ABSTRACT
This study aimed to apply the solvent-free microwave extraction (SFME) for isolating the essential oil from the rhizomes of cassumunar ginger and study the kinetic mechanism during the extraction process by light microscopy. A central composite design was employed to evaluate the influence of the key variables including microwave power and irradiation time on the oil recovery. The optimal process conditions of SFME were irradiation of the sample at 700 W for 70 min. Microwave power had the most significant effect on the oil recovery (p < 0.001), followed by irradiation time (p < 0.01). The major volatile constituents in the oil obtained under the optimal conditions were sabinene, terpinen-4-ol, and (E)-1-(3,4-dimethoxyphenyl) butadiene with a higher amount of the oxygenated compounds compared to those isolated by a conventional hydrodistillation (HD). Isolating the essential oil by SFME showed a complete extraction with higher plant cell rupture and was quicker than HD.
1. Introduction
The search of green extraction technology with superior efficiency (ie cost-effective, sustainable and capable of producing the essential oil with the same characteristics) and more environment friendly (ie reduction of CO2 emission and waste water) is very important in all countries worldwide to meet the challenges of the 21st century (Citation1–Citation3). The solvent-free microwave extraction (SFME), an innovative clean and efficient technique, has recently gained popularity for isolating the essential oils of different plant parts (bark, flower, leaf) of many scented plants (Citation2–Citation8). The principle in the extraction of this technique combines both microwave heating and dry-distillation for extraction the volatiles at the atmospheric pressure (Citation7). In SFME processes, the in-situ water present inside the tissue of the fresh plant material or a small amount of water added to the dry samples is heated by microwave energy due to dipole rotation, followed by increasing the localized high pressure, which leads to the bursting of the oil cells and the evaporation of the essential oil by azeotropic distillation (Citation8). Classical extraction techniques like hydrodistillation (HD), steam distillation and solvent extraction are high energy-consuming methods with long processing times, solvent consumption or toxic solvent residues (Citation3), which consequently induce further costs (Citation7).
One of the important traditionally used aromatic plants in tropical Asian countries especially Bangladesh, Cambodia, India, Malaysia, Myanmar, Laos and Thailand with increasing demand at international markets for medicinal, cosmetic and aromatherapy utilization, is cassumunar ginger [Zingiber montanum (J.Koenig) Link ex A.Dietr., Zingiberaceae family] (Citation9–Citation11). The fresh rhizomes where the numerous oil globules are located, contain 0.57–2.10% v/w essential oil, mainly consisting of sabinene (25–45%), γ-terpinene (5–10%), α-terpinene (2–5%), terpinen-4-ol (25–45%), (E)-1-(3,4-dimethoxyphenyl) butadiene (DMPBD) (1–10%) (Citation12–Citation14) that exhibit the strong anti-inflammatory activity (Citation9,Citation15–Citation19). Because of the potential use of the oil in pharmaceutical industry as well as its well-documented clinical efficacy, the Thai National Drug Committee has approved pharmaceutical products containing cassumunar ginger oil as an alternative source of non-steroidal anti-inflammatory drugs for sprains and muscular aches (Citation20). Many commercial products containing cassumunar ginger oil for muscle pain relief are available on the market. The characteristic pleasant odor with earthy, camphoraceous features and relaxation property also makes the cassumunar ginger oil being of interest among aroma-therapists for Thai massage and spa applications (Citation21). However, the cassumunar ginger oil sold in the market is generally extracted by using conventional methods (hydro- and steam distillation) (information obtained from factory visits). Therefore, it is very important to find out a fast and economic method capable of acquiring a highly pure essential oil from the cassumunar ginger rhizomes. The isolation of cassumunar ginger oil using the SFME has not been reported yet.
In continuation of an on-going work of the authors’ group related to the extraction of the potential natural products for health promotion, this study mainly aimed to apply the SFME for the isolation of the essential oil from cassumunar ginger rhizomes. The two key operating factors (microwave power and irradiation time) of the selected method influencing the essential oil yield were optimized by application of the central composite design (CCD) to obtain the highest possible yield of the target oil. This technique has given a large amount of information without the need of numerous experimental trials and provided the data to evaluate the interaction effects of the test factors and the optimum conditions for the desired responses (Citation22–Citation24). Moreover, the extraction mechanism of the method used was investigated by a microscopic technique to understand the action of the microwave irradiation in more details. Additionally, other quality properties of the isolated essential oil (ie volatile compositions, specific gravity, refractive index and solubility) were determined and compared to those of an essential oil extracted by a conventional HD.
