 (Soeda et al. Citation2010) and SHIP-2 knockout mice showed increased liver and skeletal muscle insulin sensitivity (Soeda et al. Citation2010). SHIP-2 protein is significantly increased in the quadriceps muscle, epididymal fat tissue and brain, but not in the liver, of diabetic db/db mice relative to controls (Soeda et al. Citation2010; Hori et al. Citation2002). Furthermore, mutations in SHIP-2 are associated with diabetes and the metabolic syndrome in population studies (Ishida et al. Citation2006; Kagawa et al. Citation2005; Marion et al. Citation2002), which may explain why some individuals have increased susceptibility to developing diabetes.
Other mechanisms
Receptors and effectors of inositol compounds and their derivatives are also dysregulated in diabetes. For example, insulin-induced activation of glycerol-3-phosphate acyltransferase by D-chiro-inositol-containing IPGs appeared defective in the adipocytes of type II diabetic Goto-Kakizaki rats and was associated with altered lipid synthesis (Farese et al. Citation1994). Meanwhile, renal type I inositol-1,4,5-trisphosphate receptor expression was reduced in the kidneys of diabetic rats and mice (Sharma et al. Citation1999) and a polymorphism in this gene was found to be a risk factor for type 1 diabetes in a genetic mapping study (Swedish cohort) (Roach et al. Citation2006).
Polycystic ovary syndrome
Polycystic ovary syndrome (PCOS) afflicts 6-10% of women worldwide and is the most common endocrine disorder in young women (Goodarzi et al. Citation2011). PCOS is characterized by amenorrhea or oligomenorrhoea, ovulatory dysfunction, hyper-androgenism and polycystic ovaries (Ehrmann Citation2005). Women with PCOS are more insulin resistant than matched controls (Ehrmann et al. Citation1999) and have an increased risk of obesity, hirsutism, cardiovascular disease, type 2 diabetes, GDM, obstetric complications and infertility (Unfer et al. Citation2017). The role of inositol in PCOS has been reviewed by others (Unfer et al. Citation2017; Carlomagno, Unfer, and Roseff Citation2011; Nestler and Unfer Citation2015; Regidor et al. Citation2018), so this review will focus on how inositol may influence insulin sensitivity and glucose and lipid metabolism in PCOS.
Treatment of PCOS with inositol supplements
Myo-inositol and D-chiro-inositol supplementation separately and in combination have been shown to improve insulin sensitivity, glycemia and androgen status, circulating lipid profiles, blood pressure, and anthropometry in PCOS (Unfer et al. Citation2012; Facchinetti et al. Citation2015; Unfer et al. Citation2017; Pundir et al. Citation2018; Formuso, Stracquadanio, and Ciotta Citation2015; Cianci et al. Citation2015; Laganà, Barbaro, and Pizzo Citation2015; Nestler et al. Citation1999; Iuorno et al. Citation2002).
A meta-analysis of nine RCTs involving 496 women with PCOS (Unfer et al. Citation2017) showed that inositol treatment (myo-inositol or chiro-inositol) significantly decreased fasting insulin (Standardized Mean Difference (SMD) −1.021 µU/mL, 95% CI: −1.791 to −0.251, P = 0.009) and HOMA-IR (−0.585, −1.145 to −0.025, P = 0.041). Interestingly, in obese PCOS patients, myo-inositol administration appeared more effective in reducing insulin resistance (area under the curve of OGTT) in those with higher fasting plasma insulin levels, suggesting that inositol treatment may be most effective in those who are more insulin resistant (Genazzani et al. Citation2012).
Meanwhile, a separate meta-analysis of ten RCTs involving 362 women with PCOS showed that inositol supplementation (myo-inositol or D-chiro-inositol) was associated with significantly improved ovulation rate and increased frequency of menstruation compared with placebo (Pundir et al. Citation2018). Favorable effects of myo-inositol on ovaries and gametes could also potentially improve assisted reproduction technology outcomes (Nestler and Unfer Citation2015; Bevilacqua et al. Citation2015) and supplementation reduced the likelihood of PCOS mothers developing GDM in one small study (D’anna et al. Citation2012). Some studies have also suggested that combined D-chiro-inositol and myo-inositol treatment may be more effective than myo-inositol alone (Colazingari et al. Citation2013; Nordio and Proietti Citation2012). However, more definitive studies are needed before clinical recommendations can be made.
Inositol dysregulation in polycystic ovary syndrome
In PCOS, urinary D-chiro-inositol was inversely correlated with insulin sensitivity (assessed using multiple methods) and was found to be a strong independent predictor of peripheral insulin resistance (Baillargeon et al. Citation2006; Baillargeon et al. Citation2010). However, this association with insulin resistance is unlikely to be unique to PCOS, since in a study on Greek women, urinary D-chiro-inositol was found to be correlated with hyperinsulinemia, even after correction for BMI and waist-to-hip ratio regardless of PCOS status (Baillargeon et al. Citation2008). It is not yet clear whether plasma and urinary inositols deviate from normal in PCOS. The same study on Greek women, showed no significant differences in plasma and urinary D-chiro-inositol or myo-inositol between PCOS patients and controls (Baillargeon et al. Citation2008). Meanwhile, a separate study on American women by the same group, showed that obese PCOS patients had higher urinary concentrations and lower plasma concentrations of D-chiro-inositol compared with non-obese non-PCOS women, while myo-inositol does not appear altered (Baillargeon et al. Citation2006). However, these changes in inositol content were not significant when adjusted for BMI and/or insulin sensitivity again suggesting that they may be related to differences in BMI or insulin sensitivity rather than PCOS itself. Follicular fluid and serum concentrations of myo-inositol were correlated with oocyte quality and ovulatory activity in women undergoing IVF suggesting that myo-inositol deficiency could lead to impaired fertility (Chiu et al. Citation2002). Overall, these findings suggest that inositol dysregulation in PCOS could be involved in the insulin resistance and infertility issues associated with the syndrome. However, further work is required to untangle how inositol is linked with PCOS, insulin resistance and BMI.
Inositol and IPG regulation in PCOS
The precise mechanisms of inositol involvement in regulating insulin sensitivity in PCOS remains poorly understood. In non-PCOS women, glucose consumption is associated with a spike in circulating P-IPG thought to be caused by an insulin-induced cleavage of GPIs (Baillargeon et al. Citation2006). Several studies have shown that patients with PCOS show reduced glucose-stimulated release of P-IPG into the circulation and that this reduction has been correlated with decreased insulin sensitivity (Cheang et al. Citation2008; Baillargeon et al. Citation2006; Baillargeon et al. Citation2010; Baillargeon et al. Citation2008). Since P-IPG acts as an insulin second messenger and insulin mimic, decreased P-IPG release likely contributes to insulin resistance and obesity in these women. However, these studies do not adequately control for variance in obesity status and further research is necessary to draw conclusions. Interestingly, although metformin therapy decreased post-prandial serum insulin concentrations in obese women with PCOS, glycemia-induced release of P-IPG was not altered (Baillargeon et al. Citation2004). This finding may suggest that P-IPG secretion is regulated by factors that do not overlap with those affected by metformin and raises the prospect of synergism in metformin and inositol combination therapy in PCOS.
It was initially hypothesized that the increased urinary excretion of D-chiro-inositol led to reduced systemic D-chiro-inositol and D-chiro-inositol containing P-IPG (Nestler et al. Citation1999). If this was true it would suggest oral D-chiro-inositol supplementation could restore reduced D-chiro-inositol-containing P-IPGs availability in women with PCOS and improve some clinical features (Nestler et al. Citation1999). However, D-chiro-inositol supplementation in women with PCOS did not increase glucose-stimulated release of P-IPG (Cheang et al. Citation2008). Furthermore, there is no evidence that increased urinary D-chiro-inositol excretion results in systemic D-chiro-inositol deficiency, or that D-chiro-inositol availability is the limiting factor in P-IPG production.
At the local tissue level, ovarian thecal cells from women with PCOS show increased chiro-inositol:myo-inositol ratios and increased epimerase activity compared to controls, suggesting that in PCOS an overactive ovarian epimerase converts more myo-inositol into D-chiro-inositol (Heimark, McAllister, and Larner Citation2014). Increased D-chiro-inositol excretion in PCOS could therefore be due to increased ovarian synthesis and such possibilities need to be investigated to ensure that supplementation studies do not worsen a possible D-chiro-inositol oversupply.
Future studies also need to consider the effects of D-chiro-inositol supplementation on pinitol containing IPGs. Treatment with INS-2, a pinitol containing P-IPG (Larner, Brautigan, and Thorner Citation2010; Larner et al. Citation2003), increased testosterone synthesis in PCOS thecal cells, suggesting that an increase in pinitol based P-IPGs could contribute to the hyperandrogenism seen in PCOS (Nestler et al. Citation1998). However, although pinitol is synthesized from D-Chiro-inositol in plants (Vernon and Bohnert Citation1992), there is not yet evidence that this occurs in animals (Lin, Ma, Gopalan, et al. Citation2009). It is therefore not yet known whether an increase in D-chiroinositol caused by supplementation or increased epimerase activity could increase pinitol containing P-IPGs.
Inositols and their derivatives clearly play important roles in various aspects of the pathophysiology of PCOS, but further research is necessary to disentangle these details. In particular, most studies did not examine the effects of BMI and a lack of suitable controls means it is unclear whether effects are due to obesity, insulin resistance or PCOS.
Inositol in pregnancy
Pregnancy is associated with increasing maternal insulin resistance as gestation progresses, reducing uptake of glucose and lipids by maternal tissues and mobilizing resources to promote fetal nutritional supply, especially in the latter half of pregnancy (Napso et al. Citation2018). To drive such maternal adaptation to pregnancy, pro-diabetic biological signals including hormones, adipocytokines and exosomes are released into the maternal circulation from the placenta, with some originating from the fetus (Scioscia Citation2017; Kunjara, Greenbaum, et al. Citation2000; Jayabalan et al. Citation2017). Our understanding of these processes remains incomplete, but inositol appears to play an important role (Scioscia Citation2017; Kunjara, Greenbaum, et al. Citation2000; Jayabalan et al. Citation2017).
Maternal inositols in an uncomplicated pregnancy and lactation
To date, we know very little about how maternal inositols change during gestation and after delivery. One older study reported that the maternal serum concentration of myo-inositol remains stable across gestation and is similar to that in non-pregnant women, at around 21–26 mmol/L (Quirk and Bleasdale Citation1983). However, this study would not have captured all the different derivatives of inositol nor all inositol stereoisomers in the circulation. In urine, there is a two-fold increase in P-IPGs in the third trimester of uncomplicated pregnancies compared with non-pregnant controls (Kunjara, Greenbaum, et al. Citation2000), and at least one of these P-IPGs is unique to the pregnant state (Paine et al. Citation2003). A cross-sectional study showed that urinary P-IPGs rise between the 18th and 42nd week of pregnancy (Scioscia, Gumaa, et al. Citation2007), suggesting that IPGs could play a role in mediating the changes in glucose and lipid metabolism that occur over this time period (Scioscia, Gumaa, et al. Citation2007). Moreover, urinary P-IPG levels are highest prior to delivery and are significantly higher during labor than in non-laboring women, suggesting that P-IPGs may also be associated with labor onset and progress (Paine et al. Citation2003).
Unfortunately, IPG research in pregnancy is hindered by limitations in analytical techniques for measuring different IPGs. Currently, IPGs can be quantified by separating the A-IPG and P-IPG fractions and testing fractions for enzymatic activity or for inositol content (Paine et al. Citation2006), but this is laborious, nonspecific and will only quantify some and not all IPGs. There is an antibody-mediated P-IPG assay that is specific for one pregnancy-specific P-IPG, but it works for urine samples and doesn’t work well for plasma or serum samples (Paine et al. Citation2003).
Human breast milk contains a very large amount of un-derivatized inositol (), but this falls over the first 6 weeks after birth (Pereira et al. Citation1990). However, whether this decrease reflects declining maternal inositol after pregnancy, or decreasing infant need for inositol is not known.
Thus, overall, the changes in maternal inositol biology across gestation and after delivery remain poorly characterized.
Placental and fetal inositols in pregnancy
Placental inositol
The amount of measured inositol in human placental tissue is influenced by the method of extraction, sample processing and the assay method. By gas chromatography, the human placenta was found to contain 0.53 mmol/kg of un-derivatized inositol (Toh et al. Citation1987) and by enzymatic assay, to contain 2.7 mmol/kg of un-derivatized and 3.5 mmol/kg total acid hydrolyzable inositol, which includes all un-derivatized and derivatized forms (Islam, Selvam, et al. Citation2019) (). These amounts are similar to those reported in rat placenta (0.7–1.7 mmol/kg (Battaglia et al. Citation1961)) and rat liver (0.57 mmol/kg (Palmano, Whiting, and Hawthorne Citation1977)), but much lower than rat brain or kidney () (Palmano, Whiting, and Hawthorne Citation1977). Little is known about the types or proportions of inositol isomers in placenta, but current studies suggest myo-inositol is the predominant form (Islam, Selvam, et al. Citation2019).
Fetal inositol
Substantial synthesis and metabolism of myo-inositol occurs in fetal tissues suggesting an important role for inositol in fetal development. In sheep and goats, fetal circulating inositol is substantially higher than that of adults (), whilst fetal CNS, kidney and skeletal muscle inositol levels are at the upper end of corresponding adult tissues, whereas fetal liver and heart inositol levels are generally lower than in adults ().
The human fetal lung and liver also showed high IMPA1 activity (myo-inositol synthesis) (Quirk and Bleasdale Citation1983) and in rabbit fetal kidney both ISYNA1 (myo-inositol synthesis) and MIOX (myo-inositol catabolism) were highly expressed (Bry and Hallman Citation1991). This is consistent with the possibility of important roles for inositol in fetal development. Relatively high inositol concentrations were also found in human second trimester amniotic fluid (79.0 µM, GCMS), suggesting that the fetal kidney can excrete inositol (Santamaria, Di Benedetto, et al. Citation2016). The presence of inositol synthesis, metabolism and excretion indicates that the fetus can to some extent regulate fetal inositol availability. The inositol content of cranial and spinal neuroectodermal tissue in rat embryos was found to double between day 11 and 12, but little else is known about how fetal tissue inositol is altered or regulated as gestation progresses (Sussman and Matschinsky Citation1988).
Inositol transfer and transport
There was a consistently higher inositol concentration reported in term umbilical artery blood (75 µM (HPLC) (Brusati et al. Citation2005)) that flows from the fetus to the placenta, compared with term umbilical venous blood (64 µM), which flows from the placenta back to the fetus (Toh et al. Citation1987; Santamaria, Di Benedetto, et al. Citation2016; Campling and Nixon Citation1954), which is highly suggestive of fetal to placental transfer of inositol.
Moreover, a stable isotope transport study, involving the maternal administration of 2H6-inositol 2 hours prior to cesarean delivery, indicated that only a small fraction (10% or less) of fetal circulating myo-inositol was derived from transplacental maternal supply in late pregnancy (Staat et al. Citation2012). Since, the concentration of inositol in both venal and arterial cord blood is much higher than maternal blood (26 µM (Brusati et al. Citation2005)), this suggests that the fetus synthesizes substantial amounts of inositol and that there is net fetal inositol export to the placenta.
Inositol concentrations in umbilical cord blood obtained by cordocentesis at mid-gestation (125 µM (Quirk and Bleasdale Citation1983)) and from neonates born preterm (108 µM), was significantly higher than at term (86 µM) (Pereira et al. Citation1990) suggesting a decline in fetal circulating inositol with advancing gestation. In rabbits and kittens circulating inositol falls rapidly in the first month post-delivery reaching equilibrium after 28 days (Campling and Nixon Citation1954). Taken together, these studies suggests that the fetus may export declining amounts of inositol to the placenta with advancing gestation. It is not known whether this decline is due to inositol being more important for fetal-placental development early in pregnancy or whether this decrease acts as an important regulatory signal in maternal-placental-fetal communication.
Inositol transporters SMIT-1 (Berry et al. Citation1995), SMIT-2 (Roll et al. Citation2002) and the SMIT signal transcription factor TonEBP are highly expressed in the placenta (Shin et al. Citation2012; Trama et al. Citation2000), suggesting placental inositol transport between the maternal-placental-fetal compartments is important. Na+ concentrations are generally higher outside the cell than inside the cell due to the action of the Na/K-ATPase, so SMIT (a sodium-inositol symporter) likely transports inositol into the placental syncytiotrophoblast cell barrier from both maternal and fetal compartments (Schneider Citation2015; Johansson, Jansson, and Powell Citation2000). The proton gradient between the maternal-placental-fetal compartments is not constant throughout gestation, and in the presence of other inositol efflux transporters, the net directionality of inositol transport through HMIT (a proton-inositol symporter) remains unclear (Schneider Citation2015; Strange et al. Citation1994; Isaacks et al. Citation1999; Jackson and Strange Citation1993Ganapathy et al., 2000). Overall, it would appear that the placenta is able to both synthesize and import inositol, suggesting an important role for inositol in placental function (see section “Placental and fetal inositols in pregnancy”). Interestingly, SMIT mRNA expression was very high in the brains of rat embryos, but was gradually down-regulated nearer to birth and after birth suggesting that myo-inositol and its transporter play an important role in early CNS development (Guo et al. Citation1997). In contrast, SMIT mRNA expression in kidney was very weak in the embryonic stages, but increased significantly after birth in the rat (Guo et al. Citation1997), a period of development that is equivalent to the in-utero development occurring in the third trimester of human pregnancy. This suggests that myo-inositol plays a key role in the developing fetus, particularly the CNS and kidneys, but that role might change as gestation progresses.
Inositol and fetal development
Inositol appears critical for healthy fetal development. Myo-inositol levels were increased in the urine of both large-for-gestational-age (LGA; birthweight above 90th percentile) and small-for-gestational-age (SGA; birthweight below 10th percentile) human newborns (Marincola et al. Citation2015; Barberini et al. Citation2014) and increased in intrauterine growth restricted (IUGR) piglets (Nissen et al. Citation2011), which suggests that inositol dysregulation is associated with both extremes of the fetal growth spectrum. However, the mechanistic pathways linking LGA and SGA with inositol dysregulation remains speculative. Excessive urinary inositol excretion could be a sign of inositol over production in the fetus, or conversely, reflect a pathological inositol depletion through increased excretion. Since inositols are important regulators of glucose and lipid metabolism, one could speculate that fetal modulation of its own endogenous inositol production and bio-availability could therefore be used as a signaling mechanism to tailor nutritional access from the maternal and placental compartments. In cases of LGA and SGA such inositol-mediated mechanisms are postulated to either be malfunctioning leading to pathology, or used as a compensatory mechanism to try and optimize nutritional supply in the presence of pathology, such as placental insufficiency or hyperglycemia.
For example, we postulate that alterations in inositol signals to the placenta may regulate transplacental supply of fatty acids and lipids for growth. Myo-inositol treatment of human placental explants in-vitro alters the processing of fatty acids into lipids, with myo-inositol displaying fatty acid-specific effects (Watkins et al. Citation2019). In particular, myo-inositol increased the incorporation of isotope-labeled oleic acid into triacylglycerols, phosphatidylcholines, phosphatidylethanolamines and decreased the incorporation of docosahexaenoic acid (DHA) into triacylglycerols in placental explants (Watkins et al. Citation2019). This is of importance, because the amount of each fatty acid available for fetal supply will depend on the balance between the different lipid forms incorporating the fatty acid; more ready supply if the fatty acid is found in transportable forms (e.g. non-esterified and lysophospholipid), or in labile and accessible storage forms (e.g. glycolipids), but less fetal supply if in longer term storage (e.g. structural phospholipids). Since placental lipid processing is key to regulating transplacental fatty acid fetal supply (Lewis, Wadsack, and Desoye Citation2018), myo-inositol actions in placenta likely have a significant impact on fetal growth and development.
Inositol may also affect fetal growth through the facilitation of IGF-1 and IGF-2 signaling, which utilizes PI/PIP/inositol phosphate signaling pathways (Siddle Citation2011). Maternal and fetal circulating IGF-1 and IGF-2 are strongly associated with the promotion of placental nutrient transport and fetal size (Jansson et al. Citation2013; Baumann et al. Citation2014). Increases in birthweight occur with increasing glucose and amino acid transport to the fetus, and this is likely mediated by IGF-1, since IGF-1 treatment was associated with increased placental glucose and amino acid transporter (GLUT1, SNAT2) expression in multiple placental in-vitro models (Jansson et al. Citation2013; Baumann et al. Citation2014). However, IGF also decreased lipoprotein lipase activity in placental villous explants in-vitro (Magnusson-Olsson et al. Citation2006), which could lead to decreased maternal to fetal fatty acid transfer if the same occurred in-vivo. Dysregulation in inositol content or metabolism would be expected to alter IGF signaling and could therefore have significant impact on fetal growth, with the direction of growth-change dependent on which nutritional supply pathways are most impacted.
Inositol derivatives also affect the placental secretion of hormones that modulate fetal growth. In particular, inositol phosphates are involved in inducing the release from placental syncytiotrophoblasts of human placental lactogen (hPL; see section “Placental phosphoinositides”), which has anti-insulin properties (Zeitler and Handwerger Citation1985; Zeitler, Wu, and Handwerger Citation1991; Zeitler, Markoff, and Handwerger Citation1983; Handwerger and Freemark Citation2000) and is one of the key hormones involved in maternal adaptation to pregnancy. As previously discussed (section “Inositols influence lipid metabolism, transport and storage”), both A-IPG and myo-inositol appear to decrease adipocyte leptin release in non-pregnant in-vitro and in-vivo rat models (Croze, Géloën, and Soulage Citation2015; Kunjara, Greenbaum, et al. Citation2000). If a similar effect of reduced leptin release from the placenta and adipose tissue occurs in pregnancy, it could potentially suppress mobilization of glucose, amino acids and lipids to the fetus for growth (Tessier, Ferraro, and Gruslin Citation2013). Inositol also appears to be important for CNS development. Maternal circulatory myo-inositol in the lowest decile has also been associated with spina bifida in humans (Groenen, Merkus, et al. Citation2003), although no association was seen with amniotic fluid inositol levels (Groenen, Merkus, et al. Citation2003). There are also several studies reporting associations between myo-inositol deficiency and dysmorphogenesis in rodents (Cogram et al. Citation2002; Steegers-Theunissen, Groenen, and Beemster Citation2002; Copp and Greene Citation2010; Baker et al. Citation1990) which will be discussed in detail in section “Placental phosphoinositides.”
Hypothesis: Inositol acts as a communication signal across the maternal-placental-fetal axis to regulate fetal growth and development
We postulate that levels of inositol in pregnancy, whether of maternal, fetal or placental origin, act as biological signals that can regulate maternal and placental glucose and lipid metabolism, endocrine activity and fetal nutrient supply. As a result, inositol and its derivatives are able to regulate fetal growth and development. Details of the direction of inositol exchange between the maternal, placental and fetal compartments and the main foci of inositol action in maternal and fetal tissues, and whether maternal inositol supplementation can address defects in these physiological mechanisms remain to be established. How defects in these processes may lead to macrosomia in GDM is addressed in section “Dysregulated maternal-placental-fetal lipid metabolism, adiposity and fetal growth in gestational diabetes” and in .
Figure 4. Hypothesis: Inositol, acts as a communication signal across the maternal-placental-fetal axis to regulate maternal glycemia and lipidemia, placental function and fetal physiology and therefore fetal growth and development. We also suggest that much of this inositol is of fetal origin and is likely regulated by factors such as fetal growth potential and growth velocity, forming a feedback loop and enabling the fetus to control its nutritional supply according to its needs, to regulate its own size/growth and development. However, inositol regulation becomes dysfunctional in pregnancies complicated by disorders such as GDM leading to disorders of fetal growth and maternal insulin resistance. *1 Increased excretion into the amniotic fluid decreases fetal inositol. *2 Increased excretion into maternal urine decreases maternal inositol. *3 Placental inositol impacts placental function. In particular, inositol and inositol derivatives alter the balance of placental nutrient import, metabolism, storage and export and therefore fetal nutrient supply. Inositol derivatives also affect placental signaling since they act as second messengers for hormones such as insulin and insulin like growth factor (IGF) and impact the synthesis and secretion of bioactive lipid mediators and hormones such as human placental lactogen. *4 Inositol regulates maternal lipidemia by altering the balance of lipid mobilization between different types of adipose tissue, but the direction of this inter-adipose-depot redistribution appears strongly affected by BMI. *5 Inositol and its derivatives can regulate maternal glycemia directly or by affecting maternal insulin sensitivity.
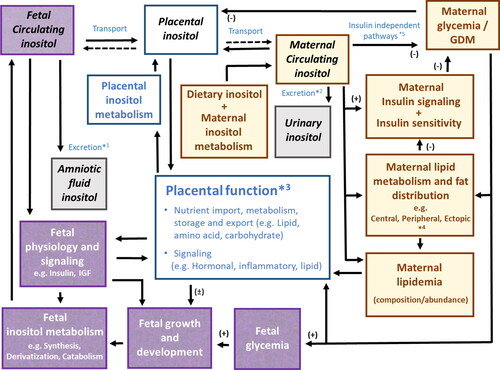
Placental and fetal IPGs
Healthy human placenta contains more P-IPG than any other studied organ (25-fold more than liver), but contains no or little detectable A-IPG (Scioscia Citation2017; Scioscia et al. Citation2013; Kunjara, Greenbaum, et al. Citation2000). P-IPG holds a critical role in the placenta and is involved in the regulation of glycogen, glucose and lipid metabolic pathways, which in other organs are mainly regulated by insulin (Larner, Brautigan, and Thorner Citation2010; Kunjara et al. Citation1999; Scioscia Citation2017). Uniquely, the placenta is not reliant on insulin for glucose uptake, a feature that preserves continuous rapid maternal-fetal transfer of glucose, which is essential for fetal survival (Lager and Powell Citation2012). The role of IPGs in facilitating anaerobic glycolysis is probably particularly important early in gestation, to provide an alternative to aerobic glucose oxidation in a low oxygen environment prior to deep trophoblast invasion, which begins at the end of the first trimester, and prior to development of an effective hemochorial placenta for optimal oxygen and nutrient transfer to the fetus (Scioscia et al. Citation2011). Later on in pregnancy, P-IPGs may play an important physiological role in the regulation of insulin resistance in order to maintain optimal fetal nutritional supply (Larner Citation2002; Kunjara, Greenbaum, et al. Citation2000; Scioscia, Gumaa, and Rademacher Citation2009). Placental P-IPG could alter placental function locally, or be released into the maternal or fetal circulation where it could alter maternal or fetal metabolism (Scioscia et al. Citation2008; , Schofield, and Rademacher Citation2003).