2. Materials and methods
2.1. Materials
The fresh 2-year-old rhizomes of cassumunar ginger were supplied by the traditional Thai Medicine Center, Phana Government Hospital, Amnaj Charoen Province, Thailand. The plant was authenticated by Dr. Bancha Yingngam with the voucher specimen (BCY UBU 2,016,031) deposited at the Herbarium of Ubon Ratchathani University. All chemicals and solvents used were of either analytical or gas chromatographic grade and purchased from Sigma Chemical Co. (St. Louis, MO, USA).
2.2. Sample preparations
The fresh cassumunar ginger rhizomes were washed with tap water and allowed to air-dry at 25 ± 1°C overnight. Prior to perform the essential oil extraction, the rhizomes were sliced and their quality was checked by drying some plant sample in a hot air oven at 50°C for 24 h to obtain moisture content of 10% or below, followed by grinding into a coarse powder. The volatile content was determined according to the method described in the Thai Herbal Pharmacopoeia (THP) (Citation25). In this study, the yield of volatile oil in the test sample was higher than the minimum quantity mentioned in THP (not less than 2.00% v/w). Consequently, the fresh rhizomes containing about 80% moisture were sliced into small pieces and the extraction procedure of the volatiles was performed immediately to minimize the loss of essential oil.
2.3. SFME
The essential oil was isolated by the SFME using a microwave equipped with a Clevenger apparatus (2.45 GHz, MAS-II, Sineo Microwave Chemistry Technology Co., Ltd, Shanghai, China). Briefly, slices (200 g) of fresh plant material were put into a glass flask inside the microwave chamber, which was connected to a Clevenger apparatus and condenser. The microwave power and irradiation time were performed at atmospheric pressure according to the design of experiment (see section: Experimental design). The essential oils obtained were dehydrated with anhydrous sodium sulfate and kept at 4°C for further analysis. Three separate measurements were performed in all experiments.
2.4. Experimental design
Two operational variables influencing the yield of cassumunar ginger oil, including microwave power (X1) and irradiation time (X2), were investigated. The response variable was essential oil yield (Y). A 4–factorial, 4–axial 5–centered CCD based response surface methodology was employed in the thirteen randomized experiments to develop a response surface and the optimum condition, study their effects on the desired dependent variable, calculate the experimental error and prove the suitability of the model. Five levels of the independent variables were coded at – 1.41, – 1, 0, + 1 and + 1.41 for statistical analysis. The experimental values were analyzed by the multiple regressions through the least square method and the results were fitted with a polynomial model following equation (Eq. 1)
Y is the yield of essential oil expressed as mL/100 g of initial fresh matter. β0, βi, βii and βji represent the constant coefficients of intercept, linear, quadratic and interactive terms, respectively. Xi and Xj are the independent variables (microwave power and irradiation time). The adequacy of the models was also tested by the predicted R2, adjusted R2, and coefficient of variance. The relationship between the independent and response variables was constructed as the three- and two-dimensional plots by employing the fitted model obtained.
2.5. Process optimization and validation
The optimum condition of SFME should provide the highest yield of the target oil with minimizing the extraction time. Thus, a desirability function (d) for the simultaneous optimization of the response was applied to calculate the desired conditions of the model as follows (Eq. 2):
N, ri and m are the numbers of response, the importance of a particular response and partial desirability function for a specific response, respectively. The value of d varies in the range of 0 ≤ d ≥ 1. The average experimental response obtained was compared to the predicted value.
2.6. Conventional HD
The conventional HD of the essential oil was also conducted using a Clevenger-type apparatus according to the THP (Citation25) with some modifications. A quantity of 200 g sliced plant material was mixed with 700 mL distilled water and introduced to HD process for 5 h until the volume of essential oil kept constant. The essential oil was collected in the same way as in SFME for further analyses.
2.7. Analyses
2.7.1. Essential oil yield
The yield of cassumunar ginger essential oil isolated from the rhizomes by the SFME and HD method was expressed in term of the volume of the oil collected (mL) per 100 g of initial fresh plant material (% v/w).