Even though placental P-IPGs can regulate placental functions without insulin involvement, insulin may still promote placental P-IPG actions by effecting P-IPG synthesis and transport. For example, in-vitro studies showed that insulin stimulation caused more P-IPGs to be released by maternal-facing syncytiotrophoblast microvillous membrane isolates (Scioscia et al. Citation2008). This increase in P-IPG is thought to be due to the insulin induced activation of placental GPI-specific phospholipase D (GPI-PLD) which cleaves IPG from precursor GPIs (Scioscia et al. Citation2008; Deborde, Schofield, and Rademacher Citation2003).
However, IPG synthesis will also be affected by the availability of GPI precursors and GPI-PLD and nothing is known about how this may be affected by insulin.
Even though placental syncytiotrophoblast microvillous membranes contain active GPI-PLD enzyme (Deborde, Schofield, and Rademacher Citation2003), no GPI-PLD mRNA has been found in BeWo cells (trophoblast-like cell line) or in two placental cDNA libraries (Deborde, Schofield, and Rademacher Citation2003). This suggests GPI-PLD is not synthesized by the placenta and is instead of maternal or fetal origin (Deborde, Schofield, and Rademacher Citation2003). Large amounts of GPI-PLD are exported into the circulation by the liver outside of pregnancy, suggesting that placental GPI cleavage maybe regulated by the maternal or fetal liver (Low Citation2000).
We do not know if placental GPI is of maternal, placental or fetal origin. However, Deborde et al. has suggested that the structure of placental syncytiotrophoblast may regulate how easily GPI-PLD can access GPIs stored in caveolae membrane invaginations and that this may alter IPG availability in various pregnancy complications (Deborde, Schofield, and Rademacher Citation2003; Parpal, Gustavsson, and Strålfors Citation1995; Anderson et al. Citation1992).
Although some IPGs appear to be synthesized by the placenta, one pregnancy-specific P-IPG which can be detected by an antibody assay appears to be formed by the fetus. In uncomplicated pregnancies, this pregnancy-specific P-IPG was found to be several hundred times more concentrated in amniotic fluid than in maternal urine (Scioscia Citation2017; Paine et al. Citation2006; Scioscia et al. Citation2011). Furthermore in placenta, this pregnancy-specific P-IPG was predominantly localized in the syncytiotrophoblast, endothelium of placental vessels and extravillous trophoblast in the basal plate, with only a little immunostaining within the villous stroma (Scioscia et al. Citation2013). If this pregnancy specific P-IPG is of fetal origin, it could potentially act as a signal to the mother by which the fetus can regulate its own nutritional supply according to its needs. However, evidence for this postulation is still scarce and needs to be explored further. Interestingly amniotic fluid P-IPG was decreased in the third trimester compared to earlier in pregnancy (Scioscia, Gumaa, et al. Citation2007) possibly suggesting that this P-IPG is more important early in pregnancy, or that a decrease in this P-IPG is an important signal in late pregnancy.
Placental phosphoinositides
Phosphoinositide (PI) lipids act as important signaling molecules in the placenta and are a major store of essential polyunsaturated fatty acids (PUFA) within the fetal-placental unit (Bayon et al. Citation1993). A placental phosphatidylinositol synthase (CDIPT) has previously been isolated and characterized, suggesting that the placenta can synthesize phosphoinositide lipids (Antonsson Citation1994). Arachidonic acid (AA, an omega-6 fatty acid; 20:4) for example, comprises 33% of the fatty acids held in placental PI (Bitsanis et al. Citation2005). Such storage reservoirs are important, because the fetal-placental unit cannot synthesize PUFA due to a lack of desaturase enzymes (Bayon et al. Citation1993). PUFAs can be released from placental phospholipids by phospholipase A (PLA) (Schrey et al. Citation1992; Schrey, Read, and Steer Citation1987; Petit et al. Citation1989), a process vital for fetal PUFA supply and brain development (Crawford Citation2000), and for the synthesis of many PUFA-derived signaling molecules essential for fetal and placental development and labor such as prostaglandins and leukotrienes (Holub Citation1986; Walsh and Parisi Citation1986; Boone, Currie, and Leung Citation1993).
Inositol and arachidonic acid in dysmorphogenesis
Myo-inositol’s role in preventing fetal anomalies appears to be partially mediated via its effect on AA metabolism and prostaglandin synthesis. It is well-established that dysmorphogenesis, particularly fetal neural tube defects, are associated with poorly controlled preexisting diabetes in humans (Wentzel and Eriksson Citation2020). Similar defects are also observed in streptozocin-induced diabetic pregnant rat and mouse embryos incubated with excess glucose (Wentzel et al. Citation2001; Sussman and Matschinsky Citation1988; Baker et al. Citation1990; Hashimoto et al. Citation1990). Such embryos also displayed an associated decrease in inositol content, particularly in the neuroectoderm (Steegers-Theunissen, Groenen, and Beemster Citation2002; Wentzel et al. Citation2001; Sussman and Matschinsky Citation1988; Baker et al. Citation1990; Hashimoto et al. Citation1990), and indeed, the addition of either prostaglandin E2 or myo-inositol prevented the neural tube defects (Zeitler, Wu, and Handwerger Citation1991; Baker et al. Citation1990). Separately, in the non-diabetic curly tail mice model, D-chiro-inositol and myo-inositol supplementation in-vitro was found to reduce the frequency of spina bifida (Cogram et al. Citation2002).
The protective effect of myo-inositol was reversed in-vivo by the addition of compounds that inhibited the synthesis of prostaglandins from AA (Baker et al. Citation1990). This suggests that inositol plays an important role by providing adequate AA for prostaglandin synthesis necessary for healthy CNS development although the nature of this role is not known (Cogram et al. Citation2002; Baker et al. Citation1990).
Inositol might increase the availability of AA by acting as a structural base for greater AA storage in the form of PI. Alternatively, inositol might influence the metabolism and release of AA from a range of phospholipids through regulating lipid synthesis and lysis. For example, cleavage of inositol containing PIP2 stimulates protein kinase C and activates PLA2, the phospholipase that cleaves PUFA from phospholipids (Wentzel et al. Citation2001). Inositol could also affect the downstream synthesis of prostaglandins. However, further studies are needed to confirm the inositol-related mechanisms on AA release and prostaglandin synthesis.
Rat embryos incubated in high glucose concentrations and rat embryos from mothers with streptozocin-induced diabetes showed decreased expression of cytosolic PLA2 (Wentzel et al. Citation2001) and cyclooxygenase COX-2 (the enzyme catalyzing the synthesis of prostaglandins from AA; (Wentzel, Welsh, and Eriksson Citation1999)) compared with respective controls on embryonic day E10 but not E11 of gestation. This suggests a narrow gestational window for phospholipid cleavage, AA release and prostaglandin synthesis to impact CNS development. However, this change has yet to be linked to inositol. If it is, then the timing of inositol supplementation in pregnancy to prevent defects would be critical.
Interestingly, incubation of mouse embryos with scyllo-inositol caused similar defects as incubation with glucose, which suggests that scyllo-inositol competes as a ligand with myo-inositol in early development to disrupt the important role played by myo-inositol (Steegers-Theunissen, Groenen, and Beemster Citation2002; Wentzel et al. Citation2001; Baker et al. Citation1990).
Interactions of inositol derivatives and lipids in maternal-placental-fetal physiology
The inter-relationships between placental inositol derivatives, AA availability, maternal endocrine regulation by the placenta and fetal growth are complex. In-vitro AA treatment in various models of placental explants, dispersed placental cells and cultured term human syncytiotrophoblast, stimulated the hydrolysis of PIPs by phospholipase C (Zeitler and Handwerger Citation1985; Zeitler, Wu, and Handwerger Citation1991; Zeitler, Markoff, and Handwerger Citation1983Petit et al., 1989). This caused the release of placental inositol phosphates, which then induced a rapid release of human placental lactogen (hPL) (Zeitler and Handwerger Citation1985; Zeitler, Wu, and Handwerger Citation1991; Zeitler, Markoff, and Handwerger Citation1983Petit et al., 1989). This process is important especially in the third trimester of pregnancy, because hPL changes the metabolic state of the mother to facilitate mobilization of glucose and fatty acids for fetal nutrition (Zeitler, Wu, and Handwerger Citation1991; Handwerger and Freemark Citation2000). Inositol phosphates are also crucial in regulating placental lipid metabolism. For example, PIP2 activates placental phospholipase D, which hydrolyzes phospholipids to produce phosphatidic acid, a bioactive lipid which modulates kinase signaling (Vinggaard and Hansen Citation1995; Liscovitch et al. Citation2000; Wang et al. Citation2006).
Thus, in pregnancy, inositols, PI lipids, PIPs and inositol phosphates play critical roles in regulating paracrine, endocrine, intracellular and intercellular signaling, to influence maternal and fetal physiology, and fetal nutritional provision. Given the diversity of inositol involvement in various biological pathways of maternal-placental-fetal physiology, some form of inositol dysregulation is likely involved in the pathophysiological processes of pregnancy complications, and conversely, great potential for inositol-related interventions to aid in preventing or managing these complications.
Gestational diabetes
Gestational diabetes (GDM) is glucose intolerance first recognized during pregnancy, which is associated with increased risk of short term and long term adverse outcomes for both mother and child (Sacks et al. Citation2012). Both hyperglycemia and lipid dysregulation are postulated to be involved in its pathophysiology (Gallo, Barrett, and Nitert Citation2017). GDM is associated with increased risk of hypertensive disorders of pregnancy, preterm delivery and cesarean section delivery and babies are at risk of numerous negative consequences including neonatal hypoglycemia, jaundice and perinatal death (Metzger et al. Citation2008; Crowther et al. Citation2005; Mitanchez et al. Citation2015). GDM is also associated with fetal macrosomia which is linked with increased risk of shoulder dystocia and birth trauma (Metzger et al. Citation2008; Crowther et al. Citation2005; Mitanchez et al. Citation2015). In the longer term offspring are at an increased risk of major chronic health conditions including obesity, type 2 diabetes, metabolic syndrome and cardiovascular disease later in life, while women who had GDM are 7 times more likely to later develop type 2 diabetes than controls (Mitanchez et al. Citation2015; Bellamy et al. Citation2009; Ruchat, Hivert, and Bouchard Citation2013; Ma et al. Citation2015).
Pregnancy is a pro-diabetogenic state and is associated with rising maternal insulin resistance, increased maternal glucose intolerance and an elevation of circulating non-esterified fatty acids, most prominently in the third trimester (Scioscia Citation2017; Kunjara, Greenbaum, et al. Citation2000; Jayabalan et al. Citation2017). These changes are essential for providing the fetus with adequate nutrients, but individual hereditary and environmental vulnerabilities can lead to accentuated changes that result in GDM and related complications in some women. It has been postulated that women who are already sub-clinically metabolically challenged preconception may be unable to mount an adequate insulin response in pregnancy, resulting in GDM (Catalano et al. Citation2003). Since inositols play an influential role in pregnancy and in diabetes, it is important to understand the interactions between GDM and inositol metabolism and action, in order to fully exploit inositol’s potential as an intervention in this context.
Relationship between gestational diabetes and inositol
Alterations in the level of inositols in biological fluids are apparent many weeks prior to the clinical detection of hyperglycemia and GDM diagnosis (Murphy et al. Citation2016). When assessed during early pregnancy, women who would subsequently manifest the signs of GDM later in pregnancy displayed increased first trimester urinary D-chiro-inositol and myo-inositol (Murphy et al. Citation2016) and increased second trimester amniotic fluid myo-inositol (Santamaria, Di Benedetto, et al. Citation2016), compared to women who did not subsequently develop GDM. Such findings suggest that dysregulated inositol metabolism and excretion in the mother or the fetal-placental unit predates the development of hyperglycemia, and may be involved in the generation of pathological events leading to GDM development. Since similar increases in urinary D-chiro-inositol and myo-inositol were also observed in non-pregnant overweight and obese diabetic adults (Ostlund et al. Citation1993; Hong et al. Citation2012), and in obese diabetic rodent models (), it has been postulated that a similar pathological pathway of inositol dysregulation may be shared by GDM and adult diabetes that is driven by obesity.
Currently, there is little evidence to suggest how inositol content and actions in the maternal and fetal tissues, are altered in GDM, nor how they may alter the risk of GDM development. Our data suggests that placental inositol content is significantly reduced in GDM pregnancies compared with non-GDM cases (Pillai et al. Citation2020) and work is currently on-going to establish the underlying mechanisms for this difference. However, the concurrent rise of both amniotic fluid and maternal urinary inositol during pregnancy suggests that GDM does not conform to the theory of a general diabetic inositol deficiency caused by increased urinary inositol excretion. More SMIT-1 protein was found in GDM placenta compared to non-GDM placenta, with expression changes localized to the trophoblast suggesting that increased inositol transport into the placenta occur in GDM (Mejia, Reynolds, and Arroyo Citation2016). In contrast, our studies showed a decline in IMPA1 (myo-inositol synthesis), SMIT-2 and HMIT expression, associated with reductions in placental inositol content, with increasing maternal fasting glycemia (Pillai et al. Citation2020). However, many other inositol-related factors are likely altered in GDM, and further work is needed to understand how inositol alterations within the maternal-placental-fetal axis relates to GDM pathophysiology.
P-IPGs in pregnancy and GDM
P-IPGs may play an important physiological role in the regulation of insulin resistance in pregnancy (Larner Citation2002; Kunjara, Greenbaum, et al. Citation2000; Scioscia, Gumaa, and Rademacher Citation2009). Urinary P-IPGs measured by PDH assay were found to physiologically increase with increasing gestation among non-diabetic women (Kunjara, Greenbaum, et al. Citation2000; Scioscia, Gumaa, and Rademacher Citation2009). Since insulin resistance also rises in late pregnancy, and because of the link between urinary IPGs and insulin resistance in the non-pregnant state, it has been suggested that P-IPGs are likely involved in the development of insulin resistance in the pregnant state (Larner Citation2002; Kunjara, Greenbaum, et al. Citation2000; Scioscia, Gumaa, and Rademacher Citation2009).
Urinary P-IPGs in women with preexisting type 2 diabetes and those who subsequently developed GDM, were elevated in the first two trimesters of pregnancy compared with non-diabetic pregnancy (Kunjara, Greenbaum, et al. Citation2000; Scioscia, Gumaa, and Rademacher Citation2009). Furthermore, in one small study of women with GDM, urinary P-IPG levels were found to correlate with antenatal glycemia in the third trimester and with birthweight, but these correlations were not observed in the control group (Scioscia, Gumaa, et al. Citation2007). However, the amount of P-IPG in maternal tissues and plasma in pregnancies complicated by diabetes compared to uncomplicated pregnancies is not known. Increased urinary P-IPG may either reflect an adaptive systematic increase in P-IPG to try to counter insulin resistance, or reflect pathological increased urinary excretion, which could in turn reduce systemic P-IPG and promote insulin resistance.
Even though early pregnancy urinary P-IPGs are higher in diabetic pregnancies, women with preexisting type 2 diabetes and GDM do not show the normal ontogenic rise in urinary P-IPG with advancing gestation (Kunjara, Greenbaum, et al. Citation2000; Scioscia, Gumaa, and Rademacher Citation2009). Therefore, by the late third trimester, urinary P-IPG levels in diabetic and non-diabetic groups are similar () (Kunjara, Greenbaum, et al. Citation2000; Scioscia, Gumaa, and Rademacher Citation2009). This could suggest that GDM pregnancies go through the P-IPG and glycemic-related metabolic adaptations associated with later pregnancy at much earlier gestations. If true, this could increase fetal nutritional supply over a longer period and possibly contribute to excessive fetal growth and adiposity in GDM (Scioscia et al. Citation2011; Scioscia, Gumaa, and Rademacher Citation2009).
A-IPGs in pregnancy and GDM
In the third trimester, urinary A-IPG was found to be lower in GDM than in non-GDM controls and correlated negatively with glycemic control, but positively with BMI (Scioscia, Gumaa, et al. Citation2007). This study also found a trend suggestive of an association between urinary A-IPG and birth weight. Since A-IPG in-vitro influences many glucose, lipid and insulin-related metabolic processes, in particular stimulating the synthesis of lipids and glycogen, while lowering lipolysis (D’Anna and Santamaria Citation2018; D’Oria et al. Citation2017; Malvasi et al. Citation2014; Pintaudi, Di Vieste, and Bonomo Citation2016; D'Anna et al., Citation2013; Matarrelli et al. Citation2013), it is plausible that A-IPG may play a role in the pathophysiology of glucose intolerance and fetal macrosomia. However, in-vivo mechanistic studies are lacking and we cannot exclude the possibility that A-IPG may only be an associated indicator of glucose intolerance. Furthermore, since tissue A-IPG has not yet been quantified in GDM we cannot tell whether low maternal urinary A-IPG reflects low systemic A-IPG or decreased excretion. A-IPGs have been reported to inhibit leptin release from adipocytes, increase lipid synthesis and decrease the oxidative disposal of glucose in a variety of cell types (Kunjara, Greenbaum, et al. Citation2000; Scioscia, Gumaa, et al. Citation2007; Kunjara, Greenbaum, et al. Citation2000). However, the action of A-IPGs in pregnancy remains unknown and it is difficult to predict how these molecules may be involved in GDM.
Ipgs as biomarkers of GDM
Whether urinary or serum inositols or inositol derivatives such as IPGs could represent useful biomarkers to identify in early pregnancy women who are at significant risk of developing GDM later in pregnancy remains to be explored. Examining if inositol biomarkers could improve predictive power on top of that provided by conventional clinical risk assessments using factors such as previous history of GDM, previous fetal macrosomia, high BMI and family history of diabetes. Preventive measures could be instituted early and targeted at those who are most likely to benefit.
Prevention and treatment of gestational diabetes with inositols
Inositol supplementation is a promising intervention for the prevention and treatment of GDM. A recent meta-analysis (Zhang et al. Citation2019) of 5 RCTs (Farren et al. Citation2017; D'Anna et al., Citation2013; Matarrelli et al. Citation2013; DʼAnna et al. Citation2015; Santamaria, Di Benedetto, et al. Citation2016) with a total of 927 pregnant women with metabolic risk factors, found that the daily supplementation of pregnant women with 2 − 4 g myo-inositol from early pregnancy reduced the incidence of GDM (RR 0.43, 95%CI 0.21–0.89), and preterm delivery (RR 0.36, 0.17–0.73). Other meta-analyses of these studies generally agreed with these findings (Crawford et al. Citation2015; Zheng et al. Citation2015; Santamaria et al. Citation2018; Vitagliano et al. Citation2019). These RCTs were mainly conducted in Caucasian women who had risk factors for developing GDM namely obesity/overweight (DʼAnna et al. Citation2015; Santamaria, Di Benedetto, et al. Citation2016), a family history of type 2 diabetes (D'Anna et al., Citation2013), or an impaired fasting glucose in the first trimester (Matarrelli et al. Citation2013), with supplementation with myo-inositol mostly beginning from the late first trimester onwards. In a separate study, myo-inositol treatment starting preconception in women with PCOS (n = 83) was also found to reduce the risk of developing GDM (17.45% vs 54%, odds ratio 2.4, 95% CI, 1.3–4.4) and this difference remained significant even after adjusting for covariates (age, BMI, parity, family history of diabetes) (D’anna et al. Citation2012).
Two studies have looked at the effects of D-chiro-inositol combined with myo-inositol. One small randomized control trial on non-obese Italian pregnant women (n = 157) with elevated fasting glucose in the first or early second trimester, who were supplemented with either myo-inositol (4000 mg), D-chiro-inositol (500 mg), combined myo- and D-chiro-inositol (1100/27.6 mg) or placebo, showed that all the inositol treatments significantly decreased fasting and post-prandial glycemia and reduced the risk of developing GDM (Celentano et al. Citation2020). Non-inferiority analysis demonstrated the largest benefit was found in the myo-inositol group, although dosage differences make comparison difficult (Celentano et al. Citation2020). In contrast, another study in Irish women (n = 240) with a family history of diabetes found that a combination of myo-inositol 1100 mg and D-chiro-inositol 27.6 mg/day did not lower GDM incidence compared to controls (Farren et al. Citation2017).
One meta-analysis (Zhang et al. Citation2019) showed that myo-inositol had no substantial impact on the OGTT 2-h post-glucose load response or on neonatal birthweight and macrosomia risk (birthweight >4 kg). However, the meta-analysis was likely underpowered to demonstrate modulation of fetal size because of the low prevalence of macrosomia (Zhang et al. Citation2019). In contrast, the Santamaria et al. (Citation2018) meta-study which focused only on a subset of three RCTs in an Italian population at risk of GDM (high BMI or family history of type 2 diabetes n = 595), showed that myo-inositol significantly decreased GDM diagnosis, fasting and post-prandial glycemia, preterm birth and macrosomia. The effect of myo-inositol on insulin resistance in these Italian studies appears to be population dependent with HOMA-IR significantly decreased in overweight and obese pregnant women (DʼAnna et al. Citation2015; Santamaria, Di Benedetto, et al. Citation2016), but not those with a family history of type 2 diabetes (D'Anna et al., Citation2013). However, these studies were open label and were all conducted by the same research group on a homogeneous population. Thus, it remains uncertain if findings can be generalized to other populations.
One study also analyzed the effect of myo-inositol treatment on lipids in pregnancy (Malvasi et al. Citation2014). In this small trial (24 treatment, 24 control), the combination of myo-inositol, D-chiro-inositol, folic acid and manganese given to non-obese pregnant women was associated with reduced glycemia, as well as total cholesterol, low-density lipoproteins (LDL), high-density lipoproteins (HDL), and triglycerides compared with control. However, it is unclear whether these changes are due to inositol or to other treatment components.
Despite these promising findings, all reviews to date have concluded there is insufficient data to properly evaluate whether the use of myo-inositol during pregnancy is efficacious in reducing the risk of GDM development. In particular, the populations studied were too small and lacked diversity, and many studies were open-label (unblinded) and thus exposed to bias. Furthermore, there is a lack of data on neonatal outcomes including perinatal mortality and serious infant morbidity and longer-term offspring health. Also, there is insufficient data to determine whether myo-inositol is a better treatment option than D-chiro-inositol or inositol combinations, or whether these molecules have interactive effects (Celentano et al. Citation2020).
Further evaluation in large-scale, multicentre, double-blinded RCTs are necessary to prove the efficacy of inositols in reducing the risk of GDM, macrosomia and preterm delivery. Furthermore, these trials will need to include pregnant women of different ethnicities and more varied risk factors, with examination of different doses of myo-inositol and other inositol isomers in comparison with placebo. An example of such a trial is the NiPPeR study (Godfrey et al. Citation2017) (clinicaltrials.gov NCT02509988) which included women of multiple ethnicities with both low and high metabolic risks from UK, Singapore and New Zealand. The intervention is a nutritional formulation containing myo-inositol, probiotics and additional micronutrients, commenced prior to conception and continued throughout pregnancy, and results are expected to be published later in 2020. There is also another smaller on-going clinical trial in the UK (EMmY study, ISRCTN48872100) (Amaefule et al. Citation2018) of myo-inositol supplementation starting in early pregnancy in women at high risk of GDM development. With completion of these trials a clearer picture will emerge on the efficacy of inositol supplementation in reducing pregnancy risks, the safety of the intervention, and whether specific subgroups would benefit more than others.
Inositol use after GDM diagnosis
Upon the diagnosis of GDM, maternal hyperglycemia can be controlled by a variety of interventions, usually starting with diet control, exercise and lifestyle changes, then adding in therapies such as metformin and/or insulin if required (Falavigna et al. Citation2012; Sweeting et al. Citation2016). Two randomized open-label trials have tested the effect of inositol in women already diagnosed with GDM. One trial on 69 women with GDM found that myo-inositol (4000 mg) improved insulin resistance (lower HOMA-IR) and increased circulating adiponectin (Corrado et al. Citation2011). Another trial (n = 80) tested the effect 4000 mg myo-inositol or 500 mg D-chiro-inositol or 1100/27.6 mg myo/D-chiro-inositol (Fraticelli et al. Citation2018). This study found that insulin resistance was significantly decreased in women given myo-inositol, and that groups on myo-inositol-containing interventions (but not the chiro-inositol alone group) were less likely to require insulin therapy, and delivered newborns with significantly lower birthweights. A third observational study also found that women with GDM treated with 1.2 g myo-inositol were less likely to require insulin treatment later in pregnancy but lacked a contemporaneous control group for valid comparison (Lubin et al. Citation2016). Collectively, these studies suggest that myo-inositol could potentially be considered as first line GDM therapy, or as adjuvant therapy with insulin to reduce insulin dosage and reduce the risk of hypoglycemia associated with this treatment, although this remains to be clinically evaluated.
Dysregulated maternal-placental-fetal lipid metabolism, adiposity and fetal growth in gestational diabetes
According to Pederson, fetal macrosomia is a result of the anabolic and lipogenic effect of fetal hypersecretion of pancreatic insulin, which is stimulated by increased maternal-fetal glucose transfer in maternal diabetes (Pedersen Citation1952). However, GDM cases with apparently satisfactory glycemic control, still show an increased risk of fetal macrosomia, and neonates even if appropriately-grown-for-gestational-age as judged by birthweight, still show increased neonatal adiposity in body composition measures (Poston et al. Citation2015; Zhuo, Wang, and Yu Citation2014). Furthermore, maternal circulating TAGs and free fatty acids, have been positively correlated with neonatal weight and fat mass in well-controlled GDM, but not in non-GDM pregnancies (Schaefer-Graf et al. Citation2008; Schaefer et al. Citation2011). Hence, it has been postulated that dysregulated lipid metabolism across the maternal-placental-fetal axis, on top of glycemia, may contribute to the development of excessive fetal growth and adiposity in GDM (Herrera and Ortega-Senovilla Citation2010). Furthermore, it is already well documented that in GDM there is reduced maternal-placental-fetal transfer of the essential long chain (LC)-PUFAs (Herrera and Ortega-Senovilla Citation2018; Larqué et al. Citation2014; Delhaes et al. Citation2018; I et al. Citation2002; Fraser et al. Citation1990; Schwartz et al. Citation1994) and it is likely that further abnormalities of lipid regulation will be described with on-going research.