2.7.2. Identification of the volatile components
The volatile components in the essential oils obtained from both the SFME and HD were analyzed by gas chromatography-mass spectrometry (GC–MS) (Agilent 7890A and 5975C Mass Selective Detector; Agilent Technologies, Palo Alto, CA, USA) according to the previously reported method (Citation23). A fused silica capillary column 5% phenylmethylsiloxane (HP-5MS, 30 m long × 250 μm ID, 0.25 μm film thickness, Agilent J&W, Lawrence, Kansas, USAAQ: Please supply the missing state and city names here.) was applied as stationary phase. The following chromatographic conditions were used: 60°C to 270°C at 3°C/min; carrier gas helium; flow rate 1 mL/min; injection volume of the sample (cassumunar ginger essential oil in hexane) 1 μL; split ratio 1:50; injection temperature 240°C; detection temperature 280°C. The volatile composition of the samples was identified by comparison of their retention indices relative to a homologous series of C8–C20 alkanes on HP-5MS capillary column and those values reported in the literature and the mass spectra database (NIST 08 MS and Wiley Libraries). The percentage composition of the essential oil was calculated using the normalization method from peak areas of the total oil.
2.7.3. Light/fluorescent microscope image analysis
The microstructure changes in the cassumunar ginger sample (control and spent after extraction) were studied with a Nikon Eclipse E200 light/fluorescent microscope connected with NIS-Elements BR 4.50 software (Nikon Instruments Inc., Japan). A transverse section of samples was stained with a mixture solution of alcian blue and safranin O (3:2, v/v) and examined for their microstructure alterations under a light/fluorescent microscope. The micrographs were carried out at 40× magnification.
2.7.4. Physical properties of the essential oil
The physical properties of the essential oils isolated from the SFME and a conventional HD were measured. Specific gravity was determined by weighing the samples on a highly sensitive balance (accuracy of ± 0.00001 g) (Mettler Toledo, Im Langacher, Greifensee, SwitzerlandAQ: Please supply the missing city and state names here.). The specific gravity value was then calculated by dividing the weight of 10 µL essential oil by that of 10 µL distilled water. Refractive index measured by the RFM 330 refractometer (Bellingham Stanley Ltd., Kent, England). The solubility of the samples was measured by repeated dilution of 1 mL essential oil with 0.1 mL 80% ethanol until a clear mixture was obtained. All measurements were performed at 20 ± 1°C.
2.8. Statistical analyses
The results are expressed as the mean ± standard deviation of three measurements. The data obtained from CCD were analyzed by analysis of variance for the polynomial model and the accuracy of the regression model. P < 0.05 was considered to be a minimum of statistically significant differences among groups.
3. Results and discussion
3.1. Fitting the model
Determining the accurate optimum conditions of the experimental factors and the interrelation among the multi-factors is limited using the one-factor-at-a-time methodology (Citation22–Citation24,Citation26). Thus, the CCD was applied to the key operating factors for achieving the maximum yield of essential oil and the obtained results are presented in . The essential oil yields varied between 0 and 1.31% (v/w) depending on the operated SFME conditions. Experiment number 6 had the highest essential oil yield; whereas, the lowest yield was found in the experiment numbers 1 and 5. These observations were in conformity with an earlier report for cassumunar ginger oil isolated from the fresh plant material (Citation12) and their variation was due to the extraction conditions applied.
Table 1. The matrix of CCD applied for isolation of the essential oil from cassumunar ginger rhizomes.
Prior to evaluating the key factors influencing the yields of essential oil, the deleted studentized residuals after model refinement were analyzed to check the normal distribution of the residuals. The results are shown in . The values of residuals fit on a straight line to a major extent with a slight scattering of some points of the experimental numbers. This result confirmed that the experimental data were normally distributed and capable to give accurate data. A regression analysis was then applied to the obtained experimental data to fit the mathematical model. The quadratic model was suitable to determine the optimum conditions for extraction of the cassumunar ginger oil and the obtained mathematical equation that predicted the essential oil yield was represented as follows (Eq. 3):
Figure 1. Normal probability plot showing the value of studentized residuals after model refinement. The numbers in the graph indicate the experimental numbers in .
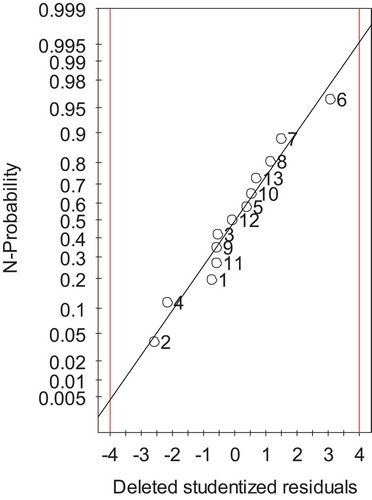
Yield of essential oil
was plotted to illustrate the relative importance of the effects and rank the factors influencing the oil yield. The linear terms of microwave power and irradiation time (and
) exhibited a positive effect on the oil yield that is contrary to a negative effect of quadratic terms for both independent factors (
and
). There was no interaction between the microwave power and the irradiation time (
) on the oil extracting yield (p > 0.05). Thus, changing the microwave power and irradiation time had highly influence on the isolated oil yield.