Inositol treatment of animal models of GDM appears to alter maternal lipid metabolism, but effects vary depending on the model studied. Myo-inositol treatment in the genetically-modified db/+ mouse model of GDM reduced maternal intra-abdominal, gonadal and perirenal adiposity, but had no apparent effect on fetal size (Plows et al. Citation2017). In contrast, in a high fat diet mouse model of GDM, myo-inositol treatment did not affect maternal glycemia or fat accretion (Plows et al. Citation2020), but did increase maternal adipose tissue mRNA expression of Ir, Irs1, Akt2, and Pck1, which are all key members of the insulin signaling pathway, and demonstrated an associated decrease in offspring birthweight (Plows et al. Citation2020). This suggests that inositol might have a pro-insulin or an insulin-like effect in adipose tissue resulting in decreased maternal lipid mobilization and lipid transfer for fetal growth. Myo-inositol may also alter lipid supply to the fetus by affecting placental lipids metabolism as discussed in section “Inositol and fetal development.”
Current management of GDM does not involve lipid monitoring nor treatment of dyslipidemia, and lipid lowering drugs are generally contraindicated in pregnancy. Myo-inositol and its derivatives, as naturally-occurring compounds, are promising candidates to address this metabolic dysfunction in GDM since they can alter both glucose and lipid metabolism. This venture must firstly involve development of a much deeper understanding of how inositols influence lipid metabolism in maternal tissues and the placenta, and how they may influence maternal-fetal lipid transfer. Then, further clinical studies focusing on lipid and adiposity outcomes in pregnancy are necessary to determine whether myo-inositol or any other inositol isomers or derivatives could be an effective treatment for the anomalous lipidomic features of GDM.
Postulated actions of inositol in gestational diabetes
The mechanisms by which myo-inositol supplementation may reduce the risk of GDM and fetal macrosomia are not understood (Coustan Citation2013). Diabetes is classically hypothesized to be a condition of myo-inositol deficiency (see above 4.5). However, there has been no clear evidence for a general systemic inositol deficiency in GDM women that would require correction (, section “Relationship between gestational diabetes and inositol”). Current findings instead suggest a complex situation where inositol perturbations may be caused by ill-defined alterations in synthesis, transport and metabolism, and may possibly differ between tissue types. Furthermore, these alterations could be either pathological leading to the development of GDM, or be an adaptive response to the GDM condition to minimize its impact. Therefore, there is not yet a full picture of how these various processes would be affected by maternal inositol supplementation and what the net effects would be in different individuals. However, here we have drawn together threads of our current understanding () upon which new knowledge can be added.
Myo-inositol supplementation has been shown to improve insulin resistance and glycemic and lipid status in various metabolic conditions in the non-pregnant state and may, in theory, also act on maternal tissues during pregnancy to improve maternal metabolic health (Croze and Soulage Citation2013; Coustan Citation2013; Zhang et al. Citation2019). However, pregnancy involves not just the mother, but also the placenta and the fetus, and any intervention needs to consider the potential risks and benefits on all three.
Inositol and inositol derivatives are thought to play a role in the action or release of many hormones that affect maternal metabolism including insulin, thyroid stimulating hormone, leptin and human placental lactogen (Van Sande et al. Citation2006; Sayers and Hanyaloglu Citation2018; Berridge Citation2009; Zeitler et al., 1991; Handwerger and Freemark Citation2000; Pérez-Pérez et al. Citation2016; Hauguel-de Mouzon, Lepercq, and Catalano Citation2006). We postulate that alterations in placental inositol content, could alter fetal nutritional supply by releasing endocrine signals that affect maternal metabolism or by directly changing placental glucose, lipid and amino acid metabolism and transport. Observational data from the GUSTO mother-offspring cohort (Chu et al. Citation2020) and from a separate smaller study (Pillai, et al. Citation2020) showed that a high placental inositol content is associated with a suppression of the fetal growth-promoting and pro-adipogenic effects of maternal glycemia.
Whether maternal inositol supplementation can increase placental inositol content to favorably modulate fetal growth and adiposity remains to be established.
If myo-inositol supplementation can lower maternal blood glucose and suppress mobilization of lipids to the fetus, then this could reduce excessive nutrient transfer to the fetus, reducing macrosomia risk, but if taken to an extreme this may possibly result in fetal nutritional insufficiency and growth restriction. Furthermore, fetal poly-unsaturated fatty acid (PUFA) supply is already decreased in GDM and this may be worsened if general fatty acid transfer is decreased further.
Myo-inositol supplementation may also alter how the placenta metabolizes and transports nutrients. For example, inositol availability will impact IGF signaling, which requires the conversion of PIP2 into PIP3 (Section “Inositol and fetal development”). Increased IGF signaling in GDM is thought to increase placental glucose and amino acid transport and therefore fetal growth (Jansson et al. Citation2013; Baumann et al. Citation2014; Stanirowski et al. Citation2017). However, it is not clear whether inositol supplementation would increase or decrease IGF signaling, since whilst increased PIP2 would increase IGF signaling, increased PIP3 would decrease IGF signaling.
Our studies of labeled fatty acid uptake by human placental explants from non-GDM pregnancies showed that myo-inositol treatment in-vitro reduced the incorporation of the LC-PUFA, DHA, into lipids, but increased the incorporation of the mono-unsaturated fatty acid, oleic acid, into lipids (Watkins et al. Citation2019). This suggests that myo-inositol acts differently on PUFA compared to non-PUFA. In a best-case scenario, reduced incorporation of PUFA into placental lipids could make available more un-esterified PUFA to be transported to the fetus to alleviate fetal PUFA deficiency. By the same logic, increased incorporation of non-PUFA into lipids could decrease the transport of non-PUFA and decrease the risk of GDM-associated macrosomia. However, further studies are needed to investigate the response of non-GDM and GDM placenta to inositol treatment and to explore how this may impact fetal growth.
Another consideration is how concurrent insulin treatment, during GDM management, may influence the effects of on-going inositol supplementation. In-vitro, insulin can stimulate cultured non-GDM placental trophoblast microvillous membranes to release the insulin mimic P-IPGs (Scioscia et al. Citation2008), potentially promoting further insulin-like actions in placenta and mother. In insulin-treated GDM, there are more insulin receptors in trophoblasts found at the end of pregnancy than in diet-treated GDM. Therefore, insulin treatment could potentially increase both placental P-IPG-insulin-mimic release and increase placental responsivity to insulin/insulin mimics through receptor up-regulation, in addition to the direct effects of insulin treatment on maternal tissues to lower glycemia (Scioscia et al. Citation2008; Desoye and Hauguel-de Mouzon Citation2007).
How these different actions of inositol and inositol derivatives, and the actions of various hormones interact and work together to bring about maternal adaptations to pregnancy and optimize fetal growth is not well studied to date. Neither is it understood how inositol supplementation and pathological states like GDM alter these actions.
Another important consideration is that myo-inositol supplementation is currently being proposed as a preventive measure for GDM. Such a strategy would involve myo-inositol exposure of many pregnancies which would not ultimately develop GDM. Thus, any potential regulatory changes or harms brought about by inositol treatment during pregnancy needs to be properly evaluated and understood in both uncomplicated and at-risk pregnancies, before widespread implementation of programs for GDM prevention and treatment with myo-inositol supplementation.
Pre-eclampsia
Pre-eclampsia is characterized by the onset of new hypertension or exacerbation of preexisting hypertension, accompanied by significant proteinuria or multi-system disorder during pregnancy, with the pathophysiology arising from abnormal placental implantation and endothelial dysfunction (Cheng and Wang Citation2009). It is a major cause of maternal mortality and morbidity worldwide and is associated with intrauterine growth restriction (IUGR) and long-term health issues for both mother and baby (Cheng and Wang Citation2009; Ghulmiyyah and Sibai Citation2012). Pre-eclampsia is also a state of heightened insulin resistance, and patients with pre-eclampsia show many similar metabolic changes to those with GDM (Cheng and Wang Citation2009; Ghulmiyyah and Sibai Citation2012). In addition, women previously exposed to a pre-eclamptic pregnancy carry an increased risk of future diabetes and cardiovascular disease, while the associated hyperinsulinemia and hyperandrogenism of pregnancy may persist for up to 17 years after delivery (Kaaja et al. Citation1999; Sampson et al. Citation2004; Seely and Solomon Citation2003).
Inositol regulation and processing are also altered in pre-eclampsia, although inositol’s possible role in pre-eclampsia etiology remains unclear ( and ). Compared with control placentas, placentas from pre-eclamptic pregnancies showed higher inositol content, reduced expression of SMIT-1 protein and increased expression of the SMIT signal transcription factor TonEBP (Lee et al. Citation2014). The former two alterations in pre-eclampsia-affected placenta are in contrast to those reported in GDM-affected placentas, hinting at pathophysiological divergence, despite the apparent metabolic similarities of the two conditions described earlier. Like GDM, however, maternal urinary, placental and amniotic fluid P-IPGs are higher in pre-eclampsia cases than in uncomplicated pregnancies (Paine et al. Citation2006; Williams et al. Citation2007; Dawonauth et al. Citation2014). Furthermore, maternal urinary P-IPG rises steeply in the weeks prior to the clinical onset of signs of pre-eclampsia, and this increase was linked with exacerbated insulin resistance (Williams et al. Citation2007; Paine et al. Citation2010), just as in GDM. Nevertheless, longitudinal studies measuring maternal urinary P-IPG from the first trimester onwards (spot urine collection, normalized to creatinine) (Scioscia et al. Citation2011; Paine et al. Citation2010) showed P-IPG levels could not reliably predict pre-eclampsia, because healthy women also had brief sporadic episodes of increased P-IPG excretion for unknown reasons. It is to be noted that glucose consumption is known to cause a spike in circulating P-IPG in the non-pregnant state (Baillargeon et al. Citation2006) and it seems likely that the P-IPG content of individual urine or plasma samples will be influenced by previous food consumption. If this is the case, the brief sporadic episodes of increased P-IPG excretion seen in the study described above could be caused by dietary factors and future studies should take this into account, or set out to perform assessments in the fasting state.
Table 8. Overview of inositol dysregulation associated with conditions involving insulin resistance.
A key limitation of existing studies lies in the quantification methods for P-IPG. The increase in urinary P-IPG is larger when quantified by an antibody assay that detects only the pregnancy-specific P-IPG (Paine et al. Citation2006; Williams et al. Citation2007; Dawonauth et al. Citation2014), than when using the (less specific) PDH phosphatase activity assay that measures a variety of P-IPGs (Kunjara, Greenbaum, et al. Citation2000). This suggests that the pregnancy specific P-IPG may be largely responsible for urinary P-IPG increase in pre-eclampsia. Of note, pre-eclampsia was not associated with an increased amount of general serum P-IPGs as measured by pyruvate dehydrogenase assay (Paine et al. Citation2006). Since the antibody assay does not work well in plasma or serum, it is difficult to confirm if circulating pregnancy-specific P-IPG fraction is similarly increased.
Like in uncomplicated pregnancies, in pre-eclampsia there is more P-IPG in amniotic fluid than in corresponding maternal urine (Scioscia et al. Citation2011). However, in pre-eclamptic pregnancies, but apparently not in uncomplicated pregnancies, there are also higher amounts of P-IPG in the umbilical arteries and vein relative to maternal uterine vein and peripheral vein (Scioscia et al. Citation2011). This suggests that the increased maternal urinary, placental and amniotic fluid P-IPG observed in pre-eclampsia is likely of fetal origin (Scioscia et al. Citation2011). Since P-IPG can act as an insulin-like signal this could represent an example of fetal-maternal communication, possibly as an adaptive response to the increased maternal insulin resistance. Alternatively, P-IPG produced by the fetus may play a role in the evolving pathophysiology as subsequently discussed.
As mentioned earlier, placental IPGs may be cleaved and released in response to insulin by placental GPI-PLD (Scioscia et al. Citation2006; Scioscia et al. Citation2008; Deborde, Schofield, and Rademacher Citation2003). However, although placental P-IPG appears increased in pre-eclampsia (Kunjara, Greenbaum, et al. Citation2000), insulin-induced release of P-IPG is decreased in pre-eclamptic placental explants in-vitro (Scioscia et al. Citation2006; Scioscia et al. Citation2008; Deborde, Schofield, and Rademacher Citation2003). The net effect of alterations in maternal, placental and fetal inositol biology in pre-eclampsia is therefore unclear (Scioscia et al. Citation2006; Scioscia et al. Citation2008).
Furthermore, GPI-PLD protein expression at delivery is similar in placentas from both uncomplicated and pre-eclamptic pregnancies (Deborde, Schofield, and Rademacher Citation2003). This suggests that increased placental P-IPG could be due to increased synthesis or import, rather than to alterations in GPI cleavage, or to the increased insulin-resistance of pre-eclampsia placenta (Scioscia et al. Citation2006; Scioscia et al. Citation2008; Deborde, Schofield, and Rademacher Citation2003). However, the degree to which the placenta may become insulin resistant in pre-eclampsia is not well understood (Desoye and Hauguel-de Mouzon Citation2007; Challier, Hauguel, and Desmaizieres Citation1986).
It has been postulated that P-IPGs may play a role in the generation of pre-eclampsia pathology (Kunjara et al. Citation1999; Scioscia et al. Citation2011). Some P-IPGs have insulin-like effects, so the increase in P-IPG could alter maternal physiology to further compromise nutrient mobilization to the fetus resulting in fetal growth restriction (Kunjara et al. Citation1999; Scioscia et al. Citation2011). P-IPGs also increase glycogen synthesis in rat and bovine liver by activating pyruvate dehydrogenase phosphatase, glycogen synthase phosphatase, and glycerol-3-phosphate acyltransferase (Varela-Nieto, León, and Caro Citation1996). It has therefore been suggested that increased placental P-IPGs could be responsible for the observed increase in placental glycogen synthesis and glycogen synthase activity in pre-eclampsia (Deborde, Schofield, and Rademacher Citation2003). However, IPGs have a range of bioactivities (Kunjara, Greenbaum, et al. Citation2000), and the precise role of the pregnancy-specific P-IPG, and other IPGs in the dysregulation of maternal-fetal physiology remains to be elucidated.
Insulin signaling though the PI3K signaling pathway was decreased in pre-eclampsia in the placenta compared to controls (Scioscia et al. Citation2006). These changes decreased PIP3 and PKB activation which in turn are thought to cause endothelial dysfunction and insulin resistance (D'Oria et al., Citation2017). Human endothelial cells (HUVECs), exposed in vitro to myo-inositol or D-chiro-inositol, showed increased PKB phosphorylation on Ser473, reflective of increased activation. Furthermore, the magnitude of changes induced by inositol treatment were greater than could be induced by insulin ( D'Oria et al. Citation2017). These findings suggest that inositol may be able to combat the pre-eclampsia-related defects in PI3K signaling, suggesting that increased inositol in pre-eclampsia could be adaptive.
Myo-inositol and D-chiro-inositol were also found to increase mitogen-activated protein kinase (MAPK) phosphorylation (D'Oria et al. Citation2017; Brancho et al. Citation2003). MAPK phosphorylation and activity are upregulated in both the placenta and umbilical cord in pre-eclampsia (Afroze et al. Citation2016). MAPK regulates diverse cellular processes, including placental development and trophoblast invasion and growth (Adams et al. Citation2000; Li et al. Citation2003), but whether the consequences of increased MAPK activation are protective or pathological in pre-elcampsia is unclear (Adams et al. Citation2000; Li et al. Citation2003; Peters et al. Citation2000).
Overall, it is not yet clear how systemic and local inositol concentrations are altered in pre-eclampsia, nor how this may alter maternal insulin resistance (D'Oria et al. Citation2017). It is thus, too early to suggest if inositols or their derivatives could be potential therapeutic targets in pre-eclampsia.
Conclusion
Inositols and their derivatives are highly bioactive molecules that influence glycemia regulation, insulin signaling and lipid metabolism. Optimal inositol intake, metabolism, transport and excretion are necessary for health, and inositol dysregulation is associated with conditions involving insulin resistance, dysglycemia and deranged lipidemia, including those occurring during pregnancy such as gestational diabetes and pre-eclampsia ().
Inositols participate in maternal-placental-fetal cross talk and play an important regulatory role in the adaptation of maternal physiology to nurture a healthy pregnancy. Thus, inositol supplements could be promising interventions for the prevention and treatment of a range of metabolic disorders and in adverse conditions in pregnancy such as GDM. However, their effects need to be better understood in order to ensure they are used safely and effectively, targeting relevant populations.
Author contributions and acknowledgements
Manuscript was written by Watkins O.C., and Chan S.Y. with advice and editing provided by Yong H.E.J. and Sharma N. We acknowledge Celes Maria Catherine Dado, Samantha Grace Loon Magadia, Preben Selvam, Victoria K. Cracknell and Reshma Appukuttan Pillai for their support in preparing this manuscript.
Supplemental Material
Download MS Word (12.9 KB)Disclosure statement
This research is supported by a Clinician Scientist Award awarded to Chan S.Y. from the Singapore National Medical Research Council (NMRC/CSA-INV/0010/2016), by the National University of Singapore, National University Health System Singapore and the A*STAR Singapore Institute for Clinical Sciences. SYC is part of an academic consortium that has received research funding from Nestec and is a co-inventor on pending patents, which covers the use of inositol in human health applications. The other authors have no financial or personal conflict of interest to declare.
References
- Adams, R. H., A. Porras, G. Alonso, M. Jones, K. Vintersten, S. Panelli, A. Valladares, L. Perez, R. Klein, and A. R. Nebreda. 2000. Essential role of p38α MAP kinase in placental but not embryonic cardiovascular development. Molecular Cell 6 (1):109–116. doi: 10.1016/S1097-2765(05)00014-6.
- Afroze, S. H., R. R. Kalagiri, M. Reyes, J. D. Zimmerman, M. R. Beeram, N. Drever, D. C. Zawieja, T. J. Kuehl, and M. N. Uddin. 2016. Apoptotic and stress signaling markers are augmented in preeclamptic placenta and umbilical cord. BBA Clinical 6:25–30. doi: 10.1016/j.bbacli.2016.05.003.
- Alvarez, J., J. Sanchez-Arias, A. Guadano, F. Estevez, I. Varela, J. Feliu, and J. Mato. 1991. Transport in isolated rat hepatocytes of the phospho-oligosaccharide that mimics insulin action. Effects of adrenalectomy and glucocorticoid treatment. Biochemical Journal 274 (2):369–74. doi: 10.1042/bj2740369.
- Amaefule, C. E., Z. Drymoussi, J. Dodds, L. Sweeney, E. Pizzo, J. Daru, J. Robson, L. Poston, A. Khalil, J. Myers, et al. 2018. Effectiveness and acceptability of myo-inositol nutritional supplement in the prevention of gestational diabetes (EMmY): A protocol for a randomised, placebo-controlled, double-blind pilot trial. BMJ Open 8 (9):e022831., doi: 10.1136/bmjopen-2018-022831.
- Andersen, D., and B. Holub. 1976. The influence of dietary inositol on glyceride composition and synthesis in livers of rats fed different fats. The Journal of Nutrition 106 (4):529–36. doi: 10.1093/jn/106.4.529.
- Andersen, D., and B. Holub. 1980. Myo-inositol-responsive liver lipid accumulation in the rat. The Journal of Nutrition 110 (3):488–95. doi: 10.1093/jn/110.3.488.
- Andersen, D., and B. Holub. 1980. The relative response of hepatic lipids in the rat to graded levels of dietary myo-inositol and other lipotropes. The Journal of Nutrition 110 (3):496–504. doi: 10.1093/jn/110.3.496.
- Anderson, R. G., B. A. Kamen, K. G. Rothberg, and S. W. Lacey. 1992. Potocytosis: Sequestration and transport of small molecules by caveolae. Science255 (5043):410–412. doi: 10.1126/science.1310359.
- Antonsson, B. E. 1994. Purification and characterization of phosphatidylinositol synthase from human placenta. Biochemical Journal 297 (3):517–522. doi: 10.1042/bj2970517.
- Antony, P. J., G. R. Gandhi, A. Stalin, K. Balakrishna, E. Toppo, K. Sivasankaran, S. Ignacimuthu, and N. A. Al-Dhabi. 2017. Myoinositol ameliorates high-fat diet and streptozotocin-induced diabetes in rats through promoting insulin receptor signaling. Biomedicine & Pharmacotherapy = Biomedecine & Pharmacotherapie 88:1098–113. doi: 10.1016/j.biopha.2017.01.170.
- Aouameur, R., S. Da Cal, P. Bissonnette, M. J. Coady, and J.-Y. Lapointe. 2007. SMIT2 mediates all myo-inositol uptake in apical membranes of rat small intestine. American Journal of Physiology. Gastrointestinal and Liver Physiology 293 (6):G1300–G1307. doi: 10.1152/ajpgi.00422.2007.
- Arner, R. J., K. S. Prabhu, J. T. Thompson, G. R. Hildenbrandt, A. D. Liken, and C. C. Reddy. 2001. Myo-Inositol oxygenase: Molecular cloning and expression of a unique enzyme that oxidizes myo-inositol and D-chiro-inositol. The Biochemical Journal 360 (Pt 2):313–320. doi: 10.1042/bj3600313.
- Arner, R. J., K. S. Prabhu, V. Krishnan, M. C. Johnson, and C. C. Reddy. 2006. Expression of myo-inositol oxygenase in tissues susceptible to diabetic complications. Biochemical and Biophysical Research Communications 339 (3):816–820. doi: 10.1016/j.bbrc.2005.11.090.
- Artini, P. G., O. Di Berardino, F. Papini, A. Genazzani, G. Simi, M. Ruggiero, and V. Cela. 2013. Endocrine and clinical effects of myo-inositol administration in polycystic ovary syndrome. A randomized study. Gynecological Endocrinology 29 (4):375–379. doi: 10.3109/09513590.2012.743020.
- Ashcroft, F. M., M. Rohm, A. Clark, and M. F. Brereton. 2017. Is type 2 diabetes a glycogen storage disease of pancreatic β cells? Cell Metabolism 26 (1):17–23. doi: 10.1016/j.cmet.2017.05.014.
- Asplin, I., G. Galasko, and J. Larner. 1993. Chiro-inositol deficiency and insulin resistance: A comparison of the chiro-inositol-and the myo-inositol-containing insulin mediators isolated from urine, hemodialysate, and muscle of control and type II diabetic subjects. Proceedings of the National Academy of Sciences 90 (13):5924–8. doi: 10.1073/pnas.90.13.5924.
- Baillargeon, J. P., E. Diamanti-Kandarakis, R. E. Ostlund, T. Apridonidze, M. J. Iuorno, and J. E. Nestler. 2006. Altered D-chiro-inositol urinary clearance in women with polycystic ovary syndrome. Diabetes Care 29 (2):300–5. doi: 10.2337/diacare.29.02.06.dc05-1070.
- Baillargeon, J.-P., J. E. Nestler, R. E. Ostlund, T. Apridonidze, and E. Diamanti-Kandarakis. 2008. Greek hyperinsulinemic women, with or without polycystic ovary syndrome, display altered inositols metabolism. Human Reproduction 23 (6):1439–46. doi: 10.1093/humrep/den097.
- Baillargeon, J.-P., M. J. Iuorno, D. J. Jakubowicz, T. Apridonidze, N. He, and J. E. Nestler. 2004. Metformin therapy increases insulin-stimulated release of D-chiro-inositol-containing inositolphosphoglycan mediator in women with polycystic ovary syndrome. The Journal of Clinical Endocrinology and Metabolism 89 (1):242–9. doi: 10.1210/jc.2003-030437.
- Baillargeon, J. P., M. J. Iuorno, T. Apridonidze, and J. E. Nestler. 2010. Uncoupling between insulin and release of a D-chiro-inositol–containing inositolphosphoglycan mediator of insulin action in obese women with polycystic ovary syndrome. Metabolic Syndrome and Related Disorders 8 (2):127–36. doi: 10.1089/met.2009.0052.
- Baker, L., R. Piddington, A. Goldman, J. Egler, and J. Moehring. 1990. Myo-inositol and prostaglandins reverse the glucose inhibition of neural tube fusion in cultured mouse embryos. Diabetologia 33 (10):593–596. doi: 10.1007/BF00400202.
- Balla, T. 2013. Phosphoinositides: Tiny lipids with giant impact on cell regulation. Physiological Reviews 93 (3):1019–137. doi: 10.1152/physrev.00028.2012.
- Barberini, L., A. Noto, C. Fattuoni, D. Grapov, A. Casanova, G. Fenu, M. Gaviano, R. Carboni, G. Ottonello, M. Crisafulli, et al. 2014. Urinary metabolomics (GC-MS) reveals that low and high birth weight infants share elevated inositol concentrations at birth. The Journal of Maternal-Fetal & Neonatal Medicine 27 (sup2):20–26., doi: 10.3109/14767058.2014.954786.
- Barker, C. J., and P.-O. Berggren. 2013. New horizons in cellular regulation by inositol polyphosphates: Insights from the pancreatic β-cell. Pharmacological Reviews 65 (2):641–69. doi: 10.1124/pr.112.006775.
- Barker, D. 2004. The developmental origins of adult disease. Journal of the American College of Nutrition 23 (sup6):588S–595S. doi: 10.1080/07315724.2004.10719428.
- Battaglia, F. C., G. Meschia, J. N. Blechner, and D. H. Barron. 1961. The free myo‐inositol concentration of adult and fetal tissues of several species. Quarterly Journal of Experimental Physiology and Cognate Medical Sciences 46:188–193. doi: 10.1113/expphysiol.1961.sp001532.