Figure 2. The scaled and centered coefficient plot after model refinement showing the influence of the test factors ( microwave power,
irradiation time) on the yield of cassumunar ginger oil.
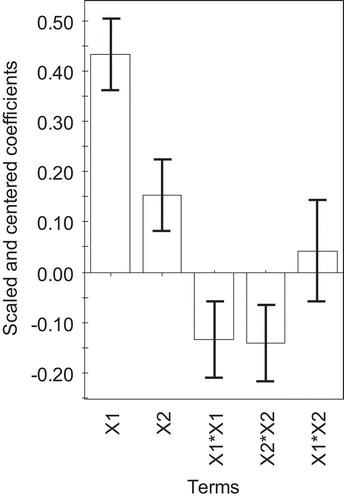
The goodness of fit and adequacy of the developed model was assessed by application of the analysis of variance. Ideally, the model with good data prediction should have the absolute F-value higher than the tabulated value of F-distribution at a level of significance α (Citation2,Citation23,Citation24). As summarized in , the Fisher variation ratio (the ratio between the mean square of the model and the residual error of the experimental values, F-value) was 48.87, indicating the significant model (p < 0.0001). There was only a 0.01% chance that a model F-value could occur due to noise. In this case, this result confirmed model reliability.
Table 2. ANOVA statistics showing the effect of microwave power and irradiation time on the yield of essential oil.
Moreover, the correlation coefficient () and the lack-of-fit value were also evaluated to check the quality of the proposed model. As depicted in , the experimental values of the essential oil yields obtained close to a straight line of the predicted values with the
and adjusted-R2 values were 0.9722 and 0.9523, respectively. This means that the presented model had the capability to estimate the oil yield because the resulting R2 values are higher than 0.75 (Citation23,Citation26). The p-value of lack-of-fit is higher than 0.05 (p = 0.0579, non-significant) and could imply that the assumption of the constant variance was satisfied. The value of an adequate precision that measures the signal-to-noise ratio was 20.40, reinforcing an adequate signal due to the fact that a ratio higher than 4 is desirable. The values of standard deviation and coefficient of variation (CV) were 0.09 and 13.10%, respectively. These results indicate a good precision of the experimental and predicted values. Therefore, it could be concluded that the constructed model could be used to navigate the design space.
3.2. Response surface analyses
The response surface three-dimensional and contour profiles for the oil yield as a function of microwave power and irradiation time are illustrated in . The oil yield tremendously changed with increasing microwave power at a given irradiation time (). The recovery of oil yield reached a peak value [1.20% (v/w)] at 700 W microwave power and 70 min irradiation time. No further rise in the oil yield was observed even if the microwave power was increased to a value higher than 700 W. Moreover, more details were visualized in the two-dimensional contour plot in . The oil yield increased when the microwave power was increased from 100 W to 700 W, as well as the irradiation time from 8 min to 70 min. A similar tendency was reported in literature for Dryopteris fragrans: the quantity of the plant oil mainly depends on microwave power and irradiation time (Citation27). This result can be explained by the in-situ water in the plant cells, which absorb microwave energy, favorable to plant cell swelling and increasing the driving force of mass transfer. However, further irradiating of the plant sample after 90 min tended to diminish the oil yield, probably due to either partial thermal degradation or hydrolysis of some volatile molecules and volatilization (Citation2,Citation23,Citation28). Thus, a moderate microwave power (≤ 700 W) and shorter irradiation time (≤ 90 min) should be chosen as an appropriate condition to increase the extraction yield.
3.3. Optimization of the SFME for the essential oil isolation and model validation
The test factors were simultaneously considered in the optimization process by solving Eq. (2) and Eq. (3). The results are shown in . Based on the desirability function technique, the SFME conditions for obtaining the maximum oil yield were 700 W microwave power and 70 min irradiation time with a high desirability function value of 0.94. This optimal point located within the operating parameter ranges and additional three experiments were conducted to verify the accuracy of the model. Under the SFME conditions obtained by this process, the experiment value [1.21 ± 0.10% (v/w)] of the oil yield was consistent with that of the predicted value [1.22 ± 0.11% (v/w)] (p > 0.05). The high correlation between the experimental and the predicted results confirmed that the resulting model had the accuracy to predict the response value.
3.4. Comparison of SFME and HD
The SFME was applied for the isolation of cassumunar ginger oil to minimize the waste water of the process and reduce processing time. In the present work, the oil isolated from the matrices of cassumunar ginger using SFME showed a reduced extraction time (70 min) compared to conventional HD (5 h). This was due to the different fundamentals of the SFME and HD. In SFME, both, the mass and the heat transfer mostly occur from inside to outside under microwave irradiation. Moreover, heat transfer partly occurs also from outside to inside of the plant cells. In the HD, the heat transfer occurs from outside to inside the plant matrices, which is opposite to the direction of the mass transfer of the essential oil (Citation2,Citation3). Additionally, the temperature inside the extraction flask of the HD process increased much slower than the SFME. Therefore, a synergistic combination of the heat and mass gradients occurring in the SFME process resulted in enhancing the overall rate of essential oil extraction. Literature data reported of other aromatic plants extracted by SFME supported our findings (Citation4,Citation6).
3.5. Chemical composition of essential oil
The volatile compounds in the essential oil obtained by the SFME at the optimal conditions were qualitatively analyzed by GC-MS. A total of 29 volatile substances were identified from cassumunar ginger essential oil (). Sabinene, terpinen-4-ol and DMPBD were the major components in the oil extracted by SFME, accounting for 17.80, 20.81 and 28.62%, respectively (). Some differences were clearly found based on the peak area obtained from GC analysis. SFME produced a higher concentration of oxygenated compounds compared to the HD. Similar findings have been reported for the extraction of essential oil from Amomum tsao-ko (Citation2). This leads to the conclusion that the presence of oxygenated compounds in the oil exhibits different behavior compared to the non-oxygenated groups of compounds when the oil is extracted from plant material by SFME. The oxygenated compounds have a higher dipolar moment with a higher microwave power absorption and easier extraction than monoterpenes or sesquiterpenes with a lower dipolar moment (Citation29).
Table 3. Chemical composition of essential oil extracted from cassumunar ginger rhizomes using the optimized SFME condition and HD.
3.6. Mechanism of SFME on boosting the essential oil yield
More details of the extraction mechanism of SFME were studied by microscopic technique. shows the normal tissue of the untreated cassumunar ginger rhizomes. The untreated plant material consisted of epidermal cells having rectangular and elongated cells, followed by thin walled, irregular elongated, 6–10 layered cork cells. The cortex compartment had several layers of parenchymatous cells with intercellular air spaces. Oil globules were abundant in the parenchymatous cortex and pith. This observation clearly showed that the essential oil is deposited inside the plant cells.
Figure 7. Microscopic images showing the normal plant tissue and oil globule of the cassumunar ginger rhizomes: (a) light microscope, (b) fluorescent microscope FITC filter and (c) fluorescent microscope Tx Red filter (bar = 250 μm).
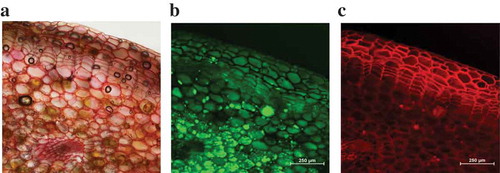
The microstructural views of the transverse section of cassumunar ginger rhizomes before and after extraction by SFME and HD are given in . The unprocessed sample () showed a regular structure of the oil globules in parenchyma with normal cellulosic walls. The cell walls of parenchyma were still intact with some ruptures, atrophic and wrinkles appearing in the sample after HD treatment for 5 h (). The losses of oil cells from the plant matrices were clearly observed with completely crimped and broken plant cell walls after SFME for 70 min ().
Figure 8. Light micrographs of cassumunar ginger rhizome samples: (a) untreated sample, (b) sample treated with HD for 5 h and (c) sample treated with SFME for 70 min (bar = 100 μm).