- Baumann, M. U., H. Schneider, A. Malek, V. Palta, D. V. Surbek, R. Sager, S. Zamudio, and N. P. Illsley. 2014. Regulation of human trophoblast GLUT1 glucose transporter by insulin-like growth factor I (IGF-I). PLoS One 9 (8):e106037. doi: 10.1371/journal.pone.0106037.
- Bayon, Y., M. Croset, V. Chirouze, J. Tayot, and M. Lagarde. 1993. Phospholipid molecular species from human placenta lipids. Lipids 28 (7):631–636. doi: 10.1007/BF02536058.
- Beach, D. C., and P. K. Flick. 1982. Early effect of myo-inositol deficiency on fatty acid synthetic enzymes of rat liver. Biochimica et Biophysica Acta (BBA) - Lipids and Lipid Metabolism 711 (3):452–9. doi: 10.1016/0005-2760(82)90059-5.
- Behuria, B.,. S. Ghimere, S. Choudhary, M. Agrawal, R. P. Bodroth, and T. V. G. Rao. 2018. Possible enhancement of phosphatidyl inositol (PI) depends on its metabolic pathway components as second messengers: Evidence from the growth and pi levels of normal, PI grown, and UV exposed Saccharomyces cerevisiae. Global Journal of Biology. Agriculture & Health Sciences 7:26–31.
- Bellamy, L., J. P. Casas, A. D. Hingorani, and D. Williams. 2009. Type 2 diabetes mellitus after gestational diabetes: A systematic review and meta-analysis. The Lancet 373 (9677):1773–1779. doi: 10.1016/S0140-6736(09)60731-5.
- Berridge, M. J. 2009. Inositol trisphosphate and calcium signalling mechanisms. Biochimica et Biophysica Acta (Bba) - Molecular Cell Research 1793 (6):933–40. doi: 10.1016/j.bbamcr.2008.10.005.
- Berridge, M. J., and R. F. Irvine. 1989. Inositol phosphates and cell signalling. Nature 341 (6239):197–205. doi: 10.1038/341197a0.
- Berry, G. T., J. J. Mallee, H. M. Kwon, J. S. Rim, W. R. Mulla, M. Muenke, and N. B. Spinner. 1995. The human osmoregulatory Na+/myo-inositol cotransporter gene (SLC5A3): molecular cloning and localization to chromosome 21. Genomics 25 (2):507–513. doi: 10.1016/0888-7543(95)80052-N.
- Berry, G. T., Z. J. Wang, S. F. Dreha, B. M. Finucane, and R. A. Zimmerman. 1999. In vivo brain myo-inositol levels in children with Down syndrome. The Journal of Pediatrics 135 (1):94–97. doi: 10.1016/S0022-3476(99)70334-3.
- Bersudsky, Y., H. Einat, Z. Stahl, and R. H. Belmaker. 1999. Epi-inositol and inositol depletion: Two new treatment approaches in affective disorder. Current Psychiatry Reports 1 (2):141–147. doi: 10.1007/s11920-999-0023-z.
- Best, C., C. Lucas, J. M. Patterson, and J. H. Ridout. 1946. The influence of biotin upon the relative lipotropic effects of choline and inositol. The Biochemical Journal 40 (3):368–73.
- Bevilacqua, A., G. Carlomagno, S. Gerli, M. Montanino Oliva, P. Devroey, A. Lanzone, C. Soulange, F. Facchinetti, G. Carlo Di Renzo, M. Bizzarri, et al. 2015. Results from the International Consensus Conference on myo-inositol and D-chiro-inositol in Obstetrics and Gynecology–assisted reproduction technology. Gynecological Endocrinology 31 (6):441–6. doi: 10.3109/09513590.2015.1006616.
- Bitsanis, D., M. A. Crawford, T. Moodley, H. Holmsen, K. Ghebremeskel, and O. Djahanbakhch. 2005. Arachidonic acid predominates in the membrane phosphoglycerides of the early and term human placenta. The Journal of Nutrition 135 (11):2566–71. doi: 10.1093/jn/135.11.2566.
- Bizzarri, M., and G. Carlomagno. 2014. Inositol: History of an effective therapy for polycystic ovary syndrome. European Review for Medical and Pharmacological Sciences 18 (13):1896–903.
- Blazer-Yost, B. L., and C. Nofziger. 2005. Phosphoinositide lipid second messengers: New paradigms for transepithelial signal transduction. Pflugers Archiv: European Journal of Physiology 450 (2):75–82. doi: 10.1007/s00424-004-1371-5.
- Boone, D. L., W. D. Currie, and P. C. K. Leung. 1993. Arachidonic acid and cell signalling in the ovary and placenta. Prostaglandins, Leukotrienes and Essential Fatty Acids 48 (1):79–87. doi: 10.1016/0952-3278(93)90013-M.
- Bourgeois, F., M. J. Coady, and J.-Y. Lapointe. 2005. Determination of transport stoichiometry for two cation-coupled myo-inositol cotransporters: SMIT2 and HMIT. The Journal of Physiology 563 (Pt 2):333–343. doi: 10.1113/jphysiol.2004.076679.
- Brancho, D., N. Tanaka, A. Jaeschke, J.-J. Ventura, N. Kelkar, Y. Tanaka, M. Kyuuma, T. Takeshita, R. A. Flavell, and R. J. Davis. 2003. Mechanism of p38 MAP kinase activation in vivo. Genes & Development 17 (16):1969–1978. doi: 10.1101/gad.1107303.
- Brautigan, D. L., M. Brown, S. Grindrod, G. Chinigo, A. Kruszewski, S. M. Lukasik, J. H. Bushweller, M. Horal, S. Keller, S. Tamura, et al. 2005. Allosteric activation of protein phosphatase 2C by d-chiro-inositol − galactosamine, a putative mediator mimetic of insulin action. Biochemistry 44 (33):11067–73. doi: 10.1021/bi0508845.
- Brown, J., J. Crawford Tineke, and J. Alsweiler. 2015. Myo-inositol for preventing gestational diabetes – planning of reveiw. Cochrane Database Syst Rev 4
- Brusati, V., M. Jóźwik, M. Jóźwik, C. Teng, C. Paolini, A. M. Marconi, and F. C. Battaglia. 2005. Fetal and maternal non-glucose carbohydrates and polyols concentrations in normal human pregnancies at term. Pediatric Research 58 (4):700–704. doi: 10.1203/01.PDR.0000180549.86614.73.
- Bry, K., and M. Hallman. 1991. Perinatal Development of inositol synthesis and catabolism in rabbit kidney. Neonatology 60 (3-4):249–257. doi: 10.1159/000243416.
- Burgess, J. W., J. Boucher, T. A. Neville, P. Rouillard, C. Stamler, S. Zachariah, and D. L. Sparks. 2003. Phosphatidylinositol promotes cholesterol transport and excretion. Journal of Lipid Research 44 (7):1355–63. doi: 10.1194/jlr.M300062-JLR200.
- Burton, G. J., and E. Jauniaux. 2004. Placental oxidative stress: From miscarriage to preeclampsia. Journal of the Society for Gynecologic Investigation 11 (6):342–52. doi: 10.1016/j.jsgi.2004.03.003.
- Burton, G. J., M. Scioscia, and T. W. Rademacher. 2011. Endometrial secretions: Creating a stimulatory microenvironment within the human early placenta and implications for the aetiopathogenesis of preeclampsia. Journal of Reproductive Immunology 89 (2):118–25. doi: 10.1016/j.jri.2011.02.005.
- Burton, L. E., and W. W. Wells. 1976. Myo-inositol metabolism during lactation and development in the rat. The prevention of lactation-induced fatty liver by dietary myo-inositol. The Journal of Nutrition 106 (11):1617–28. doi: 10.1093/jn/106.11.1617.
- Burton, L. E., and W. W. Wells. 1977. Characterization of the lactation-dependent fatty liver in myo-inositol deficient rats. The Journal of Nutrition 107 (10):1871–83. doi: 10.1093/jn/107.10.1871.
- Burton, L. E., and W. W. Wells. 1979. Myo-inositol deficiency: Studies on the mechanism of lactation-dependent fatty liver formation in the rat. The Journal of Nutrition 109 (8):1483–91. doi: 10.1093/jn/109.8.1483.
- Van Calker, D, and R. H. Belmaker. 2000. The high affinity inositol transport system-implications for the pathophysiology and treatment of bipolar disorder. Bipolar Disorders 2 (2):102–7. doi:10.1034/j.1399-5618.2000.020203.x. PMC: 11252649
- Calker, D., and R. H. Belmaker. 2000. The high affinity inositol transport system–implications for the pathophysiology and treatment of bipolar disorder. Bipolar Disorders 2 (2):102–7. doi: 10.1034/j.1399-5618.2000.020203.x.
- Calogero, A., G. Gullo, S. La Vignera, R. Condorelli, and A. Vaiarelli. 2015. Myoinositol improves sperm parameters and serum reproductive hormones in patients with idiopathic infertility: A prospective double‐blind randomized placebo‐controlled study. Andrology 3 (3):491–5. doi: 10.1111/andr.12025.
- Campbell, W. W., M. D. Haub, J. D. Fluckey, R. E. Ostlund, Jr J. P. Thyfault, H. Morse-Carrithers, M. W. Hulver, and Z. K. Birge. 2004. Pinitol supplementation does not affect insulin-mediated glucose metabolism and muscle insulin receptor content and phosphorylation in older humans. The Journal of Nutrition 134 (11):2998–3003. doi: 10.1093/jn/134.11.2998.
- Campling, J., and D. Nixon. 1954. The inositol content of foetal blood and foetal fluids. The Journal of Physiology 126 (1):71–80. doi: 10.1113/jphysiol.1954.sp005192.
- Carlomagno, G., and V. Unfer. 2011. Inositol safety: Clinical evidences. European Review for Medical and Pharmacological Sciences 15 (8):931–6.
- Carlomagno, G., V. Unfer, and S. Roseff. 2011. The D-chiro-inositol paradox in the ovary. Fertility and Sterility 95 (8):2515–2516. doi: 10.1016/j.fertnstert.2011.05.027.
- Caro, H. N., S. Kunjara, T. Rademacher, Y. Leon, D. Jones, M. Avila, and I. Varela-Nieto. 1997. Isolation and partial characterisation of insulin-mimetic inositol phosphoglycans from human liver. Biochemical and Molecular Medicine 61 (2):214–28. doi: 10.1006/bmme.1997.2607.
- Catalano, P. M., J. P. Kirwan, S. Haugel-de Mouzon, and J. King. 2003. Gestational diabetes and insulin resistance: Role in short-and long-term implications for mother and fetus. The Journal of Nutrition 133 (5 Suppl 2):1674S–1683S. doi: 10.1093/jn/133.5.1674S.
- Cavalli, P., G. Tonni, E. Grosso, and C. Poggiani. 2011. Effects of inositol supplementation in a cohort of mothers at risk of producing an NTD pregnancy. Birth Defects Research Part A: Clinical and Molecular Teratology 91 (11):962–965. doi: 10.1002/bdra.22853.
- Celentano, C.,. B. Matarrelli, G. Pavone, E. Vitacolonna, P. A. Mattei, V. Berghella, and M. Liberati. 2020. The influence of different inositol stereoisomers supplementation in pregnancy on maternal gestational diabetes mellitus and fetal outcomes in high-risk patients: A randomized controlled trial. The Journal of Maternal-Fetal & Neonatal Medicine 33 (5):743–751. doi: 10.1080/14767058.2018.1500545.
- Ceriello, A., D. Giugliano, A. Quatraro, and F. D'Onofrio. 1988. Does a common mechanism induce diverse complications of diabetes? Diabetes Care 11 (4):372–3. doi: 10.2337/diacare.11.4.372.
- Challier, J., S. Hauguel, and V. Desmaizieres. 1986. Effect of insulin on glucose uptake and metabolism in the human placenta. The Journal of Clinical Endocrinology and Metabolism 62 (5):803–807. doi: 10.1210/jcem-62-5-803.
- Charalampous, F. C. 1959. Biochemical studies on inositol. Journal of Biological Chemistry 234 (2):220–7.
- Cheang, K. I., J. P. Baillargeon, P. A. Essah, R. E. Ostlund, T. Apridonize, L. Islam, and J. E. Nestler. 2008. Insulin-stimulated release of D-chiro-inositol-containing inositolphosphoglycan mediator correlates with insulin sensitivity in women with polycystic ovary syndrome . Metabolism: clinical and Experimental 57 (10):1390–7. doi: 10.1016/j.metabol.2008.05.008.
- Cheng, M.-H., and P.-H. Wang. 2009. Placentation abnormalities in the pathophysiology of preeclampsia. Expert Review of Molecular Diagnostics 9 (1):37–49. doi: 10.1586/14737159.9.1.37.
- Chiu, T. T., M. S. Rogers, E. L. Law, C. M. Briton-Jones, L. Cheung, and C. J. Haines. 2002. Follicular fluid and serum concentrations of myo-inositol in patients undergoing IVF: Relationship with oocyte quality. Human Reproduction 17 (6):1591–6. doi: 10.1093/humrep/17.6.1591.
- Chu, A. H., Tint, M. T. Chang, H. F. Wong, G. Yuan, W. L. Tull, D. Nijagal, B. Narayana, V. K. Meikle, and P. J. Chang Kt. 2020. High placental inositol content associated with suppressed pro-adipogenic effects of maternal glycaemia in offspring: The GUSTO cohort. International Journal of Obesity 1–11.
- Chu, S. H. W., and D. Hegsted. 1980. Myo-inositol deficiency in gerbils: Comparative study of the intestinal lipodystrophy in Meriones unguiculatus and Meriones libycus. The Journal of Nutrition 110 (6):1209–16. doi: 10.1093/jn/110.6.1209.
- Chukwuma, C. I., M. A. Ibrahim, and M. S. Islam. 2016. Myo-inositol inhibits intestinal glucose absorption and promotes muscle glucose uptake: A dual approach study. Journal of Physiology and Biochemistry 72 (4):791–801. doi: 10.1007/s13105-016-0517-1.
- Cianci, A., M. Panella, M. Fichera, C. Falduzzi, M. Bartolo, and S. Caruso. 2015. D-chiro-Inositol and alpha lipoic acid treatment of metabolic and menses disorders in women with PCOS. Gynecological Endocrinology 31 (6):483–6. doi: 10.3109/09513590.2015.1014784.
- Clements Jr, R. 1979. The metabolism of myo-inositol by the human kidney. Journal of Laboratory and Clinical Medicine 93:210–9.
- Cline, G. W., K. F. Petersen, M. Krssak, J. Shen, R. S. Hundal, Z. Trajanoski, S. Inzucchi, A. Dresner, D. L. Rothman, and G. I. Shulman. 1999. Impaired glucose transport as a cause of decreased insulin-stimulated muscle glycogen synthesis in type 2 diabetes. The New England Journal of Medicine 341 (4):240–6. doi: 10.1056/NEJM199907223410404.
- Cogram, P., S. Tesh, J. Tesh, A. Wade, G. Allan, N. D. Greene, and A. J. Copp. 2002. D-chiro-inositol is more effective than myo-inositol in preventing folate-resistant mouse neural tube defects. Human Reproduction (Oxford, England) 17 (9):2451–2458. doi: 10.1093/humrep/17.9.2451.
- Colazingari, S., M. Treglia, R. Najjar, and A. Bevilacqua. 2013. The combined therapy myo-inositol plus D-chiro-inositol, rather than D-chiro-inositol, is able to improve IVF outcomes: Results from a randomized controlled trial. Archives of Gynecology and Obstetrics 288 (6):1405–11. doi: 10.1007/s00404-013-2855-3.
- Colone, M., G. Marelli, V. Unfer, G. Bozzuto, A. Molinari, and A. Stringaro. 2010. Inositol activity in oligoasthenoteratospermia–an in vitro study. European Review for Medical and Pharmacological Sciences 14 (10):891–6.
- Condorelli, R. A., S. La Vignera, L. M. Mongioì, S. G. Vitale, A. S. Laganà, L. Cimino, and A. E. Calogero. 2017. A. Myo-inositol as a male fertility molecule: Speed them up. European Review for Medical and Pharmacological Sciences 21 (2 Suppl):30–35.
- Copp, A. J., and N. D. Greene. 2010. Genetics and development of neural tube defects. The Journal of Pathology 220 (2):217–230. doi: 10.1002/path.2643.
- Coppey, L. J., J. S. Gellett, E. P. Davidson, J. A. Dunlap, and M. A. Yorek. 2002. Effect of treating streptozotocin-induced diabetic rats with sorbinil, myo-inositol or aminoguanidine on endoneurial blood flow, motor nerve conduction velocity and vascular function of epineurial arterioles of the sciatic nerve. International Journal of Experimental Diabetes Research 3 (1):21–36. doi: 10.1080/15604280212525.
- Corrado, F., R. D’Anna, G. Di Vieste, D. Giordano, B. Pintaudi, A. Santamaria, and A. Di Benedetto. 2011. The effect of myoinositol supplementation on insulin resistance in patients with gestational diabetes. Diabetic Medicine 28 (8):972–975. doi: 10.1111/j.1464-5491.2011.03284.x.
- Coupland, N. J., C. J. Ogilvie, K. M. Hegadoren, P. Seres, C. C. Hanstock, and P. S. Allen. 2005. Decreased prefrontal Myo-inositol in major depressive disorder. Biological Psychiatry 57 (12):1526–34. doi: 10.1016/j.biopsych.2005.02.027.
- Coustan, D. R. 2013. Can a dietary supplement prevent gestational diabetes mellitus? Diabetes Care 36 (4):777–9. doi: 10.2337/dc12-2505.
- Crawford, M. 2000. Placental delivery of arachidonic and docosahexaenoic acids: implications for the lipid nutrition of preterm infants. The American Journal of Clinical Nutrition 71 (1 Suppl):275S–284S. doi: 10.1093/ajcn/71.1.275S.
- Crawford, T. J., C. A. Crowther, J. Alsweiler, and J. Brown. 2015. Antenatal dietary supplementation with myo-inositol in women during pregnancy for preventing gestational diabetes. Cochrane Database of Systematic Reviews
- Crowther, C. A., J. E. Hiller, J. R. Moss, A. J. McPhee, W. S. Jeffries, and J. S. Robinson. 2005. Australian carbohydrate intolerance study in pregnant women trial G. Effect of treatment of gestational diabetes mellitus on pregnancy outcomes. New England Journal of Medicine 352 (24):2477–2486. doi: 10.1056/NEJMoa042973.
- Croze, M. L., A. Géloën, and C. O. Soulage. 2015. Abnormalities in myo-inositol metabolism associated with type 2 diabetes in mice fed a high-fat diet: Benefits of a dietary myo-inositol supplementation. British Journal of Nutrition 113 (12):1862–75. doi: 10.1017/S000711451500121X.
- Croze, M. L., and C. O. Soulage. 2013. Potential role and therapeutic interests of myo-inositol in metabolic diseases. Biochimie 95 (10):1811–27. doi: 10.1016/j.biochi.2013.05.011.
- Czech, M. P. 2000. PIP2 and PIP3: Complex roles at the cell surface. Cell 100 (6):603–6. doi: 10.1016/S0092-8674(00)80696-0.
- D’Anna, R., and A. Santamaria. 2018. Myo-inositol supplementation in gestational diabetes. Nutrition and diet in maternal diabetes, 229–35.Springer.
- D’anna, R., V. Di Benedetto, P. Rizzo, E. Raffone, M. Interdonato, F. Corrado, and A. Di Benedetto. 2012. Myo-inositol may prevent gestational diabetes in PCOS women. Gynecological Endocrinology 28 (6):440–2. doi: 10.3109/09513590.2011.633665.
- D’Oria, R., L. Laviola, M. Scioscia, F. Fascilla, S. Bettocchi, and F. Giorgino. 2017. PKB/Akt phosphorylation in human umbilical vein endothelial cells is highly induced by myo-inositol and D-chiro inositol: Novel potential insights in the pathogenesis of preeclampsia. Pregnancy Hypertension: An International Journal of Women's Cardiovascular Health 7:56. doi: 10.1016/j.preghy.2016.10.005.
- DʼAnna, R., A. Di Benedetto, A. Scilipoti, A. Santamaria, M. L. Interdonato, E. Petrella, I. Neri, B. Pintaudi, F. Corrado, and F. Facchinetti. 2015. Myo-inositol supplementation for prevention of gestational diabetes in obese pregnant women: A randomized controlled trial. Obstetrics and Gynecology 126 (2):310–315. doi: 10.1097/AOG.0000000000000958.
- D'Anna, R., A. Scilipoti, D. Giordano, C. Caruso, M. L. Cannata, M. L. Interdonato, F. Corrado, and A. Di Benedetto. 2013. Di Benedetto A. myo-Inositol supplementation and onset of gestational diabetes mellitus in pregnant women with a family history of type 2 diabetes: A prospective, randomized, placebo-controlled study. Diabetes Care 36 (4):854–857. doi: 10.2337/dc12-1371.
- Daughaday, W. H., and J. Larner. 1954. The renal excretion of inositol in normal and diabetic human beings. The Journal of Clinical Investigation 33 (3):326–32. doi: 10.1172/JCI102901.
- Davanzo, P., K. Yue, M. A. Thomas, T. Belin, J. Mintz, T. Venkatraman, E. Santoro, S. Barnett, and J. McCracken. 2003. Proton magnetic resonance spectroscopy of bipolar disorder versus intermittent explosive disorder in children and adolescents. The American Journal of Psychiatry 160 (8):1442–52. doi: 10.1176/appi.ajp.160.8.1442.
- Davanzo, P., M. A. Thomas, K. Yue, T. Oshiro, T. Belin, M. Strober, and J. McCracken. 2001. Decreased anterior cingulate myo-inositol/creatine spectroscopy resonance with lithium treatment in children with bipolar disorder. Neuropsychopharmacology: Official Publication of the American College of Neuropsychopharmacology 24 (4):359–69. doi: 10.1016/S0893-133X(00)00207-4.
- Davis, A., M. Christiansen, J. F. Horowitz, S. Klein, M. K. Hellerstein, and R. E. Ostlund. 2000. Effect of pinitol treatment on insulin action in subjects with insulin resistance. Diabetes Care 23 (7):1000–5. doi: 10.2337/diacare.23.7.1000.
- Dawonauth, L., L. Rademacher, A. D. L’Omelette, S. Jankee, M. Y. L. K. Yan, R. B. Jeeawoody, and T. W. Rademacher. 2014. Urinary inositol phosphoglycan-P type: Near patient test to detect preeclampsia prior to clinical onset of the disease. A study on 416 pregnant Mauritian women. Journal of Reproductive Immunology 101-102:148–152. doi: 10.1016/j.jri.2013.06.001.
- De, V. L., A. M. La, V. Cappelli, A. Stendardi, R. Focarelli, M. Musacchio, and P. Piomboni. 2012. Evaluation of the treatment with D-chiro-inositol on levels of oxidative stress in PCOS patients. Minerva Ginecologica 64:531–8.
- Debierre-Grockiego, F., and R. T. Schwarz. 2010. Immunological reactions in response to apicomplexan glycosylphosphatidylinositolsF Debierre-Grockiego and R T Schwarz. Glycobiology 20 (7):801–11. doi: 10.1093/glycob/cwq038.
- Deborde, S., J. Schofield, and T. Rademacher. 2003. Placental GPI-PLD is of maternal origin and its GPI substrate is absent from placentae of pregnancies associated with pre-eclampsia. Journal of Reproductive Immunology 59 (2):277–294. doi: 10.1016/S0165-0378(03)00054-8.
- Del Monte, M. A., R. Rabbani, T. C. Diaz, S. A. Lattimer, J. Nakamura, M. C. Brennan, and D. A. Greene. 1991. Sorbitol, myo-inositol, and rod outer segment phagocytosis in cultured hRPE cells exposed to glucose: In vitro model of myo-inositol depletion hypothesis of diabetic complications. Diabetes 40 (10):1335–45. doi: 10.2337/diabetes.40.10.1335.
- Delhaes, F., S. A. Giza, T. Koreman, G. Eastabrook, C. A. McKenzie, S. Bedell, T. R. H. Regnault, and B. de Vrijer. 2018. Altered maternal and placental lipid metabolism and fetal fat development in obesity: Current knowledge and advances in non-invasive assessment. Placenta 69:118–124. doi: 10.1016/j.placenta.2018.05.011.
- Desoye, G., and S. Hauguel-de Mouzon. 2007. The human placenta in gestational diabetes mellitus: The insulin and cytokine network. Diabetes Care 30 (Supplement 2):S120–S126. doi: 10.2337/dc07-s203.
- Dessì, A., and V. Fanos. 2013. Myoinositol: A new marker of intrauterine growth restriction? Journal of Obstetrics and Gynaecology: Gynaecology 33 (8):776–80. doi: 10.3109/01443615.2013.831046.
- Di Cianni, G., R. Miccoli, L. Volpe, C. Lencioni, and S. Del Prato. 2003. Intermediate metabolism in normal pregnancy and in gestational diabetes. Diabetes/Metabolism Research and Reviews 19 (4):259–270. doi: 10.1002/dmrr.390.
- Di Daniel, E., M. H. Mok, E. Mead, C. Mutinelli, E. Zambello, L. L. Caberlotto, T. J. Pell, C. J. Langmead, A. J. Shah, G. Duddy, et al. 2009. Evaluation of expression and function of the H+/myo-inositol transporter HMIT. BMC Cell Biology 10 (1):54. doi: 10.1186/1471-2121-10-54.
- Di Paolo, G., and P. De Camilli. 2006. Phosphoinositides in cell regulation and membrane dynamics. Nature 443 (7112):651–7. doi: 10.1038/nature05185.
- Dona, G., C. Sabbadin, C. Fiore, M. Bragadin, F. L. Giorgino, E. Ragazzi, C. G. Bordin, and L. Armanini. 2012. D. Inositol administration reduces oxidative stress in erythrocytes of patients with polycystic ovary syndrome. European Journal of Endocrinology 166:703–10.
- D'Oria, R., L. Laviola, F. Giorgino, V. Unfer, S. Bettocchi, and M. Scioscia. 2017. PKB/Akt and MAPK/ERK phosphorylation is highly induced by inositols: Novel potential insights in endothelial dysfunction in preeclampsia. Pregnancy Hypertension 10:107–112. doi: 10.1016/j.preghy.2017.07.001.
- D'Souza, K., and R. M. Epand. 2014. Enrichment of phosphatidylinositols with specific acyl chains. Biochimica et Biophysica Acta (BBA) - Biomembranes 1838 (6):1501–8. doi: 10.1016/j.bbamem.2013.10.003.
- Ehrmann, D. A. 2005. Polycystic ovary syndrome. The New England Journal of Medicine 352 (12):1223–36. doi: 10.1056/NEJMra041536.
- Ehrmann, D. A., R. B. Barnes, R. L. Rosenfield, M. K. Cavaghan, and J. Imperial. 1999. Prevalence of impaired glucose tolerance and diabetes in women with polycystic ovary syndrome. Diabetes Care 22 (1):141–6. doi: 10.2337/diacare.22.1.141.
- Einat, H., and R. Belmaker. 2001. The effects of inositol treatment in animal models of psychiatric disorders. Journal of Affective Disorders 62 (1-2):113–121. doi: 10.1016/S0165-0327(00)00355-4.
- Eisenberg, F., Jr, and A. H. Bolden. 1963. Biosynthesis of inositol in rat testis homogenate. Biochemical and Biophysical Research Communications 12 (1):72–77. doi: 10.1016/0006-291X(63)90416-9.
- Elased, K. M., K. Gumaa, J. De Souza, H. Rahmoune, J. H. Playfair, and T. Rademacher. 2001. Reversal of type 2 diabetes in mice by products of malaria parasites: II. Role of inositol phosphoglycans (IPGs). Molecular Genetics and Metabolism 73 (3):248–58. doi: 10.1006/mgme.2001.3186.
- Eriksson, U. J., and L. H. Borg. 1993. Diabetes and embryonic malformations: Role of substrate-induced free-oxygen radical production for dysmorphogenesis in cultured rat embryos. Diabetes 42 (3):411–9. doi: 10.2337/diab.42.3.411.
- Eriksson, U., and L. Borg. 1991. Protection by free oxygen radical scavenging enzymes against glucose-induced embryonic malformations in vitro. Diabetologia 34 (5):325–31. doi: 10.1007/BF00405004.
- Facchinetti, F., M. Bizzarri, S. Benvenga, R. D’Anna, A. Lanzone, C. Soulage, D. G. C. Renzo, M. Hod, P. Cavalli, and T. T. Chiu. 2015. Results from the International Consensus Conference on Myo-inositol and d-chiro-inositol in Obstetrics and Gynecology: The link between metabolic syndrome and PCOS. European Journal of Obstetrics & Gynecology and Reproductive Biology 195:72–6.
- Fagerberg, L., B. M. Hallström, P. Oksvold, C. Kampf, D. Djureinovic, J. Odeberg, M. Habuka, S. Tahmasebpoor, A. Danielsson, K. Edlund, et al. 2014. Analysis of the human tissue-specific expression by genome-wide integration of transcriptomics and antibody-based proteomics. Molecular & Cellular Proteomics: Proteomics 13 (2):397–406. doi: 10.1074/mcp.M113.035600.
- Falavigna, M., M. I. Schmidt, J. Trujillo, L. F. Alves, E. R. Wendland, M. R. Torloni, S. Colagiuri, and B. B. Duncan. 2012. Effectiveness of gestational diabetes treatment: A systematic review with quality of evidence assessment. Diabetes Research and Clinical Practice 98 (3):396–405. doi: 10.1016/j.diabres.2012.09.002.
- Farese, R. V. 1983. The phosphatidate-phosphoinositide cycle: An intracellular messenger system in the action of hormones and neurotransmitters. Metabolism 32 (6):628–41. doi: 10.1016/0026-0495(83)90035-5.
- Farese, R. V., M. Standaert, K. Yamada, L. Huang, C. Zhang, D. Cooper, Z. Wang, Y. Yang, S. Suzuki, and T. Toyota. 1994. Insulin-induced activation of glycerol-3-phosphate acyltransferase by a chiro-inositol-containing insulin mediator is defective in adipocytes of insulin-resistant, type II diabetic, Goto-Kakizaki rats. Proceedings of the National Academy of Sciences 91 (23):11040–4. doi: 10.1073/pnas.91.23.11040.
- Farren, M., N. Daly, A. McKeating, B. Kinsley, M. J. Turner, and S. Daly. 2017. The prevention of gestational diabetes mellitus with antenatal oral inositol supplementation: A randomized controlled trial. Diabetes Care 40 (6):759–763. doi: 10.2337/dc16-2449.
- Fenili, D. 2010. Characterization of inositol transporters as a method for drug delivery to the central nervous system.
- Fenili, D., M. Brown, R. Rappaport, and J. McLaurin. 2007. Properties of scyllo–inositol as a therapeutic treatment of AD-like pathology. Journal of Molecular Medicine (Berlin, Germany) 85 (6):603–11. doi: 10.1007/s00109-007-0156-7.
- Fisher, S. K., J. E. Novak, and B. W. Agranoff. 2002. Inositol and higher inositol phosphates in neural tissues: Homeostasis, metabolism and functional significance. Journal of Neurochemistry 82 (4):736–754. doi: 10.1046/j.1471-4159.2002.01041.x.
- Formuso, C., M. Stracquadanio, and L. Ciotta. 2015. Myo-inositol vs. D-chiro inositol in PCOS treatment. Minerva Ginecologica 67 (4):321–325.
- Fraser, D., S. Weitzman, J. Leiberman, and E. Eschwege. 1990. Factors influencing birth weight in newborns of diabetic and non-diabetic women a population based study. European Journal of Epidemiology 6 (4):427–431. doi: 10.1007/BF00151720.
- Fraticelli, F.,. C. Celentano, I. A. Zecca, G. Di Vieste, B. Pintaudi, M. Liberati, M. Franzago, M. Di Nicola, and E. Vitacolonna. 2018. Effect of inositol stereoisomers at different dosages in gestational diabetes: An open-label, parallel, randomized controlled trial. Acta Diabetologica 55 (8):805–812. doi: 10.1007/s00592-018-1157-4.
- Freedman, N. D., R. Sinha, S. C. Moore, A. J. Cross, J. N. Sampson, S. Boca, N. E. Caporaso, and W.-Y. Huang. 2014. Metabolites of tobacco smoking and colorectal cancer risk. Carcinogenesis 35 (7):1516–22. doi: 10.1093/carcin/bgu071.
- Frej, A. D., G. P. Otto, and R. S. Williams. 2017. Tipping the scales: Lessons from simple model systems on inositol imbalance in neurological disorders. European Journal of Cell Biology 96 (2):154–163. doi: 10.1016/j.ejcb.2017.01.007.
- Frey, R., D. Metzler, P. Fischer, A. Heiden, J. Scharfetter, E. Moser, and S. Kasper. 1998. Myo-inositol in depressive and healthy subjects determined by frontal 1H-magnetic resonance spectroscopy at 1.5 tesla. Journal of Psychiatric Research 32 (6):411–20. doi: 10.1016/S0022-3956(98)00033-8.
- Fruen, B., and B. Lester. 1991. High-affinity [3 H] inositol uptake by dissociated brain cells and cultured fibroblasts from fetal mice. Neurochemical Research 16 (8):913–918. doi: 10.1007/BF00965541.
- Fukami, K., and T. Takenawa. 1989. Quantitative changes in polyphosphoinositides 1, 2-diacylglycerol and inositol 1, 4, 5-trisphosphate by platelet-derived growth factor and prostaglandin F2 alpha. The Journal of Biological Chemistry 264 (25):14985–9.
- Gaggini, M., C. Saponaro, and A. Gastaldelli. 2015. Not all fats are created equal: Adipose vs. ectopic fat, implication in cardiometabolic diseases. Hormone Molecular Biology and Clinical Investigation 22 (1):7–18. doi: 10.1515/hmbci-2015-0006.
- Galasko, G.,. S. Abe, K. Lilley, C. Zhang, and J. Larner. 1996. Circulating factors and insulin resistance. II. The action of the novel myo-inositol cyclic 1,2-inositol phosphate phosphoglycan insulin antagonist from human plasma in regulating pyruvate dehydrogenase phosphatase. The Journal of Clinical Endocrinology and Metabolism 81 (3):1051–7. doi: 10.1210/jcem.81.3.8772575.
- Gallo, L. A., H. L. Barrett, and M. D. Nitert. 2017. Review: Placental transport and metabolism of energy substrates in maternal obesity and diabetes. Placenta 54:59–67. doi: 10.1016/j.placenta.2016.12.006.
- Ganapathy, V., P. D. Prasad, M. E. Ganapathy, and F. H. Leibach. 2000. Placental transporters relevant to drug distribution across the maternal-fetal interface. The Journal of Pharmacology and Experimental Therapeutics 294 (2):413–420.
- Gao J, Nalls MA, Shi M, Joubert BR, Hernandez DG, Huang X, Hollenbeck A, Singleton AB, Chen H. 2012. An exploratory analysis on gene-environment interactions for Parkinson disease. Neurobiology of Aging, 33:2528.e1.e2521–2528.e2526 doi: 10.1016/j.neurobiolaging.2012.06.007.
- Garcia-Perez, A., and M. B. Burg. 1990. Importance of organic osmolytes for osmoregulation by renal medullary cells. Hypertension16 (6):595–602. doi: 10.1161/01.HYP.16.6.595.
- Gateva, A., V. Unfer, and Z. Kamenov. 2018. The use of inositol (s) isomers in the management of polycystic ovary syndrome: A comprehensive review. Gynecological Endocrinology 34 (7):545–6. doi: 10.1080/09513590.2017.1421632.
- Gavin, G., and E. McHenry. 1941. Inositol: A lipotropic factor. Journal of Biological Chemistry 139
- Genazzani, A. D., A. Prati, S. Santagni, F. Ricchieri, E. Chierchia, E. Rattighieri, A. Campedelli, T. Simoncini, and P. G. Artini. 2012. Differential insulin response to myo-inositol administration in obese polycystic ovary syndrome patients. Gynecological Endocrinology 28 (12):969–973. doi: 10.3109/09513590.2012.685205.
- Gerli, S., E. Papaleo, A. Ferrari, D. Renzo, and G. Randomized. 2007. Double blind placebo-controlled trial: Effects of myo-inositol on ovarian function and metabolic factors in women with PCOS. European Review for Medical and Pharmacological Sciences 11:347–54.
- Ghulmiyyah, L., and B. Sibai. 2012. Maternal mortality from preeclampsia/eclampsia. Seminars in Perinatology 36 (1):56–59. doi: 10.1053/j.semperi.2011.09.011.
- Giordano, D., F. Corrado, A. Santamaria, S. Quattrone, B. Pintaudi, A. Di Benedetto, and R. D'Anna. 2011. Effects of myo-inositol supplementation in postmenopausal women with metabolic syndrome: A perspective, randomized, placebo-controlled study. Menopause18 (1):102–104. doi: 10.1097/gme.0b013e3181e8e1b1.
- Godfrey, K. M., W. Cutfield, S. Y. Chan, P. N. Baker, and Y. S. Chong. 2017. Nutritional intervention preconception and during pregnancy to maintain healthy glucose metabolism and offspring health (“NiPPeR”): Study protocol for a randomised controlled trial. Trials 18 (1):131. doi: 10.1186/s13063-017-1875-x.
- Goel, M., and V. N. Azev. 2009. The biological activity of structurally defined inositol glycans.
- Goodarzi, M. O., D. A. Dumesic, G. Chazenbalk, and R. Azziz. 2011. Polycystic ovary syndrome: Etiology, pathogenesis and diagnosis. Nature Reviews. Endocrinology 7 (4):219–31. doi: 10.1038/nrendo.2010.217.
- Grases, F., Simonet, B. M. Vucenik, I. Prieto, R. M. Costa,‐Bauzá, A. March, J. G. Shamsuddin. and A. M. 2001. Absorption and excretion of orally administered inositol hexaphosphate (IP6 or phytate) in humans. BioFactors 15 (1):53–61. doi: 10.1002/biof.5520150105.
- Greene, D. A., P. V. De Jesus, Jr., and A. I. Winegrad. 1975. Effects of insulin and dietary myoinositol on impaired peripheral motor nerve conduction velocity in acute streptozotocin diabetes. The Journal of Clinical Investigation 55 (6):1326–36. doi: 10.1172/JCI108052.
- Greene, D., and A. Mackway. 1986. Decreased myo-inositol content and Na+-K+-ATPase activity in superior cervical ganglion of STZ-diabetic rat and prevention by aldose reductase inhibition. Diabetes 35 (10):1106–8. doi: 10.2337/diab.35.10.1106.
- Greene, D., S. Chakrabarti, S. Lattimer, and A. Sima. 1987. Role of sorbitol accumulation and myo-inositol depletion in paranodal swelling of large myelinated nerve fibers in the insulin-deficient spontaneously diabetic bio-breeding rat. Reversal by insulin replacement, an aldose reductase inhibitor, and myo-inositol. The Journal of Clinical Investigation 79 (5):1479–85. doi: 10.1172/JCI112977.
- Groenen, P. M., H. M. Merkus, F. C. Sweep, R. A. Wevers, F. S. Janssen, and R. P. Steegers-Theunissen. 2003. Kinetics of myo-inositol loading in women of reproductive age. Annals of Clinical Biochemistry: International Journal of Laboratory Medicine 40 (1):79–85. doi: 10.1258/000456303321016213.
- Groenen, P. M., P. G. Peer, R. A. Wevers, D. W. Swinkels, B. Franke, E. C. Mariman, and R. P. Steegers-Theunissen. 2003. myo-inositol, glucose, and zinc status is associated with the risk of offspring with spina bifida. American Journal of Obstetrics and Gynecology 189 (6):1713–1719. doi: 10.1016/S0002-9378(03)00807-X.
- Groenen, P. M., R. A. Wevers, F. S. Janssen, J. H. Tuerlings, J. M. Merkus, and R. P. Steegers-Theunissen. 2003. Are myo-inositol, glucose and zinc concentrations in amniotic fluid of fetuses with spina bifida different from controls? Early Human Development 71 (1):1–8. doi: 10.1016/S0378-3782(02)00040-3.
- Guan, G.,. P. Dai, and I. Shechter. 2003. cDNA cloning and gene expression analysis of human myo-inositol 1-phosphate synthase. Archives of Biochemistry and Biophysics 417 (2):251–259. doi: 10.1016/S0003-9861(03)00388-6.
- Guo, W., S. Shimada, H. Tajiri, A. Yamauchi, T. Yamashita, S. Okada, and M. Tohyama. 1997. Developmental regulation of Na+/myo-inositol cotransporter gene expression. Molecular Brain Research 51 (1-2):91–96. doi: 10.1016/S0169-328X(97)00220-9.
- Handwerger, S., and M. Freemark. 2000. The roles of placental growth hormone and placental lactogen in the regulation of human fetal growth and development. Journal of Pediatric Endocrinology & Metabolism: JPEM 13 (4):343–356. doi: 10.1515/JPEM.2000.13.4.343.
- Hansen, B., and N. Bodkin. 1986. Heterogeneity of insulin responses: Phases leading to type 2 (non-insulin-dependent) diabetes mellitus in the rhesus monkey. Diabetologia 29 (10):713–9. doi: 10.1007/BF00870281.
- Hansen, S. B. 2015. Lipid agonism: The PIP 2 paradigm of ligand-gated ion channels. Biochimica et Biophysica Acta (BBA)-Molecular and Cell Biology of Lipids 1851:620–8.
- Harwood, A. J. 2005. Lithium and bipolar mood disorder: The inositol-depletion hypothesis revisited. Molecular Psychiatry 10 (1):117–26. doi: 10.1038/sj.mp.4001618.
- Hashimoto, M., S. Akazawa, M. Akazawa, M. Akashi, H. Yamamoto, Y. Maeda, Y. Yamaguchi, H. Yamasaki, D. Tahara, and T. Nakanishi. 1990. Effects of hyperglycaemia on sorbitol and myo-inositol contents of cultured embryos: Treatment with aldose reductase inhibitor and myo-inositol supplementation. Diabetologia 33 (10):597–602. doi: 10.1007/BF00400203.
- Hauguel-de Mouzon, S., J. Lepercq, and P. Catalano. 2006. The known and unknown of leptin in pregnancy. American Journal of Obstetrics and Gynecology 194 (6):1537–1545. doi: 10.1016/j.ajog.2005.06.064.
- Hauser, G., and V. N. Finelli. 1963. The biosynthesis of free and phosphatide myo-inositol from glucose by mammalian tissue slices. Journal of Biological Chemistry 238:64.
- Häussinger, D. 1998. Osmoregulation of liver cell function: signalling, osmolytes and cell heterogeneity. Cell Volume Regulation. Vol 123, ; 185–204 Karger Publishers.
- Hayashi, E., R. Hasegawa, and T. Tomita. 1976. Accumulation of neutral lipids in Saccharomyces carlsbergensis by myo-inositol deficiency and its mechanism. Reciprocal regulation of yeast acetyl-CoA carboxylase by fructose bisphosphate and citrate. The Journal of Biological Chemistry 251 (18):5759–69.
- Hayashi, E., T. Maeda, R. Hasegawa, and T. Tomita. 1978. The effect of myo-inositol deficiency on lipid metabolism in rats: III. The mechanism of an enhancement in lipolysis due to myo-inositol deficiency in rats. Biochimica et Biophysica Acta 531 (2):197–205. doi: 10.1016/0005-2760(78)90143-1.
- Hegsted, D., K. Hayes, A. Gallagher, and H. Hanford. 1973. Inositol deficiency: An intestinal lipodystrophy in the gerbil. The Journal of Nutrition 103 (2):302–7. doi: 10.1093/jn/103.2.302.
- Heimark, D., J. McAllister, and J. Larner. 2014. Decreased myo-inositol to chiro-inositol (M/C) ratios and increased M/C epimerase activity in PCOS theca cells demonstrate increased insulin sensitivity compared to controls. Endocrine Journal 61 (2):111–7. doi: 10.1507/endocrj.EJ13-0423.
- Hernández-Mijares, A., C. Bañuls, J. E. Peris, N. Monzó, A. Jover, L. Bellod, V. M. Victor, and M. Rocha. 2013. A single acute dose of pinitol from a naturally-occurring food ingredient decreases hyperglycaemia and circulating insulin levels in healthy subjects. Food Chemistry 141 (2):1267–72. doi: 10.1016/j.foodchem.2013.04.042.
- Herrera, E., and H. Ortega-Senovilla. 2010. Disturbances in lipid metabolism in diabetic pregnancy–are these the cause of the problem? Best Practice & Research Clinical Endocrinology & Metabolism 24 (4):515–525. doi: 10.1016/j.beem.2010.05.006.
- Herrera, E., and H. Ortega-Senovilla. 2018. Implications of lipids in neonatal body weight and fat mass in gestational diabetic mothers and non-diabetic controls. Current Diabetes Reports 18 (2):7. doi: 10.1007/s11892-018-0978-4.
- Hipps, P. P., K. E. Ackermann, and W. R. Sherman. 1982. Inositol epimerase - Inosose reductase from bovine brain. Methods in enzymology. Vol 89, 593–8. Academic Press.
- Hipps, P. P., W. H. Holland, and W. R. Sherman. 1977. Interconversion of myo- and scyllo-inositol with simultaneous formation of neo-inositol by an NADP + dependent epimerase from bovine brain. Biochemical and Biophysical Research Communications 77 (1):340–346. doi: 10.1016/S0006-291X(77)80202-7.
- Holub, B. J. 1986. Metabolism and function of myo-inositol and inositol phospholipids. Annual Review of Nutrition 6:563–97. doi: 10.1146/annurev.nu.06.070186.003023.
- Holub, B. J. The nutritional significance, metabolism, and function of myo-inositol and phosphatidylinositol in health and disease. Advances in nutritional research, 1982, 107–41. Springer.
- Hong, J. H., H. W. Jang, Y. E. Kang, J. H. Lee, K. S. Kim, H. J. Kim, K. R. Park, and B. J. Ku. 2012. Urinary chiro-and myo-inositol levels as a biological marker for type 2 diabetes mellitus. Disease Markers 33 (4):193–199. doi: 10.1155/2012/734718.
- Hori, H.,. T. Sasaoka, H. Ishihara, T. Wada, S. Murakami, M. Ishiki, and M. Kobayashi. 2002. Association of SH2-containing inositol phosphatase 2 with the insulin resistance of diabetic db/db mice. Diabetes 51 (8):2387–94. doi: 10.2337/diabetes.51.8.2387.
- Hu, M. L., Y. K. Chen, and Y. F. Lin. 1995. The antioxidant and prooxidant activity of some B vitamins and vitamin-like compounds. Chemico-Biological Interactions 97 (1):63–73. doi: 10.1016/0009-2797(95)03608-8.
- Huang, L., M. Fonteles, D. Houston, C. Zhang, and J. Larner. 1993. Chiroinositol deficiency and insulin resistance. III. Acute glycogenic and hypoglycemic effects of two inositol phosphoglycan insulin mediators in normal and streptozotocin-diabetic rats in vivo. Endocrinology 132 (2):652–7. doi: 10.1210/endo.132.2.8425485.
- I, E., H. d. Valk, B.Moll, E. t. Braak, and V. G. 2002. Macrosomia despite good glycaemic control in Type I diabetic pregnancy; results of a nationwide study in The Netherlands. Diabetologia 45 (11):1484–9. doi:10.1007/s00125-002-0958-7.
- Isaacks, R. E., A. S. Bender, C. Y. Kim, Y. F. Shi, and M. D. Norenberg. 1999. Effect of osmolality and anion channel inhibitors on myo‐inositol efflux in cultured astrocytes. Journal of Neuroscience Research 57 (6):866–871. doi: 10.1002/(SICI)1097-4547(19990915)57:6<866::AID-JNR12>3.0.CO;2-K.
- Ishida, S., A. Funakoshi, K. Miyasaka, H. Shimokata, F. Ando, and S. Takiguchi. 2006. Association of SH-2 containing inositol 5′-phosphatase 2 gene polymorphisms and hyperglycemia. Pancreas 33:63–7. doi: 10.1097/01.mpa.0000222317.82231.16.
- Islam, M. O., P. Selvam, R. Appukuttan Pillai, O. C. Watkins, and S. Y. Chan. 2019. An enzymatic assay for quantification of inositol in human term placental tissue. Analytical Biochemistry 586:113409. doi: 10.1016/j.ab.2019.113409.
- Iuorno, M., Maria, J. Jakubowicz, M. Daniela, J. Baillargeon, M. Jean-Patrice, D. B. Pamela, Gunn, M. Ronald, D. Allan, P. Nestler, G, et al. 2002. Effects of D-chiro-inositol in lean women with the polycystic ovary syndrome. Endocrine Practice: Official Journal of the American College of Endocrinology and the American Association of Clinical Endocrinologists 8 (6):417–23. doi: 10.4158/EP.8.6.417.
- Jackson, P. S., and K. Strange. 1993. Volume-sensitive anion channels mediate swelling-activated inositol and taurine efflux. American Journal of Physiology-Cell Physiology 265 (6):C1489–C1500. doi: 10.1152/ajpcell.1993.265.6.C1489.
- Jansson, N., F. J. Rosario, F. Gaccioli, S. Lager, H. N. Jones, S. Roos, T. Jansson, and T. L. Powell. 2013. Activation of placental mTOR signaling and amino acid transporters in obese women giving birth to large babies. The Journal of Clinical Endocrinology & Metabolism 98 (1):105–113. doi: 10.1210/jc.2012-2667.
- Jayabalan, N., S. Nair, Z. Nuzhat, G. E. Rice, F. A. Zuñiga, L. Sobrevia, A. Leiva, C. Sanhueza, J. A. Gutiérrez, M. Lappas, et al. 2017. Cross talk between adipose tissue and placenta in obese and gestational diabetes mellitus pregnancies via exosomes. Frontiers in Endocrinology 8:239. doi: 10.3389/fendo.2017.00239.
- Jenkins, C., R. Wilson, J. Roberts, H. Miller, J. H. McKillop, and J. J. Walker. 2000. Antioxidants: Their role in pregnancy and miscarriage. Antioxidants & Redox Signaling 2 (3):623–8. doi: 10.1089/15230860050192369.
- Jiang, W. D., L. Feng, Y. Liu, J. Jiang, and X. Q. Zhou. 2009. Myo‐inositol prevents oxidative damage, inhibits oxygen radical generation and increases antioxidant enzyme activities of juvenile Jian carp (Cyprinus carpio var. Jian. Aquaculture Research 40 (15):1770–6. doi: 10.1111/j.1365-2109.2009.02283.x.
- Johansson, M., T. Jansson, and T. Powell. 2000. Na+-K+-ATPase is distributed to microvillous and basal membrane of the syncytiotrophoblast in human placenta. American Journal of Physiology-Regulatory, Integrative and Comparative Physiology 279 (1):R287–R294. doi: 10.1152/ajpregu.2000.279.1.R287.
- Jung, T.-S., J.-R. Hahm, J.-J. Kim, J.-H. Jung, M.-Y. Kang, S.-W. Moon, K.-W. Lee, H.-C. Kim, J.-D. Lee, J.-H. Kim, et al. 2005. Determination of urinary myo-/chiro-inositol ratios from Korean diabetes patients. Yonsei Medical Journal 46 (4):532–8. doi: 10.3349/ymj.2005.46.4.532.
- Kaaja, R., H. Laivuori, M. Laakso, M. J. Tikkanen, and O. Ylikorkala. 1999. Evidence of a state of increased insulin resistance in preeclampsia. Metabolism 48 (7):892–896. doi: 10.1016/S0026-0495(99)90225-1.
- Kagawa, S., T. Sasaoka, S. Yaguchi, H. Ishihara, H. Tsuneki, S. Murakami, K. Fukui, T. Wada, S. Kobayashi, I. Kimura, et al. 2005. Impact of SRC homology 2-containing inositol 5′-phosphatase 2 gene polymorphisms detected in a Japanese population on insulin signaling. The Journal of Clinical Endocrinology & Metabolism 90 (5):2911–9., doi: 10.1210/jc.2004-1724.
- Kalra, B., S. Kalra, and J. Sharma. 2016. The inositols and polycystic ovary syndrome. Indian Journal of Endocrinology and Metabolism 20 (5):720–724. doi: 10.4103/2230-8210.189231.
- Kang, M.J., J.I. Kim, S.Y. Yoon, J. C. Kim, and I.J. Cha. 2006. Pinitol from soybeans reduces postprandial blood glucose in patients with type 2 diabetes mellitus. Journal of Medicinal Food 9 (2):182–6. doi: 10.1089/jmf.2006.9.182.
- Kawa, J. M., R. Przybylski, and C. G. Taylor. 2003. Urinary chiro-inositol and myo-inositol excretion is elevated in the diabetic db/db mouse and streptozotocin diabetic rat. Experimental Biology and Medicine (Maywood, N.J.) 228 (8):907–14. doi: 10.1177/153537020322800806.
- Kennington, A. S., C. R. Hill, J. Craig, C. Bogardus, I. Raz, H. K. Ortmeyer, B. C. Hansen, G. Romero, and J. Larner. 1990. Low urinary chiro-inositol excretion in non-insulin-dependent diabetes mellitus. New England Journal of Medicine 323 (6):373–8. doi: 10.1056/NEJM199008093230603.
- Kennington, O. A. 1992. Insulin mediators and their inositol components.
- Kim, H. J., K. S. Park, S. K. Lee, K. W. Min, K. A. Han, Y. K. Kim, and B. J. Ku. 2012. Effects of pinitol on glycemic control, insulin resistance and adipocytokine levels in patients with type 2 diabetes mellitus. Annals of Nutrition and Metabolism 60 (1):1–5. doi: 10.1159/000334834.
- Kim, J. N., S. N. Han, and H. K. Kim. 2014. Phytic acid and myo-inositol support adipocyte differentiation and improve insulin sensitivity in 3T3-L1 cells. Nutrition Research 34 (8):723–31. doi: 10.1016/j.nutres.2014.07.015.
- Kim, J., E. Darè, S. S. Rajasekaran, S. H. Ryu, P.-O. Berggren, and C. J. Barker. 2019. Inositol pyrophosphates and Akt/PKB: Is the pancreatic β-cell the exception to the rule? Cellular Signalling 58:131–6. doi: 10.1016/j.cellsig.2019.02.003.
- Kim, J.-I., J. Kim, M.-J. Kang, M.-S. Lee, J.-J. Kim, and I.-J. Cha. 2005. Effects of pinitol isolated from soybeans on glycaemic control and cardiovascular risk factors in Korean patients with type II diabetes mellitus: A randomized controlled study. European Journal of Clinical Nutrition 59 (3):456–458. doi: 10.1038/sj.ejcn.1602081.
- Kim, M. J., K. H. Yoo, J. H. Kim, Y. T. Seo, B. W. Ha, J. H. Kho, Y. G. Shin, and C. H. Chung. 2007. Effect of pinitol on glucose metabolism and adipocytokines in uncontrolled type 2 diabetes. Diabetes Research and Clinical Practice 77 (3):S247–S251. doi: 10.1016/j.diabres.2007.01.066.
- Kondoh, G., H. Tojo, Y. Nakatani, N. Komazawa, C. Murata, K. Yamagata, Y. Maeda, T. Kinoshita, M. Okabe, R. Taguchi, et al. 2005. Angiotensin-converting enzyme is a GPI-anchored protein releasing factor crucial for fertilization. Nature Medicine 11 (2):160–6. doi: 10.1038/nm1179.
- Krystal, G. 2000. Lipid phosphatases in the immune system. Seminars in Immunology 12 (4):397–403. doi: 10.1006/smim.2000.0222.
- Kunjara, S., A. L. Greenbaum, D.-Y. Wang, H. N. Caro, P. McLean, C. W. Redman, and T. W. Rademacher. 2000. Inositol phosphoglycans and signal transduction systems in pregnancy in preeclampsia and diabetes: Evidence for a significant regulatory role in preeclampsia at placental and systemic levels. Molecular Genetics and Metabolism 69 (2):144–58. doi: 10.1006/mgme.2000.2964.
- Kunjara, S., D. Y. Wang, A. L. Greenbaum, P. McLean, A. Kurtz, and T. W. Rademacher. 1999. Inositol phosphoglycans in diabetes and obesity: Urinary levels of IPG A-type and IPG P-type, and relationship to pathophysiological changes. Molecular Genetics and Metabolism 68 (4):488–502. doi: 10.1006/mgme.1999.2936.
- Kunjara, S., D. Y. Wang, P. McLean, A. L. Greenbaum, and T. W. Rademacher. 2000. Inositol phosphoglycans and the regulation of the secretion of leptin: In vitro effects on leptin release from adipocytes and the relationship to obesity. Molecular Genetics and Metabolism 70 (1):61–68. doi: 10.1006/mgme.2000.2988.
- Laganà, A. S., L. Barbaro, and A. Pizzo. 2015. Evaluation of ovarian function and metabolic factors in women affected by polycystic ovary syndrome after treatment with D-Chiro-Inositol. Archives of Gynecology and Obstetrics 291 (5):1181–6. doi: 10.1007/s00404-014-3552-6.
- Lager, S., and T. L. Powell. 2012. Regulation of nutrient transport across the placenta. Journal of Pregnancy 2012:1–14. doi: 10.1155/2012/179827.
- Lamar, M., C. M. L. Foy, F. Beacher, E. Daly, M. Poppe, N. Archer, V. Prasher, K. C. Murphy, R. G. Morris, A. Simmons, et al. 2011. Down syndrome with and without dementia: An in vivo proton magnetic resonance spectroscopy study with implications for Alzheimer's disease. Neuroimage 57 (1):63–8. doi: 10.1016/j.neuroimage.2011.03.073.
- Lampe, D., and B. V. L. Potter. 1993. Synthesis of 2-fluoro-2-deoxy-myo-inositol 1,4,5-trisphosphate and scyllo-inositol 1,2,4-trisphosphate, novel analogues of the second messenger myo-inositol 1,4,5-trisphosphate. Tetrahedron Letters 34 (14):2365–8. doi: 10.1016/S0040-4039(00)77615-5.
- Larner, J. 2002. D-chiro-inositol–its functional role in insulin action and its deficit in insulin resistance. Journal of Diabetes Research 3:47–60.
- Larner, J., D. L. Brautigan, and M. O. Thorner. 2010. D-chiro-inositol glycans in insulin signaling and insulin resistance. Molecular Medicine 16 (11-12):543–52. doi: 10.2119/molmed.2010.00107.
- Larner, J., J. D. Price, D. Heimark, L. Smith, G. Rule, T. Piccariello, M. C. Fonteles, C. Pontes, D. Vale, and L. Huang. 2003. Isolation, structure, synthesis, and bioactivity of a novel putative insulin mediator. A galactosamine chiro-inositol pseudo-disaccharide Mn2+ chelate with insulin-like activity. Journal of Medicinal Chemistry 46 (15):3283–91. doi: 10.1021/jm030071j.
- Larqué, E., A. Pagán, M. T. Prieto, J. E. Blanco, A. Gil-Sánchez, M. Zornoza-Moreno, M. Ruiz-Palacios, A. Gázquez, H. Demmelmair, J. J. Parrilla, et al. 2014. Placental fatty acid transfer: A key factor in fetal growth. Annals of Nutrition & Metabolism 64 (3-4):247–253., doi: 10.1159/000365028.
- Lawrence, J., J. Guinovart, and J. Larner. 1977. Activation of rat adipocyte glycogen synthase by insulins. The Journal of Biological Chemistry 252 (2):444–50.
- Lazar, D. F., J. J. Knez, M. E. Medof, P. Cuatrecasas, and A. R. Saltiel. 1994. Stimulation of glycogen synthesis by insulin in human erythroleukemia cells requires the synthesis of glycosyl-phosphatidylinositol. Proceedings of the National Academy of Sciences 91 (21):9665–9. doi: 10.1073/pnas.91.21.9665.
- Lee, G., M. Price, C. Mejia, H. Galan, and J. Arroyo. 2014. Increased trophoblast expression of NFAT5/TonEBP in pre-eclamptic placentas and hyperosmolar-treated BeWo cells. European Journal of Obstetrics & Gynecology and Reproductive Biology 183:37–43. doi: 10.1016/j.ejogrb.2014.10.002.
- Letcher, A. J., M. J. Schell, and R. F. Irvine. 2008. Do mammals make all their own inositol hexakisphosphate? Biochemical Journal 416 (2):263–70. doi: 10.1042/BJ20081417.
- Lewin, L. M., Y. Yannai, S. Melmed, and M. Weiss. 1982. myo-inositol in the reproductive tract of the female rat. The International Journal of Biochemistry 14 (2):147–150. doi: 10.1016/0020-711X(82)90154-9.
- Lewis, R. M., C. Wadsack, and G. Desoye. 2018. Placental fatty acid transfer. Current Opinion in Clinical Nutrition and Metabolic Care 21 (2):78–82. doi: 10.1097/MCO.0000000000000443.
- Li, H.-Y., S.-P. Chang, C.-C. Yuan, H.-T. Chao, H.-T. Ng, and Y.-J. Sung. 2003. Induction of p38 mitogen-activated protein kinase-mediated apoptosis is involved in outgrowth of trophoblast cells on endometrial epithelial cells in a model of human trophoblast-endometrial interactions. Biology of Reproduction 69 (5):1515–1524. doi: 10.1095/biolreprod.103.015669.
- Lin, X., L. Ma, C. Gopalan, and R. E. Ostlund. 2009. D-chiro-Inositol is absorbed but not synthesised in rodents. British Journal of Nutrition 102 (10):1426–1434. doi: 10.1017/S0007114509990456.
- Lin, X., L. Ma, R. L. Fitzgerald, and R. E. Ostlund. 2009. Human sodium/inositol cotransporter 2 (SMIT2) transports inositols but not glucose in L6 cells. Archives of Biochemistry and Biophysics 481 (2):197–201. doi: 10.1016/j.abb.2008.11.008.
- Liscovitch, M., M. Czarny, G. Fiucci, and T. Xiaoqing. 2000. Phospholipase D: Molecular and cell biology of a novel gene family. Biochemical Journal 345 (3):401–415. doi: 10.1042/bj3450401.
- Lizcano, J. M., and D. R. Alessi. 2002. The insulin signalling pathway. Current Biology: CB 12 (7):R236–R238. doi: 10.1016/S0960-9822(02)00777-7.
- Low, M. G. 2000. Structure and function of GPI-specific phospholipases. In PNH and the GPI-Linked Proteins, eds. N. S. Young and J. Moss, 239–68. San Diego: Academic Press.
- Loy, A., K. G. Lurie, A. Ghosh, J. M. Wilson, L. C. MacGregor, and F. M. Matschinsky. 1990. Diabetes and the myo-inositol paradox. Diabetes 39 (10):1305–12. doi: 10.2337/diab.39.10.1305.
- Lubin, V., R. Shojai, P. Darmon, and E. Cosson. 2016. A pilot study of gestational diabetes mellitus not controlled by diet alone: First-line medical treatment with myoinositol may limit the need for insulin. Diabetes & Metabolism 42 (3):192–195. doi: 10.1016/j.diabet.2016.01.005.
- Lubrich, B., and D. van Calker. 1999. Inhibition of the high affinity Myo-inositol transport system: A common mechanism of action of antibipolar drugs? Neuropsychopharmacology: Official Publication of the American College of Neuropsychopharmacology 21 (4):519–29. doi: 10.1016/S0893-133X(99)00037-8.
- Ma, K., L. A. M. Thomason, and J. McLaurin. 2012. scyllo-Inositol, Preclinical, and Clinical Data for Alzheimer’s Disease. In: Michaelis EK, Michaelis ML, eds. Advances in Pharmacology. Vol 64: Academic Press; 177–212.
- Ma, K., L. A. Thomason, and J. McLaurin. 2012. Scyllo-inositol, preclinical, and clinical data for Alzheimer’s disease. Advances in pharmacology. Vol 64, 177–212. Elsevier.
- Ma, R. C., G. E. Tutino, K. A. Lillycrop, M. A. Hanson, and W. H. Tam. 2015. Maternal diabetes, gestational diabetes and the role of epigenetics in their long term effects on offspring. Progress in Biophysics and Molecular Biology 118 (1-2):55–68. doi: 10.1016/j.pbiomolbio.2015.02.010.
- Mackenzie, R. W., and B. T. Elliott. 2014. Akt/PKB activation and insulin signaling: A novel insulin signaling pathway in the treatment of type 2 diabetes. Diabetes, metabolic syndrome and obesity. Targets and Therapy 7:55.
- Magnusson-Olsson, A. L., B. Hamark, A. Ericsson, M. Wennergren, T. Jansson, and T. L. Powell. 2006. Gestational and hormonal regulation of human placental lipoprotein lipase. Journal of Lipid Research 47 (11):2551–2561. doi: 10.1194/jlr.M600098-JLR200.
- Malik, A., F. Steinbeis, M. A. Carillo, P. H. Seeberger, B. Lepenies, and D. Varón Silva. 2020. Immunological evaluation of synthetic glycosylphosphatidylinositol glycoconjugates as vaccine candidates against malaria. ACS Chemical Biology 15 (1):171–8. doi: 10.1021/acschembio.9b00739.
- Malvasi, A., F. Casciaro, M. Minervini, I. Kosmas, O. Mynbaev, E. Pacella, V. M. Condesnitt, A. Creanza, D. Renzo, and G. Tinelli. 2014. A. Myo-inositol, D-chiro-inositol, folic acid and manganese in second trimester of pregnancy: A preliminary investigation. European Review for Medical and Pharmacological Sciences. 18:270–274.
- Manna, P., and S. K. Jain. 2013. PIP3 but not PIP2 increases GLUT4 surface expression and glucose metabolism mediated by AKT/PKCζ/λ phosphorylation in 3T3L1 adipocytes. Molecular and Cellular Biochemistry 381 (1-2):291–9. doi: 10.1007/s11010-013-1714-7.
- Mariggiò, S., C. Iurisci, J. Sebastià, J. Patton-Vogt, and D. Corda. 2006. Molecular characterization of a glycerophosphoinositol transporter in mammalian cells. FEBS Letters 580 (30):6789–96. doi: 10.1016/j.febslet.2006.11.039.
- Marincola, F. C., A. Dessi, M. G. Pattumelli, S. Corbu, C. Ossicini, S. Ciccarelli, R. Agostino, M. Mussap, and V. Fanos. 2015. 1H NMR-based urine metabolic profile of IUGR, LGA, and AGA newborns in the first week of life. Clinica Chimica Acta; International Journal of Clinical Chemistry 451:28–34. doi: 10.1016/j.cca.2015.08.008.
- Marion, E., P. J. Kaisaki, V. Pouillon, C. Gueydan, J. C. Levy, A. Bodson, G. Krzentowski, J.-C. Daubresse, J. Mockel, J. Behrends, et al. 2002. The gene INPPL1, encoding the lipid phosphatase SHIP2, is a candidate for type 2 diabetes in rat and man. Diabetes 51 (7):2012–7., doi: 10.2337/diabetes.51.7.2012.
- Matarrelli, B., E. Vitacolonna, M. D’angelo, G. Pavone, P. A. Mattei, M. Liberati, and C. Celentano. 2013. Effect of dietary myo-inositol supplementation in pregnancy on the incidence of maternal gestational diabetes mellitus and fetal outcomes: A randomized controlled trial. The Journal of Maternal-Fetal & Neonatal Medicine26 (10):967–972. doi: 10.3109/14767058.2013.766691.
- Maurizi, A. R., M. Menduni, R. Del Toro, S. Kyanvash, D. Maggi, C. Guglielmi, A. L. Pantano, G. Defeudis, E. Fioriti, S. Manfrini, et al. 2017. A pilot study of d-chiro-inositol plus folic acid in overweight patients with type 1 diabetes. Acta Diabetologica 54 (4):361–5. doi: 10.1007/s00592-016-0954-x.
- Mayer, J., and D. Tomlinson. 1983. Prevention of defects of axonal transport and nerve conduction velocity by oral administration of myo-inositol or an aldose reductase inhibitor in streptozotocin-diabetic rats. Diabetologia 25 (5):433–8. doi: 10.1007/BF00282524.
- McLean, P., S. Kunjara, A. L. Greenbaum, K. Gumaa, J. López-Prados, M. Martin-Lomas, and T. W. Rademacher. 2008. Reciprocal control of pyruvate dehydrogenase kinase and phosphatase by inositol phosphoglycans dynamic state set by “push-pull” system. The Journal of Biological Chemistry 283 (48):33428–36. doi: 10.1074/jbc.M801781200.
- McPhee, F., C. Downes, and G. Lowe. 1991. Studies of inositol analogues as inhibitors of the phosphoinositide pathway, and incorporation of 2-deoxy-2-fluoro-myo-inositol to give analogues of phosphatidylinositol intermediates. Biochemical Journal 277 (2):407–12. doi: 10.1042/bj2770407.
- Mejia, C. A., P. R. Reynolds, and J. A. Arroyo. 2016. Placental expression of NFAT5, SLC5A3, and aldose reductase in gestational diabetes mellitus. The FASEB Journal 30 (851):855–851.
- Metzger, B. E., L. P. Lowe, A. R. Dyer, E. R. Trimble, U. Chaovarindr, D. R. Coustan, D. R. Hadden, D. R. McCance, M. Hod, H. D. McIntyre, et al. 2008. Hyperglycemia and adverse pregnancy outcomes. The New England Journal of Medicine 358 (19):1991–2002. doi: 10.1056/NEJMoa0707943.
- Michaelis, T., G. Helms, K. D. Merboldt, W. Hänicke, H. Bruhn, and J. Frahm. 1993. Identification of scyllo‐inositol in proton NMR spectra of human brain in vivo. NMR in Biomedicine 6 (1):105–109. doi: 10.1002/nbm.1940060116.
- Michaelis, T., G. Helms, K.-D. Merboldt, W. Hänicke, H. Bruhn, and J. Frahm. 1993. Identification of scyllo-inositol in proton NMR spectra of human brain in vivo. NMR in Biomedicine 6 (1):105–109. doi: 10.1002/nbm.1940060116.
- Middleton, A., and B. Setchell. 1972. The origin of inositol in the rete testis fluid of the ram. Reproduction 30 (3):473–475. doi: 10.1530/jrf.0.0300473.
- Miller, A. T., P. P. Chamberlain, and M. P. Cooke. 2008. Beyond IP3: Roles for higher order inositol phosphates in immune cell signaling. Cell Cycle (Georgetown, Tex.) 7 (4):463–7. doi: 10.4161/cc.7.4.5518.
- Miñambres, I., G. Cuixart, A. Gonçalves, and R. Corcoy. 2019. Effects of inositol on glucose homeostasis: Systematic review and meta-analysis of randomized controlled trials. Clinical Nutrition38 (3):1146–52. doi: 10.1016/j.clnu.2018.06.957.
- Mitanchez, D., C. Yzydorczyk, B. Siddeek, F. Boubred, M. Benahmed, and U. Simeoni. 2015. The offspring of the diabetic mother - Short- and long-term implications. Best Practice & Research Clinical Obstetrics & Gynaecology 29 (2):256–269. doi: 10.1016/j.bpobgyn.2014.08.004.
- Moore, C. M., J. L. Breeze, S. A. Gruber, S. M. Babb, B. B. Frederick, R. A. Villafuerte, A. L. Stoll, J. Hennen, D. A. Yurgelun-Todd, B. M. Cohen, et al. 2000. Choline, myo‐inositol and mood in bipolar disorder: A proton magnetic resonance spectroscopic imaging study of the anterior cingulate cortex. Bipolar Disorders 2 (3 Pt 2):207–16. doi: 10.1034/j.1399-5618.2000.20302.x.
- Moore, G. J., J. M. Bebchuk, J. K. Parrish, M. W. Faulk, C. L. Arfken, J. Strahl-Bevacqua, and H. K. Manji. 1999. Temporal dissociation between lithium-induced changes in frontal lobe myo-inositol and clinical response in manic-depressive illness. The American Journal of Psychiatry 156 (12):1902–8. doi: 10.1176/ajp.156.12.1902.
- Mueckler, M., and B. Thorens. 2013. The SLC2 (GLUT) family of membrane transporters. Molecular Aspects of Medicine 34 (2-3):121–138. doi: 10.1016/j.mam.2012.07.001.
- Müller, G., S. Wied, C. Piossek, A. Bauer, J. Bauer, and W. Frick. 1998. Convergence and divergence of the signaling pathways for insulin and phosphoinositolglycans. Molecular Medicine 4 (5):299–323. doi: 10.1007/BF03401738.
- Murphy, A., A. Shamshirsaz, D. Markovic, R. Ostlund, and B. Koos. 2016. Urinary excretion of myo-inositol and d-chiro-inositol in early pregnancy is enhanced in gravidas with gestational diabetes mellitus. Reproductive Sciences (Thousand Oaks, Calif.) 23 (3):365–371. doi: 10.1177/1933719115602767.
- Muscogiuri, G., S. Palomba, A. S. Laganà, and F. Orio. 2016. Inositols in the treatment of insulin-mediated diseases. International Journal of Endocrinology 2016:1. doi: 10.1155/2016/6189820.
- Nagamatsu, S., and M. Ohara-Imaizumi. 2007. IP7 debut in insulin release. Science318 (5854):1249–50. doi: 10.1126/science.1151361.
- Nakamura, J., M. A. Del Monte, D. Shewach, S. A. Lattimer, and D. A. Greene. 1992. Inhibition of phosphatidylinositol synthase by glucose in human retinal pigment epithelial cells. American Journal of Physiology-Endocrinology and Metabolism 262 (4):E417–E426. doi: 10.1152/ajpendo.1992.262.4.E417.
- Napso, T., H. E. J. Yong, J. Lopez-Tello, and A. N. Sferruzzi-Perri. 2018. The role of placental hormones in mediating maternal adaptations to support pregnancy and lactation. Frontiers in Physiology 9:1091 doi: 10.3389/fphys.2018.01091.
- Nascimento, N. R., L. M. Lessa, M. R. Kerntopf, C. M. Sousa, R. S. Alves, M. G. Queiroz, J. Price, D. B. Heimark, J. Larner, X. Du, et al. 2006. Inositols prevent and reverse endothelial dysfunction in diabetic rat and rabbit vasculature metabolically and by scavenging superoxide. Proceedings of the National Academy of Sciences of the United States of America 103 (1):218–23. doi: 10.1073/pnas.0509779103.
- Navarro-González, J. F., A. Jarque, M. Muros, C. Mora, and J. García. 2009. Tumor necrosis factor-α as a therapeutic target for diabetic nephropathy. Cytokine & Growth Factor Reviews 20 (2):165–73. doi: 10.1016/j.cytogfr.2009.02.005.
- Nestler, J. E., and V. Unfer. 2015. Reflections on inositol(s) for PCOS therapy: Steps toward success. Gynecological Endocrinology31 (7):501–5. doi: 10.3109/09513590.2015.1054802.
- Nestler, J. E., D. J. Jakubowicz, A. F. de Vargas, C. Brik, N. Quintero, and F. Medina. 1998. Insulin stimulates testosterone biosynthesis by human thecal cells from women with polycystic ovary syndrome by activating its own receptor and using inositolglycan mediators as the signal transduction system. The Journal of Clinical Endocrinology and Metabolism 83 (6):2001–5. doi: 10.1210/jcem.83.6.4886.
- Nestler, J. E., D. J. Jakubowicz, P. Reamer, R. D. Gunn, and G. Allan. 1999. Ovulatory and metabolic effects of D-chiro-inositol in the polycystic ovary syndrome. New England Journal of Medicine 340 (17):1314–20. doi: 10.1056/NEJM199904293401703.
- Newsholme, P., E. Haber, S. Hirabara, E. Rebelato, J. Procópio, D. Morgan, H. Oliveira‐Emilio, A. R. Carpinelli, and R. Curi. 2007. Diabetes associated cell stress and dysfunction: Role of mitochondrial and non‐mitochondrial ROS production and activity. The Journal of Physiology 583 (Pt 1):9–24. doi: 10.1113/jphysiol.2007.135871.
- Nielson, J. R., and J. P. Rutter. 2018. Lipid-mediated signals that regulate mitochondrial biology. Journal of Biological Chemistry 001655
- Nissen, P. M., C. Nebel, N. Oksbjerg, and H. C. Bertram. 2011. Metabolomics reveals relationship between plasma inositols and birth weight: Possible markers for fetal programming of type 2 diabetes. Journal of Biomedicine and Biotechnology 2011:1–8. doi: 10.1155/2011/378268.
- Noble, K., J. Zhang, and S. Wray. 2006. Lipid rafts, the sarcoplasmic reticulum and uterine calcium signalling: An integrated approach. The Journal of Physiology 570 (Pt 1):29–35. doi: 10.1113/jphysiol.2005.098475.
- Nordio, M., and E. Proietti. 2012. The combined therapy with myo-inositol and D-chiro-inositol reduces the risk of metabolic disease in PCOS overweight patients compared to myo-inositol supplementation alone. European Review for Medical and Pharmacological Sciences 16 (5):575–81.
- Noventa, M., A. Vitagliano, M. Quaranta, S. Borgato, B. Abdulrahim, and S. Gizzo. 2016. Preventive and therapeutic role of dietary inositol supplementation in periconceptional period and during pregnancy: A summary of evidences and future applications. Reproductive Sciences (Thousand Oaks, Calif.) 23 (3):278–88. doi: 10.1177/1933719115594018.
- Nozadze, M., E. Mikautadze, E. Lepsveridze, E. Mikeladze, N. Kuchiashvili, T. Kiguradze, M. Kikvidze, and R. Solomonia. 2011. Anticonvulsant activities of myo-inositol and scyllo-inositol on pentylenetetrazol induced seizures. Seizure20 (2):173–176. doi: 10.1016/j.seizure.2010.10.008.
- Oben, J. A., A. Mouralidarane, A.-M. Samuelsson, P. J. Matthews, M. L. Morgan, C. McKee, J. Soeda, D. S. Fernandez-Twinn, M. S. Martin-Gronert, S. E. Ozanne, et al. 2010. Maternal obesity during pregnancy and lactation programs the development of offspring non-alcoholic fatty liver disease in mice. Journal of Hepatology 52 (6):913–20. doi: 10.1016/j.jhep.2009.12.042.
- Ortmeyer, H. K. 1996. Dietary myoinositol results in lower urine glucose and in lower postprandial plasma glucose in obese insulin resistant rhesus monkeys. Obesity Research 4 (6):569–75. doi: 10.1002/j.1550-8528.1996.tb00271.x.
- Ortmeyer, H. K., L. C. Huang, L. Zhang, B. C. Hansen, and J. Larner. 1993. Chiroinositol deficiency and insulin resistance. II. Acute effects of D-chiroinositol administration in streptozotocin-diabetic rats, normal rats given a glucose load, and spontaneously insulin-resistant rhesus monkeys. Endocrinology 132 (2):646–51. doi: 10.1210/endo.132.2.8425484.
- Ortmeyer, H. K., N. Bodkin, K. Lilley, J. Larner, and B. C. Hansen. 1993. Chiroinositol deficiency and insulin resistance. I. Urinary excretion rate of chiroinositol is directly associated with insulin resistance in spontaneously diabetic rhesus monkeys. Endocrinology 132 (2):640–5. doi: 10.1210/endo.132.2.8425483.
- Ostlund, R. E., J. B. McGill, I. Herskowitz, D. M. Kipnis, J. V. Santiago, and W. R. Sherman. 1993. D-chiro-inositol metabolism in diabetes mellitus. Proceedings of the National Academy of Sciences 90 (21):9988–92. doi: 10.1073/pnas.90.21.9988.
- Owczarczyk-Saczonek, A., L. B. Lahuta, M. Ligor, W. Placek, R. J. Górecki, and B. Buszewski. 2018. The healing-promoting properties of selected cyclitols—A review. Nutrients 10 (12):1891. doi: 10.3390/nu10121891.
- Paine, M. A., M. Scioscia, P. J. Williams, K. Gumaa, C. H. Rodeck, and T. W. Rademacher. 2010. Urinary inositol phosphoglycan P-type as a marker for prediction of preeclampsia and novel implications for the pathophysiology of this disorder. Hypertension in Pregnancy 29 (4):375–384. doi: 10.3109/10641950903242667.
- Paine, M., C. Rodeck, P. Williams, and T. Rademacher. 2003. Possible involvement of inositol phosphoglycan-P in human parturition. Journal of Reproductive Immunology 59 (2):267–75. doi: 10.1016/S0165-0378(03)00053-6.
- Paine, M., M. Scioscia, K. A. Gumaa, C. Rodeck, and T. Rademacher. 2006. P-type inositol phosphoglycans in serum and amniotic fluid in active pre-eclampsia. Journal of Reproductive Immunology 69 (2):165–79. doi: 10.1016/j.jri.2005.09.008.
- Pak, Y., and J. Larner. 1992. Identification and characterization of chiroinositol-containing phospholipids from bovine liver. Biochemical and Biophysical Research Communications 184 (2):1042–7. doi: 10.1016/0006-291X(92)90696-I.
- Pak, Y., C. R. Paule, Y.-D. Bao, L. C. Huang, and J. Larner. 1993. Insulin stimulates the biosynthesis of chiro-inositol-containing phospholipids in a rat fibroblast line expressing the human insulin receptor. Proceedings of the National Academy of Sciences 90 (16):7759–7763. doi: 10.1073/pnas.90.16.7759.
- Pak, Y., L. C. Huang, K. J. Lilley, and J. Larner. 1992. In vivo conversion of [3H]myoinositol to [3H]chiroinositol in rat tissues. Journal of Biological Chemistry 267:16904–16910.
- Pak, Y., Y. Hong, S. Kim, T. Piccariello, R. V. Farese, and J. Larner. 1998. Vivo chiro-Inositol Metabolism in the Rat: A Defect in chiroInositol Synthesis from myo-Inositol and an Increased Incorporation of chiro-[3H] lnositol into Phospholipid in the Goto-Kakizaki (GK) Rat. Molecules & Cells, 8. Springer Science & Business Media BV.
- Palmano, K., P. H. Whiting, and J. N. Hawthorne. 1977. Free and lipid myo-inositol in tissues from rats with acute and less severe streptozotocin-induced diabetes. The Biochemical Journal 167 (1):229–35. doi: 10.1042/bj1670229.
- Papaleo, E., V. Unfer, J. Baillargeon, and T. Chiu. 2009. Contribution of myo-inositol to reproduction. European Journal of Obstetrics & Gynecology and Reproductive Biology 147 (2):120–123. doi: 10.1016/j.ejogrb.2009.09.008.
- Papaleo, E., V. Unfer, J.-P. Baillargeon, L. De Santis, F. Fusi, C. Brigante, G. Marelli, I. Cino, A. Redaelli, and A. Ferrari. 2007. Myo-inositol in patients with polycystic ovary syndrome: A novel method for ovulation induction. Gynecological Endocrinology 23 (12):700–3. doi: 10.1080/09513590701672405.
- Parpal, S., J. Gustavsson, and P. Strålfors. 1995. Isolation of phosphooligosaccharide/phosphoinositol glycan from caveolae and cytosol of insulin-stimulated cells. The Journal of Cell Biology 131 (1):125–135. doi: 10.1083/jcb.131.1.125.
- Parthasarathy, R., L. Parthasarathy, and R. Vadnal. 1997. Brain inositol monophosphatase identified as a galactose 1-phosphatase. Brain Research 778 (1):99–106. doi: 10.1016/S0006-8993(97)01042-1.
- Pascente, R., F. Frigerio, M. Rizzi, L. Porcu, M. Boido, J. Davids, M. Zaben, D. Tolomeo, M. Filibian, W. P. Gray, et al. 2016. Cognitive deficits and brain myo-Inositol are early biomarkers of epileptogenesis in a rat model of epilepsy. Neurobiology of Disease 93:146–155. doi: 10.1016/j.nbd.2016.05.001.
- Patrussi, L., S. Mariggiò, D. Corda, and C. Baldari. 2013. The glycerophosphoinositols: From lipid metabolites to modulators of T-cell signaling. Frontiers in Immunology 4 doi: 10.3389/fimmu.2013.00213.
- Paul, C., and D. M. Brady. 2015. Inositol modulation of essential metabolic pathways of insulin resistance in metabolic syndrome, polycystic ovarian syndrome, and type 2 diabetes. Enzyme (GS) 7:16.
- Paulick, M. G., and C. R. Bertozzi. 2008. The Glycosylphosphatidylinositol Anchor: A Complex Membrane-Anchoring Structure for Proteins. Biochemistry 47 (27):6991–7000. doi: 10.1021/bi8006324.
- Pedersen, J. 1952. Diabetes and pregnancy; blood sugar of newborn infants during fasting and glucose administration. Nordisk Medicin 47 (30):1049.
- Pereira, G. R., L. Baker, J. Egler, L. Corcoran, and R. Chiavacci. 1990. Serum myoinositol concentrations in premature infants fed human milk, formula for infants, and parenteral nutrition. The American Journal of Clinical Nutrition 51 (4):589–593. doi: 10.1093/ajcn/51.4.589.
- Pérez-Pérez, A., P. Guadix, J. Maymó, J. L. Dueñas, C. Varone, M. Fernández-Sánchez, and V. Sanchez-Margalet. 2016. Insulin and leptin signaling in placenta from gestational diabetic subjects. Hormone and Metabolic Research = Hormon- Und Stoffwechselforschung = Hormones et Metabolisme 48 (1):62–69. doi: 10.1055/s-0035-1559722.
- Peters, T., B. Chapman, M. Wolfe, and M. Soares. 2000. Placental lactogen-I gene activation in differentiating trophoblast cells: Extrinsic and intrinsic regulation involving mitogen-activated protein kinase signaling pathways. The Journal of Endocrinology 165 (2):443–456. doi: 10.1677/joe.0.1650443.
- Petit, A., G. Guillon, M. Tence, S. Jard, N. Gallo-Payet, D. Bellabarba, J. G. Lehoux, and S. Belisle. 1989. Angiotensin II stimulates both inositol phosphate production and human placental lactogen release from human trophoblastic cells. The Journal of Clinical Endocrinology and Metabolism 69 (2):280–286. doi: 10.1210/jcem-69-2-280.
- Pettitt, T. R., S. K. Dove, A. Lubben, S. D. Calaminus, and M. J. Wakelam. 2006. Analysis of intact phosphoinositides in biological samples. Journal of Lipid Research 47 (7):1588–96. doi: 10.1194/jlr.D600004-JLR200.
- Philipps, L., S. Santhakumaran, C. Gale, E. Prior, K. Logan, M. Hyde, and N. Modi. 2011. The diabetic pregnancy and offspring BMI in childhood: A systematic review and meta-analysis. Diabetologia 54 (8):1957–1966. doi: 10.1007/s00125-011-2180-y.
- Pillai, R. A., Islam M. O., P. Selvam, N. Sharma, A. H. Chu, O. C. Watkins, K. M. Godfrey, R. M. Lewis, and S. Y. Chan 2020. Placental inositol reduced in gestational diabetes as glucose alters inositol transporters and IMPA1 enzyme expression. JCEM. In press
- Pintaudi, B., G. Di Vieste, and M. Bonomo. 2016. The effectiveness of myo-inositol and D-chiro inositol treatment in type 2 diabetes. International Journal of Endocrinology 2016:1–5. doi: 10.1155/2016/9132052.
- Plows, J. F., F. Budin, R. A. Andersson, V. J. Mills, K. Mace, S. T. Davidge, M. H. Vickers, P. N. Baker, I. Silva-Zolezzi, and J. L. Stanley. 2017. The effects of myo-inositol and B and D vitamin supplementation in the db/+ mouse model of gestational diabetes mellitus. Nutrients 9 (2):141. doi: 10.3390/nu9020141.
- Plows, J., J. R. Nieves, F. Budin, K. Mace, C. Reynolds, M. Vickers, I. Silva-Zolezzi, P. Baker, and J. Stanley. 2020. The effects of myo-inositol and probiotic supplementation in a high fat fed preclinical model of glucose intolerance in pregnancy. British Journal of Nutrition 123 (5):516–39. doi: 10.1017/S0007114519003039.
- Poston, L., R. Bell, H. Croker, A. C. Flynn, K. M. Godfrey, L. Goff, L. Hayes, N. Khazaezadeh, S. M. Nelson, E. Oteng-Ntim, et al. 2015. Effect of a behavioural intervention in obese pregnant women (the UPBEAT study): A multicentre, randomised controlled trial. The Lancet. Diabetes & Endocrinology 3 (10):767–777. doi: 10.1016/S2213-8587(15)00227-2.
- Prynne, C. J., A. McCarron, M. E. Wadsworth, and A. M. Stephen. 2010. Dietary fibre and phytate–a balancing act: Results from three time points in a British Birth Cohort. British Journal of Nutrition 103 (2):274–80. doi: 10.1017/S0007114509991644.
- Pugliese, G., R. G. Tilton, A. Speedy, E. Santarelli, D. M. Eades, M. A. Province, C. Kilo, W. R. Sherman, and J. R. Williamson. 1990. Modulation of hemodynamic and vascular filtration changes in diabetic rats by dietary myo-inositol. Diabetes 39 (3):312–22. doi: 10.2337/diab.39.3.312.
- Pundir, J., D. Psaroudakis, P. Savnur, P. Bhide, L. Sabatini, H. Teede, A. Coomarasamy, and S. Thangaratinam. 2018. Inositol treatment of anovulation in women with polycystic ovary syndrome: A meta‐analysis of randomised trials. BJOG: An International Journal of Obstetrics & Gynaecology 125 (3):299–308. doi: 10.1111/1471-0528.14754.
- Quirk, J. G., Jr., and J. E. Bleasdale. 1983. myo-Inositol homeostasis in the human fetus. Obstetrics and Gynecology 62 (1):41–44.
- Rajasekaran, S. S., J. Kim, G.-C. Gaboardi, J. Gromada, S. B. Shears, K. T. dos Santos, E. L. Nolasco, S. d S. Ferreira, C. Illies, M. Köhler, et al. 2018. Inositol hexakisphosphate kinase 1 is a metabolic sensor in pancreatic β-cells. Cellular Signalling 46:120–8. doi: 10.1016/j.cellsig.2018.03.001.
- Reeves, V. L., C. M. Thomas, and E. J. Smart. 2012. Lipid rafts, caveolae and GPI-linked proteins, 3–13. Caveolins and Caveolae: Springer.
- Regidor, P.-A., A. E. Schindler, B. Lesoine, and R. Druckman. 2018. Management of women with PCOS using myo-inositol and folic acid. New clinical data and review of the literature. Hormone Molecular Biology and Clinical Investigation 0 (0) doi: 10.1515/hmbci-2017-0067.
- Roach, J. C., K. Deutsch, S. Li, A. F. Siegel, L. M. Bekris, D. C. Einhaus, C. M. Sheridan, G. Glusman, L. Hood, A. Lernmark, et al. 2006. Genetic mapping at 3-kilobase resolution reveals inositol 1,4,5-triphosphate receptor 3 as a risk factor for type 1 diabetes in Sweden. American Journal of Human Genetics 79 (4):614–27., doi: 10.1086/507876.
- Roll, P., A. Massacrier, S. Pereira, A. Robaglia-Schlupp, P. Cau, and P. Szepetowski. 2002. New human sodium/glucose cotransporter gene (KST1): Identification, characterization, and mutation analysis in ICCA (infantile convulsions and choreoathetosis) and BFIC (benign familial infantile convulsions) families. Gene 285 (1-2):141–148. doi: 10.1016/S0378-1119(02)00416-X.
- Romero, G., and J. Larner. 1993. Insulin mediators and the mechanism of insulin action. Advances in pharmacology. Vol 24, 21–50. Elsevier.
- Rubin, L. J., and C. C. Hale. 1993. Characterization of a Mg-dependent, Na-inositol Co-transport process in cardiac sarcolemmal vesicles. Journal of Molecular and Cellular Cardiology 25 (6):721–731. doi: 10.1006/jmcc.1993.1084.
- Ruchat, S. M., M. F. Hivert, and L. Bouchard. 2013. Epigenetic programming of obesity and diabetes by in utero exposure to gestational diabetes mellitus. Nutrition Reviews 71 (Suppl 1):S88–S94. doi: 10.1111/nure.12057.
- Sacks, D. A., D. R. Hadden, M. Maresh, C. Deerochanawong, A. R. Dyer, B. E. Metzger, L. P. Lowe, D. R. Coustan, M. Hod, J. J. Oats, et al. 2012. Frequency of gestational diabetes mellitus at collaborating centers based on IADPSG consensus panel-recommended criteria: The Hyperglycemia and Adverse Pregnancy Outcome (HAPO) Study. Diabetes Care 35 (3):526–528. doi: 10.2337/dc11-1641.
- Salman, M., J. T. Lonsdale, G. S. Besra, and P. J. Brennan. 1999. Phosphatidylinositol synthesis in mycobacteria. Biochimica et Biophysica Acta 1436 (3):437–450. doi: 10.1016/S0005-2760(98)00151-9.
- Saltiel, A. R. 1990. Second messengers of insulin action. Diabetes Care 13 (3):244–56. doi: 10.2337/diacare.13.3.244.
- Sampson, M., M. Wolf, R. Thadhani, J. L. Ecker, E. W. Seely, C. Lam, S. A. Karumanchi, V. P. Sukhatme, A. S. M. Shakir, A. Daftary, et al. 2004. Preeclampsia and future cardiovascular disease: Potential role of altered angiogenesis and insulin resistance. The Journal of Clinical Endocrinology & Metabolism 89 (12):6239–6243. doi: 10.1210/jc.2004-0548.
- Santamaria, A., A. Alibrandi, A. Di Benedetto, B. Pintaudi, F. Corrado, F. Facchinetti, and R. D’Anna. 2018. Clinical and metabolic outcomes in pregnant women at risk for gestational diabetes mellitus supplemented with myo-inositol: A secondary analysis from 3 RCTs. American Journal of Obstetrics and Gynecology 219 (3):300.e1–300.e6. doi: 10.1016/j.ajog.2018.05.018.
- Santamaria, A., A. Di Benedetto, E. Petrella, B. Pintaudi, F. Corrado, R. D’Anna, I. Neri, and F. Facchinetti. 2016. Myo-inositol may prevent gestational diabetes onset in overweight women: A randomized, controlled trial. The Journal of Maternal-Fetal & Neonatal Medicine 29 (19):3234–3237. doi: 10.3109/14767058.2015.1121478.
- Santamaria, A., D. Giordano, F. Corrado, B. Pintaudi, M. Interdonato, G. D. Vieste, A. D. Benedetto, and R. D'Anna. 2012. One-year effects of myo-inositol supplementation in postmenopausal women with metabolic syndrome. Climacteric15 (5):490–495. doi: 10.3109/13697137.2011.631063.
- Santamaria, A., F. Corrado, G. Baviera, G. Carlomagno, V. Unfer, and R. D’anna. 2016. Second trimester amniotic fluid myo-inositol concentrations in women later developing gestational diabetes mellitus or pregnancy-induced hypertension. The Journal of Maternal-Fetal & Neonatal Medicine 29 (14):2245–2247. doi: 10.3109/14767058.2015.1081886.
- Santamaria, A., F. Corrado, M. L. Interdonato, G. Baviera, G. Carlomagno, P. Cavalli, V. Unfer, and R. D'Anna. 2014. Myo-inositol in Down syndrome amniotic fluid. A case-control study. Prenatal Diagnosis 34 (9):917–8. doi: 10.1002/pd.4398.
- Satake, W., Y. Nakabayashi, I. Mizuta, Y. Hirota, C. Ito, M. Kubo, T. Kawaguchi, T. Tsunoda, M. Watanabe, A. Takeda, et al. 2009. Genome-wide association study identifies common variants at four loci as genetic risk factors for Parkinsons disease. Nature Genetics 41 (12):1303–1307. doi: 10.1038/ng.485.
- Sayers, N., and A. C. Hanyaloglu. 2018. Intracellular follicle-stimulating hormone receptor trafficking and signaling. Frontiers in Endocrinology 9:653. doi: 10.3389/fendo.2018.00653.
- Schaefer, ‐Graf, U., Meitzner, K. Ortega,‐Senovilla, H. Graf, K. Vetter, K. Abou‐Dakn, M. Herrera. and E. 2011. Differences in the implications of maternal lipids on fetal metabolism and growth between gestational diabetes mellitus and control pregnancies. Diabetic Medicine 28 (9):1053–1059. doi: 10.1111/j.1464-5491.2011.03346.x.
- Schaefer-Graf, U. M., K. Graf, I. Kulbacka, S. L. Kjos, J. Dudenhausen, K. Vetter, and E. Herrera. 2008. Maternal lipids as strong determinants of fetal environment and growth in pregnancies with gestational diabetes mellitus. Diabetes Care 31 (9):1858–1863. doi: 10.2337/dc08-0039.
- Schlemmer, U., W. Frølich, R. M. Prieto, and F. Grases. 2009. Phytate in foods and significance for humans: Food sources, intake, processing, bioavailability, protective role and analysis. Molecular Nutrition & Food Research 53 (S2):S330–S375. doi: 10.1002/mnfr.200900099.
- Schneider, S. 2015. Inositol transport proteins. FEBS Letters 589 (10):1049–1058. doi: 10.1016/j.febslet.2015.03.012.
- Schrey, M. P., A. M. Read, and P. J. Steer. 1987. Stimulation of phospholipid hydrolysis and arachidonic acid mobilization in human uterine decidua cells by phorbol ester. The Biochemical Journal 246 (3):705–713. doi: 10.1042/bj2460705.
- Schrey, M. P., J. R. Holt, P. A. Cornford, H. Monaghan, and F. Al-Ubaidi. 1992. Human decidua is a target tissue for bradykinin and kallikrein: Phosphoinositide hydrolysis accompanies arachidonic acid release in uterine decidua cells in vitro. The Journal of clinical Endocrinology and Metabolism 74 (2):426–435. doi: 10.1210/jcem.74.2.1309839.
- Schwartz, R., P. A. Gruppuso, K. Petzold, D. Brambilla, V. Hiilesmaa, and K. A. Teramo. 1994. Hyperinsulinemia and macrosomia in the fetus of the diabetic mother. Diabetes Care 17 (7):640–648. doi: 10.2337/diacare.17.7.640.
- Scioscia, M. 2017. D-chiro inositol phosphoglycans in preeclampsia: Where are we, where are we going? Journal of Reproductive Immunology 124:1–7. doi: 10.1016/j.jri.2017.09.010.
- Scioscia, M., A. Pesci, G. Zamboni, B. Huppertz, and L. Resta. 2013. D-chiro-inositol phosphoglycan expression in human placenta at term in diabetes. Archives of Gynecology and Obstetrics 288 (2):459–60. doi: 10.1007/s00404-013-2729-8.
- Scioscia, M., K. Gumaa, and T. W. Rademacher. 2009. The link between insulin resistance and preeclampsia: New perspectives. Journal of Reproductive Immunology 82 (2):100–5. doi: 10.1016/j.jri.2009.04.009.
- Scioscia, M., K. Gumaa, L. E. Selvaggi, C. H. Rodeck, and T. W. Rademacher. 2009. Increased inositol phosphoglycan P-type in the second trimester in pregnant women with type 2 and gestational diabetes mellitus. Journal of Perinatal Medicine 37 (5):469–471. doi: 10.1515/JPM.2009.082.
- Scioscia, M., K. Gumaa, M. Whitten, L. E. Selvaggi, C. H. Rodeck, and T. W. Rademacher. 2007. Inositol phosphoglycan P-type in healthy and preeclamptic pregnancies. Journal of Reproductive Immunology 76 (1-2):85–90. doi: 10.1016/j.jri.2007.03.016.
- Scioscia, M., K. Gumaa, S. Kunjara, M. A. Paine, L. E. Selvaggi, C. H. Rodeck, and T. W. Rademacher. 2006. Insulin resistance in human preeclamptic placenta is mediated by serine phosphorylation of insulin receptor substrate-1 and-2. The Journal of Clinical Endocrinology & Metabolism 91 (2):709–17. doi: 10.1210/jc.2005-1965.
- Scioscia, M., M. A. Paine, K. Gumaa, C. H. Rodeck, and T. W. Rademacher. 2008. Release of inositol phosphoglycan P-type by the human placenta following insulin stimulus: A multiple comparison between preeclampsia, intrauterine growth restriction, and gestational hypertension. The Journal of Maternal-Fetal & Neonatal Medicine 21 (8):581–5. doi: 10.1080/14767050802199934.
- Scioscia, M., P. J. Williams, K. Gumaa, N. Fratelli, C. Zorzi, and T. W. Rademacher. 2011. Inositol phosphoglycans and preeclampsia: From bench to bedside. Journal of Reproductive Immunology 89 (2):173–177. doi: 10.1016/j.jri.2011.03.001.
- Scioscia, M., S. Kunjara, K. Gumaa, A. M. Gomez Galan, P. McLean, C. H. Rodeck, and T. W. Rademacher. 2007. Altered urinary release of inositol phosphoglycan A-type in women with gestational diabetes mellitus. Gynecologic and Obstetric Investigation 64 (4):217–23. doi: 10.1159/000106494.
- Scioscia, M., S. Kunjara, K. Gumaa, P. McLean, C. Rodeck, and T. Rademacher. 2007. Urinary excretion of inositol phosphoglycan P‐type in gestational diabetes mellitus. Diabetic Medicine 24 (11):1300–1304. doi: 10.1111/j.1464-5491.2007.02267.x.
- Seaquist, E. R., and R. Gruetter. 1998. Identification of a high concentration of scyllo-inositol in the brain of a healthy human subject using 1H- and 13C-NMR . Magnetic Resonance in Medicine 39 (2):313–316. doi: 10.1002/mrm.1910390220.
- Seely, E. W., and C. G. Solomon. 2003. Insulin resistance and its potential role in pregnancy-induced hypertension. The Journal of Clinical Endocrinology and Metabolism 88 (6):2393–2398. doi: 10.1210/jc.2003-030241.
- Shaldubina, A., R. Buccafusca, R. Johanson, G. Agam, R. Belmaker, G. Berry, and Y. Bersudsky. 2007. Behavioural phenotyping of sodium‐myo‐inositol cotransporter heterozygous knockout mice with reduced brain inositol. Genes, Brain and Behavior 6 (3):253–259. doi: 10.1111/j.1601-183X.2006.00253.x.
- Sharma, K., L. Wang, Y. Zhu, A. DeGuzman, G.-Y. Cao, R. B. Lynn, and S. K. Joseph. 1999. Renal type I inositol 1, 4, 5-trisphosphate receptor is reduced in streptozotocin-induced diabetic rats and mice. American Journal of Physiology-Renal Physiology 276 (1):F54–F61. doi: 10.1152/ajprenal.1999.276.1.F54.
- Shears, S. B. 2015. Inositol pyrophosphates: Why so many phosphates? Advances in Biological Regulation 57:203–16. doi: 10.1016/j.jbior.2014.09.015.
- Sherman, W. R., M. A. Stewart, M. M. Kurien, and S. L. Goodwin. 1968. The measurement of myo-inositol, myo-inosose-2 and scyllo-inositol in mammalian tissues. Biochimica et Biophysica Acta (Bba) - General Subjects 158 (2):197–205. doi: 10.1016/0304-4165(68)90131-1.
- Sherman, W. R., M. A. Stewart, P. C. Simpson, and S. L. Goodwin. 1968. The identification of myo-inosose-2 and scyllo-inositol in mammalian tissues. Biochemistry 7 (2):819–824. doi: 10.1021/bi00842a040.
- Sherman, W. R., S. L. Goodwin, and K. D. Gunnell. 1971. neo-Inositol in mammalian tissues. Identification, measurement, and enzymic synthesis from mannose 6-phosphate. Biochemistry 10 (19):3491–3499. doi: 10.1021/bi00795a002.
- Shewan, A., D. J. Eastburn, and K. Mostov. 2011. Phosphoinositides in cell architecture. Cold Spring Harbor Perspectives in Biology 3 (8):a004796. doi: 10.1101/cshperspect.a004796.
- Shimon, H., Y. Sobolev, M. Davidson, V. Haroutunian, R. H. Belmaker, and G. Agam. 1998. Inositol levels are decreased in postmortem brain of schizophrenic patients. Biological Psychiatry 44 (6):428–432. doi: 10.1016/S0006-3223(98)00071-7.
- Shin, J.-A., H.-M. Kwon, K.-H. Han, and H.-Y. Lee. 2012. TonEBP and SMIT expression in human placenta. Anatomy & Cell Biology 45 (3):155–159. doi: 10.5115/acb.2012.45.3.155.
- Siddle, K. 2011. Signalling by insulin and IGF receptors: Supporting acts and new players. Journal of Molecular Endocrinology 47 (1):R1–R10. doi: 10.1530/JME-11-0022.
- Silverstone, P. H., B. M. McGrath, and H. Kim. 2005. Bipolar disorder and myo‐inositol: A review of the magnetic resonance spectroscopy findings. Bipolar Disorders 7 (1):1–10. doi: 10.1111/j.1399-5618.2004.00174.x.
- Simons, K., and R. Ehehalt. 2002. Cholesterol, lipid rafts, and disease. The Journal of Clinical Investigation 110 (5):597–603. doi: 10.1172/JCI16390.
- Soeda, Y., H. Tsuneki, H. Muranaka, N. Mori, S. Hosoh, Y. Ichihara, S. Kagawa, X. Wang, N. Toyooka, Y. Takamura, et al. 2010. The inositol phosphatase SHIP2 negatively regulates insulin/IGF-I actions implicated in neuroprotection and memory function in mouse brain. Molecular Endocrinology (Baltimore, Md.) 24 (10):1965–77. doi: 10.1210/me.2010-0163.
- Solomonia, R., E. Mikautadze, M. Nozadze, N. Kuchiashvili, E. Lepsveridze, and T. Kiguradze. 2010. Myo-inositol treatment prevents biochemical changes triggered by kainate-induced status epilepticus. Neuroscience Letters 468 (3):277–81. doi: 10.1016/j.neulet.2009.11.012.
- Staat, B. C., H. L. Galan, J. E. Harwood, G. Lee, A. M. Marconi, C. L. Paolini, A. Cheung, and F. C. Battaglia. 2012. Transplacental supply of mannose and inositol in uncomplicated pregnancies using stable isotopes. The Journal of Clinical Endocrinology and Metabolism 97 (7):2497–2502. doi: 10.1210/jc.2011-1800.
- Stamler, C. J., D. Breznan, T. A. Neville, F. J. Viau, E. Camlioglu, and D. L. Sparks. 2000. Phosphatidylinositol promotes cholesterol transport in vivo. Journal of Lipid Research 41 (8):1214–21.
- Stanirowski, P. J., D. Szukiewicz, M. Pyzlak, N. Abdalla, W. Sawicki, and K. Cendrowski. 2017. Impact of pre-gestational and gestational diabetes mellitus on the expression of glucose transporters GLUT-1, GLUT-4 and GLUT-9 in human term placenta. Endocrine 55 (3):799–808. doi: 10.1007/s12020-016-1202-4.
- Steegers-Theunissen, R., P. Groenen, and P. Beemster. 2002. Involvement of Inositol in Reproduction. Nutrition Reviews 60 (3):80–87. doi: 10.1301/00296640260042748.
- Steer, T. E., and G. R. Gibson. 2002. The microbiology of phytic acid metabolism by gut bacteria and relevance for bowel cancer. International Journal of Food Science and Technology 37 (7):783–90. doi: 10.1046/j.1365-2621.2002.00616.x.
- Stewart, M. A., W. R. Sherman, and J. T. Harris. 1970. Effects of galactose on levels of free myo‐inositol in rat tissues. Annals of the New York Academy of Sciences 165:609–614.
- Stokes, C. E., and J. N. Hawthorne. 1987. Reduced phosphoinositide concentrations in anterior temporal cortex of Alzheimer-diseased brains. Journal of Neurochemistry 48 (4):1018–1021. doi: 10.1111/j.1471-4159.1987.tb05619.x.
- Stokes, C. E., K. R. W. Gillon, and J. N. Hawthorne. 1983. Free and total lipid myo-inositol concentrations decrease with age in human brain. Biochimica et Biophysica Acta (BBA) - Lipids and Lipid Metabolism 753 (1):136–138. doi: 10.1016/0005-2760(83)90108-X.
- Strange, K., F. Emma, A. Paredes, and R. Morrison. 1994. Osmoregulatory changes in Myo-inositol content and Na+/Myo-inositol contransport in rat cortical astrocytes. Glia 12 (1):35–43. doi: 10.1002/glia.440120105.
- Strieleman, P. J., M. A. Connors, and B. E. Metzger. 1992. Phosphoinositide metabolism in the developing conceptus. Effects of hyperglycemia and scyllo-inositol in rat embryo culture. Diabetes 41 (8):989–997. doi: 10.2337/diab.41.8.989.
- Strieleman, P., and B. E. Metzger. 1993. Glucose and scyllo-inositol impair phosphoinositide hydrolysis in the 10.5-day cultured rat conceptus: a role in dysmorphogenesis? Teratology 48 (3):267–278. doi: 10.1002/tera.1420480310.
- Strieleman, P., M. Connors, and B. E. Metzger. 1992. Phosphoinositide metabolism in the developing conceptus: Effects of hyperglycemia and scyllo-inositol in rat embryo culture. Diabetes 41 (8):989–997. doi: 10.2337/diab.41.8.989.
- Stull, A. J., J. P. Thyfault, M. D. Haub, R. E. Ostlund, Jr, and W. W. Campbell. 2008. Relationships between urinary inositol excretions and whole-body glucose tolerance and skeletal muscle insulin receptor phosphorylation. Metabolism 57 (11):1545–1551. doi: 10.1016/j.metabol.2008.06.009.
- Sun, T-H, D. B. Heimark, T. Nguygen, J. L. Nadler, and J. Larner. 2002. Both myo-inositol to chiro-inositol epimerase activities and chiro-inositol to myo-inositol ratios are decreased in tissues of GK type 2 diabetic rats compared to Wistar controls. Biochemical and Biophysical Research Communications 293 (3):1092–8. doi: 10.1016/S0006-291X(02)00313-3.
- Sussman, I., and F. M. Matschinsky. 1988. Diabetes affects sorbitol and myo-inositol levels of neuroectodermal tissue during embryogenesis in rat. Diabetes 37 (7):974–981. doi: 10.2337/diab.37.7.974.
- Suzuki, S.,. C. Suzuki, Y. Hinokio, Y. Ishigaki, H. Katagiri, M. Kanzaki, V. N. Azev, N. Chakraborty, and M. d'Alarcao. 2014. Insulin-mimicking bioactivities of acylated inositol glycans in several mouse models of diabetes with or without obesity. PloS One 9 (6):e100466. doi: 10.1371/journal.pone.0100466.
- Suzuki, S.,. H. Kawasaki, Y. Satoh, M. Ohtomo, M. Hirai, A. Hirai, S. Hirai, M. Onoda, M. Matsumoto, Y. Hinokio, et al. 1994. Urinary chiro-inositol excretion is an index marker of insulin sensitivity in Japanese type II diabetes. Diabetes Care 17 (12):1465–8., doi: 10.2337/diacare.17.12.1465.
- Suzuki, S.,. Y. Taneda, S. Hirai, S. Abe, A. Sasaki, K-I Suzuki, and T. Toyota. 1991. Molecular mechanism of insulin resistance in spontaneous diabetic GK (Goto-Kakizaki) rats. New Directions in Research and Clinical Works for. Obesity and Diabetes Mellitus :197–203.
- Sweeting, A. N., G. P. Ross, J. Hyett, L. Molyneaux, M. Constantino, A. J. Harding, and J. Wong. 2016. Gestational diabetes mellitus in early pregnancy: Evidence for poor pregnancy outcomes despite treatment. Diabetes Care 39 (1):75–81. doi: 10.2337/dc15-0433.
- Tabrizi, R., V. Ostadmohammadi, K. B. Lankarani, P. Peymani, M. Akbari, F. Kolahdooz, and Z. Asemi. 2018. The effects of inositol supplementation on lipid profiles among patients with metabolic diseases: A systematic review and meta-analysis of randomized controlled trials. Lipids in Health and Disease 17 (1):123. doi: 10.1186/s12944-018-0779-4.
- Taguchi, R., J. Yamazaki, Y. Tsutsui, and H. Ikezawa. 1997. Identification of chiro-inositol and its formation by isomerization of myo-inositol during hydrolysis of glycosylphosphatidylinositol-anchored proteins. Archives of Biochemistry and Biophysics 342 (1):161–168. doi: 10.1006/abbi.1997.9991.
- Tessier, D., Z. Ferraro, and A. Gruslin. 2013. Role of leptin in pregnancy: Consequences of maternal obesity. Placenta 34 (3):205–211. doi: 10.1016/j.placenta.2012.11.035.
- Thomas, M. P., S. J. Mills, and B. V. L. Potter. 2016. The “Other” Inositols and Their Phosphates: Synthesis, Biology, and Medicine (with Recent Advances in myo-Inositol Chemistry). Angewandte Chemie (International ed. In English) 55 (5):1614–1650. doi: 10.1002/anie.201502227.
- Thomas, T. P., E. L. Feldman, J. Nakamura, K. Kato, M. Lien, M. J. Stevens, and D. A. Greene. 1993. Ambient glucose and aldose reductase-induced myo-inositol depletion modulate basal and carbachol-stimulated inositol phospholipid metabolism and diacylglycerol accumulation in human retinal pigment epithelial cells in culture. Proceedings of the National Academy of Sciences 90 (20):9712–6. doi: 10.1073/pnas.90.20.9712.
- Thomas, T. P., F. Porcellati, K. Kato, M. J. Stevens, W. R. Sherman, and D. A. Greene. 1994. Effects of glucose on sorbitol pathway activation, cellular redox, and metabolism of myo-inositol, phosphoinositide, and diacylglycerol in cultured human retinal pigment epithelial cells. The Journal of Clinical Investigation 93 (6):2718–24. doi: 10.1172/JCI117286.
- Thurston, J. H., W. R. Sherman, R. E. Hauhart, and R. F. Kloepper. 1989. myo-Inositol: A Newly Identified Nonnitrogenous Osmoregulatory Molecule in Mammalian Brain. Pediatric Research 26 (5):482–485. doi: 10.1203/00006450-198911000-00024.
- Toh, N., T. Inoue, H. Tanaka, and E. Kimoto. 1987. Polyol accumulation in human placenta and umbilical cord. Biological Research in Pregnancy and Perinatology 8 (1 1ST Half):13–15.
- Tominaga, T.,. R. K. Dutta, D. Joladarashi, T. Doi, J. K. Reddy, and Y. S. Kanwar. 2016. Transcriptional and translational modulation of myo-inositol oxygenase (Miox) by fatty acids Implications in renal tubular injury induced in obesity and diabetes. The Journal of Biological Chemistry 291 (3):1348–67. doi: 10.1074/jbc.M115.698191.
- Trama, J., Q. Lu, R. G. Hawley, and S. N. Ho. 2000. The NFAT-related protein NFATL1 (TonEBP/NFAT5) is induced upon T cell activation in a calcineurin-dependent manner. The Journal of Immunology 165 (9):4884–4894. doi: 10.4049/jimmunol.165.9.4884.
- Traynor-Kaplan, A., M. Kruse, E. J. Dickson, G. Dai, O. Vivas, H. Yu, D. Whittington, and B. Hille. 2017. Fatty-acyl chain profiles of cellular phosphoinositides. Biochimica et Biophysica Acta (Bba) - Molecular and Cell Biology of Lipids 1862 (5):513–22. doi: 10.1016/j.bbalip.2017.02.002.
- Trocino, R. A., S. Akazawa, M. Ishibashi, K. Matsumoto, H. Matsuo, H. Yamamoto, S. Goto, Y. Urata, T. Kondo, and S. Nagataki. 1995. Significance of glutathione depletion and oxidative stress in early embryogenesis in glucose-induced rat embryo culture. Diabetes 44 (8):992–8. doi: 10.2337/diab.44.8.992.
- Troyer, D., D. Schwertz, J. Kreisberg, and M. A. Venkatachalam. 1986. Inositol phospholipid metabolism in the kidney. Annual Review of Physiology 48:51–71. doi: 10.1146/annurev.ph.48.030186.000411.
- Tsai, Y.-H., X. Liu, and P. H. Seeberger. 2012. Chemical biology of glycosylphosphatidylinositol anchors. Angewandte Chemie (International ed. In English) 51 (46):11438–56. doi: 10.1002/anie.201203912.
- Turner, D. I., N. Chakraborty, and M. d'Alarcao. 2005. A fluorescent inositol phosphate glycan stimulates lipogenesis in rat adipocytes by extracellular activation alone. Bioorganic & Medicinal Chemistry Letters 15 (8):2023–5. doi: 10.1016/j.bmcl.2005.02.061.
- Uhl, O., H. Demmelmair, M. T. Segura, J. Florido, R. Rueda, C. Campoy, and B. Koletzko. 2015. Effects of obesity and gestational diabetes mellitus on placental phospholipids. Diabetes Research and Clinical Practice 109 (2):364–371. doi: 10.1016/j.diabres.2015.05.032.
- Unfer, V., and G. Porcaro. 2014. Updates on the myo-inositol plus D-chiro-inositol combined therapy in polycystic ovary syndrome. Expert Review of Clinical Pharmacology 7 (5):623–31. doi: 10.1586/17512433.2014.925795.
- Unfer, V., F. Facchinetti, B. Orrù, B. Giordani, and J. Nestler. 2017. Myo-inositol effects in women with PCOS: A meta-analysis of randomized controlled trials. Endocrine Connections 6 (8):647–658. doi: 10.1530/EC-17-0243.
- Unfer, V., G. Carlomagno, G. Dante, and F. Facchinetti. 2012. Effects of myo-inositol in women with PCOS: A systematic review of randomized controlled trials. Gynecological Endocrinology: The Official Journal of the International Society of Gynecological Endocrinology 28 (7):509–15. doi: 10.3109/09513590.2011.650660.
- Valluru, R., and W. Van den Ende. 2011. Myo-inositol and beyond-emerging networks under stress. Plant Science: An International Journal of Experimental Plant Biology 181 (4):387–400. doi: 10.1016/j.plantsci.2011.07.009.
- Van Sande, J., D. Dequanter, P. Lothaire, C. Massart, J. E. Dumont, and C. Erneux. 2006. Thyrotropin stimulates the generation of inositol 1, 4, 5-trisphosphate in human thyroid cells. The Journal of Clinical Endocrinology and Metabolism 91 (3):1099–107. doi: 10.1210/jc.2005-1324.
- Varela-Nieto, I., Y. León, and H. N. Caro. 1996. Cell signalling by inositol phosphoglycans from different species. Comparative Biochemistry and Physiology Part B: Biochemistry and Molecular Biology 115 (2):223–41. doi: 10.1016/0305-0491(96)00087-9.
- Vernon, D. M., and H. J. Bohnert. 1992. A novel methyl transferase induced by osmotic stress in the facultative halophyte Mesembryanthemum crystallinum. The EMBO Journal 11 (6):2077–85. doi: 10.1002/j.1460-2075.1992.tb05266.x.
- Vernon, R. G. 2005. Lipid metabolism during lactation: A review of adipose tissue-liver interactions and the development of fatty liver. The Journal of Dairy Research 72 (4):460–9. doi: 10.1017/S0022029905001299.
- Villalba, M., K. L. Kelly, and J. M. Mato. 1988. Inhibition of cyclic AMP-dependent protein kinase by the polar head group of an insulin-sensitive glycophospholipid. Biochimica et Biophysica Acta (Bba) - Molecular Cell Research 968 (1):69–76. doi: 10.1016/0167-4889(88)90045-6.
- Vinggaard, A. M., and H. S. Hansen. 1995. Characterization and partial purification of phospholipase D from human placenta. Biochimica et Biophysica Acta 1258 (2):169–176. doi: 10.1016/0005-2760(95)00121-R.
- Vitagliano, A., G. Saccone, E. Cosmi, S. Visentin, F. Dessole, G. Ambrosini, and V. Berghella. 2019. Inositol for the prevention of gestational diabetes: A systematic review and meta-analysis of randomized controlled trials. Archives of Gynecology and Obstetrics 299 (1):55–68. doi: 10.1007/s00404-018-5005-0.
- Vivien, D., E. Petitfrère, L. Martiny, H. Sartelet, P. Galéra, B. Haye, and J.-P. Pujol. 1993. IPG (inositolphosphate glycan) as a cellular signal for TGF-β1 modulation of chondrocyte cell cycle. Journal of Cellular Physiology 155 (3):437–44. doi: 10.1002/jcp.1041550302.
- Voglmayr, J., and I. White. 1971. Synthesis and metabolism of myoinositol in testicular and ejaculated spermatozoa of the ram. Journal of Reproduction and Fertility 24 (1):29–37. doi: 10.1530/jrf.0.0240029.
- Walsh, S. W., and V. M. Parisi. 1986. The role of arachidonic acid metabolites in preeclampsia. Paper presented at: Seminars in perinatology.
- Wang, X., S. P. Devaiah, W. Zhang, and R. Welti. 2006. Signaling functions of phosphatidic acid. Progress in Lipid Research 45 (3):250–278. doi: 10.1016/j.plipres.2006.01.005.
- Watkins, O. C., M. O. Islam, P. Selvam, R. A. Pillai, A. Cazenave-Gassiot, A. K. Bendt, N. Karnani, K. M. Godfrey, R. M. Lewis, M. R. Wenk, et al. 2019. Inositol alters 13C-labeled fatty acid metabolism in human placental explants. Journal of Endocrinology 243 (1):73–84., doi: 10.1530/JOE-19-0267.
- Wells, I. C., and J. Hogan. 1968. Effects of dietary deficiencies of lipotropic factors on plasma cholesterol esterification and tissue cholesterol in rats. The Journal of Nutrition 95 (1):55–62. doi: 10.1093/jn/95.1.55.
- Wells, W. W., T. A. Pittman, and H. J. Wells. 1965. Quantitative analysis of myoinositol in rat tissue by gas-liquid chromatography. Analytical Biochemistry 10 (3):450–458. doi: 10.1016/0003-2697(65)90314-3.
- Wenk, M. R., L. Lucast, G. Di Paolo, A. J. Romanelli, S. F. Suchy, R. L. Nussbaum, G. W. Cline, G. I. Shulman, W. McMurray, and P. De Camilli. 2003. Phosphoinositide profiling in complex lipid mixtures using electrospray ionization mass spectrometry. Nature Biotechnology 21 (7):813–7. doi: 10.1038/nbt837.
- Wentzel, P., and U. J. Eriksson. 2020. Embryopathy and Diabetes. Gestational Diabetes. Vol 28: Karger Publishers; 132–44.
- Wentzel, P., C. R. Wentzel, M. B. Gäreskog, and U. J. Eriksson. 2001. Induction of embryonic dysmorphogenesis by high glucose concentration, disturbed inositol metabolism, and inhibited protein kinase C activity. Teratology 63 (5):193–201. doi: 10.1002/tera.1034.
- Wentzel, P., N. Welsh, and U. J. Eriksson. 1999. Developmental damage, increased lipid peroxidation, diminished cyclooxygenase-2 gene expression, and lowered prostaglandin E2 levels in rat embryos exposed to a diabetic environment. Diabetes 48 (4):813–20. doi: 10.2337/diabetes.48.4.813.
- Werner, E. F., P. Has, G. Tarabulsi, J. Lee, and A. Satin. 2016. Early postpartum glucose testing in women with gestational diabetes mellitus. Obstetrical & Gynecological Survey 71:697–699. doi: 10.1097/01.ogx.0000510809.66411.87.
- White, D. 1973. The phospholipid composition of mammalian tissues. Form and Function of Phospholipids 441–82.
- Wilcox, R. A., W. U. Primrose, S. R. Nahorski, and R. A. J. Challiss. 1998. New developments in the molecular pharmacology of the myo-inositol 1,4,5-trisphosphate receptor. Trends in Pharmacological Sciences 19 (11):467–75. doi: 10.1016/S0165-6147(98)01260-7.
- Williams, P. J., K. Gumaa, M. Scioscia, C. W. Redman, and T. W. Rademacher. 2007. Inositol phosphoglycan P-type in preeclampsia: a novel marker. Hypertension 49 (1):84–89. ? doi: 10.1161/01.HYP.0000251301.12357.ba.
- Willmroth, F., T. Drieling, U. Lamla, M. Marcushen, H.-J. Wark, and D. Van Calker. 2007. Sodium-myo-inositol co-transporter (SMIT-1) mRNA is increased in neutrophils of patients with bipolar I disorder and down-regulated under treatment with mood stabilizers. The International Journal of Neuropsychopharmacology 10 (1):63–71. doi: 10.1017/S1461145705006371.
- Witters, L., and B. Kemp. 1992. Insulin activation of acetyl-CoA carboxylase accompanied by inhibition of the 5'-AMP-activated protein kinase. The Journal of Biological Chemistry 267 (5):2864–7.
- Wolfson, M., Y. Bersudsky, E. Zinger, M. Simkin, R. Belmaker, and L. Hertz. 2000. Chronic treatment of human astrocytoma cells with lithium, carbamazepine or valproic acid decreases inositol uptake at high inositol concentrations but increases it at low inositol concentrations. Brain Research 855 (1):158–61. doi: 10.1016/S0006-8993(99)02371-9.
- Xie, P., L. Sun, P. J. Oates, S. K. Srivastava, and Y. S. Kanwar. 2010. Pathobiology of renal specific oxidoreductase/myo-inositol oxygenase in diabetic nephropathy: Its implications in tubulo-interstitial fibrosis. American Journal of Physiology-Heart and Circulatory Physiology
- Yamashita, T., M. Tamatani, M. Taniguchi, T. Takagi, T. Yoshimine, and M. Tohyama. 1998. Regulation of Na+/myo-inositol cotransporter gene expression in hyperglycemic rat hippocampus. Brain Research. Molecular Brain Research 57 (1):167–72. doi: 10.1016/S0169-328X(98)00078-3.
- Yamazaki, H., K. C. Zawalich, and W. S. Zawalich. 2010. Physiologic implications of phosphoinositides and phospholipase C in the regulation of insulin secretion. Journal of Nutritional Science and Vitaminology 56 (1):1–8. doi: 10.3177/jnsv.56.1.
- Yorek, M. A., J. A. Dunlap, and W. L. Lowe. 1998. Opposing effects of tumour necrosis factor α and hyperosmolarity on Na+/myo-inositol co-transporter mRNA levels and myo-inositol accumulation by 3T3-L1 adipocytes. Biochemical Journal 336 (2):317–25. doi: 10.1042/bj3360317.
- Yue, D. K., S. McLennan, E. Fisher, S. Heffernan, C. Capogreco, G. R. Ross, and J. R. Turtle. 1989. Ascorbic acid metabolism and polyol pathway in diabetes. Diabetes 38 (2):257–61. doi: 10.2337/diab.38.2.257.
- Zeitler, P., and S. Handwerger. 1985. Arachidonic acid stimulates phosphoinositide hydrolysis and human placental lactogen release in an enriched fraction of placental cells. Molecular Pharmacology 28:549–554.
- Zeitler, P., E. Markoff, and S. Handwerger. 1983. Characterization of the synthesis and release of human placental lactogen and human chorionic gonadotropin by an enriched population of dispersed placental cells. The Journal of Clinical Endocrinology and Metabolism 57 (4):812–818. doi: 10.1210/jcem-57-4-812.
- Zeitler, P., Y. Q. Wu, and S. Handwerger. 1991. Melittin stimulates phosphoinositide hydrolysis and placental lactogen release: Arachidonic acid as a link between phospholipase A2 and phospholipase C signal-transduction pathways. Life Sciences 48 (21):2089–2095. doi: 10.1016/0024-3205(91)90166-9.
- Zerbino, D. R., P. Achuthan, W. Akanni, M. R. Amode, D. Barrell, J. Bhai, K. Billis, C. Cummins, A. Gall, C. G. Girón, et al. 2018. Ensembl 2018. Nucleic Acids Research 46 (D1):D754–D761. doi: 10.1093/nar/gkx1098.
- Zhan, M., I. M. Usman, L. Sun, and Y. S. Kanwar. 2015. Disruption of renal tubular mitochondrial quality control by Myo-inositol oxygenase in diabetic kidney disease. Journal of the American Society of Nephrology : JASN 26 (6):1304–21. doi: 10.1681/ASN.2014050457.
- Zhang, H., and C. Zhang. 2010. Adipose “talks” to distant organs to regulate insulin sensitivity and vascular function. Obesity (Silver Spring. Md) 18:2071.
- Zhang, H., Y. Lv, Z. Li, L. Sun, and W. Guo. 2019. The efficacy of myo-inositol supplementation to prevent gestational diabetes onset: A meta-analysis of randomized controlled trials. The Journal of Maternal-Fetal & Neonatal Medicine32 (13):2249–2255. doi: 10.1080/14767058.2018.1428303.
- Zheng, X., Z. Liu, Y. Zhang, Y. Lin, J. Song, L. Zheng, and S. Lin. 2015. Relationship between myo-inositol supplementary and gestational diabetes mellitus: A meta-analysis. Medicine 94 (42):e1604. doi: 10.1097/MD.0000000000001604.
- Zhu, X., and J. Eichberg. 1990. A myo-inositol pool utilized for phosphatidylinositol synthesis is depleted in sciatic nerve from rats with streptozotocin-induced diabetes. Proceedings of the National Academy of Sciences 87 (24):9818–22. doi: 10.1073/pnas.87.24.9818.
- Zhuo, Z.,. A. Wang, and H. Yu. 2014. Effect of metformin intervention during pregnancy on the gestational diabetes mellitus in women with polycystic ovary syndrome: a systematic review and meta-analysis. Journal of Diabetes Research 2014:381231. doi: 10.1155/2014/381231.
- Ziyadeh, F. N. 2004. Mediators of diabetic renal disease: The case for TGF-β as the major mediator. Journal of the American Society of Nephrology 15 (90010):55S–S57. doi: 10.1097/01.ASN.0000093460.24823.5B.