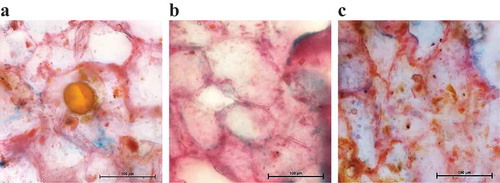
A plausible mechanism of the oil isolation by SFME was illustrated in detail by light microscopy. As shown in , the microstructure of the plant sample before extraction contained oil globules located inside the cells. An obvious effect of the microwave irradiation on the microstructure changes of the samples was found after extraction by SFME with different irradiation times (10, 25, 50, 60 and 70 min) (). In this case, the extraction mechanism could be described in that way that the microwaves interacted selectively with the in-situ water (the free water molecules) located inside the plant cells to give the ‘microwave heating effect’ during electromagnetic radiation. The heated areas inside the plant matrices near or above the boiling point of water resulted in a dramatic expansion of plant cells due to higher internal pressures and subsequent to the cell wall rupture and the bursting of oil globules [3]. Thus, the essential oil migrated quickly from the plant cells flowing toward the surrounding system. Moreover, many volatile substances having dipolar moment properties present in the essential oil can also strongly absorb the microwave energy. These properties resulted in an easier essential oil extraction if the sample contained aromatic substances with high dipolar moments in contrast to those having low dipolar moment values. In term of the eco-friendly procedure, SFME would be a promising process requiring 4.3-fold less time than HD.
3.7. Physical properties of the essential oil
The physical properties of the essential oil isolated by SFME and HD were compiled in . The essential oil obtained from SFME had a pale yellow color comparable to the conventional HD. The oil quality was then subjected to sensory analysis in comparison to the oil resulting from HD. In this case, the aroma of cassumunar ginger oil was a result of a complex mixture of volatile constituents (monoterpenes, sesquiterpenes and oxygenated compounds). A similar smell was found between the essential oils of the two extraction methods showing a strong aroma initially with top to middle notes of cassumunar ginger flavor. Moreover, the scent profile of the tested oils was classified to be cool, earthy, herbaceous and woody-smelling. Several studies suggested that the oxygenated compounds play a key role in the characteristic of the strong odor of the essential oils (Citation23,Citation30). Because the cassumunar ginger essential oil extracted by SFME and HD contained the oxygenated groups of volatile compounds as major components. These predominant compounds including terpinen-4-ol and DMPBD should be important substances in relation to such aromatic profile. Other properties of the oil isolated by SFME including relative density and refractive index were slightly higher than HD (p < 0.05). However, all the tested physical properties corresponded to the specification according to Thai Industrial Standard (Citation31).
Table 4. Physical characteristics of the essential oil isolated from the fresh cassumunar ginger rhizomes by SFME and HD..
4. Conclusions
The essential oil of cassumunar ginger rhizomes could be successfully isolated by application of the SFME combined with CCD. The second-order polynomial equation was an adequate mathematic model for calculating the most effective parameters of the extraction process. The optimal conditions were microwave power of 700 W and irradiation time of 70 min that provided the highest yield of essential oil (1.12 ± 0.10% v/w). Microwave power was the main factor underlying the extraction efficiency of oil yield, followed by microwave exposure time. The major components of the essential oil obtained from SFME and HD were slightly different, although SFME gave a higher concentration of oxygenated compounds. Meanwhile, isolation of the essential oil by SFME also provided better extractability than a conventional HD as confirmed by a more plant cell rupture following the microwave treatment. Therefore, application of the SFME with CCD for isolating the essential oil from cassumunar ginger was clearly advantageous in terms of simplicity, rapidity and environmental friendly technique. A large-scale industrial production required to be further studied for commercialization.
Acknowledgments
Research reported in this study was supported by the ASEAN-European Academic University Network (ASEA-UNINET). Special thanks to Mr. Kurt Plöschberger for his technical support on GC-MS analysis.
Disclosure statement
No potential conflict of interest was reported by the authors.
Additional information
Funding
References
- A. Racoti, A.J. Buttress, E. Binner, C. Dodds, A. Trifan and I. Calinescu, Microwave assisted hydro-distillation of essential oils from fresh ginger root (Zingiber officinale Roscoe). Journal of Essential Oil Research, 29, 471–480 2017.
- Q. Cui, L.T. Wang, J.Z. Liu, H.M. Wang, N. Guo, C.B. Gu and Y.J. Fu, Rapid extraction of Amomum tsao-ko essential oil and determination of its chemical composition, antioxidant and antimicrobial activities. Journal of Chromatography B, 1061, 364–371 2017.
- H.S. Kusuma and M. Mahfud, Comparison of conventional and microwave-assisted distillation of essential oil from Pogostemon cablin leaves: analysis and modelling of heat and mass transfer. Journal of Applied Research on Medicinal and Aromatic Plants, 4, 55–65 2017.
- H.S. Kusuma, A. Altway and M. Mahfud, Solvent-free microwave extraction of essential oil from dried patchouli (Pogostemon cablin Benth) leaves. Journal of Industrial and Engineering Chemistry, 58, 343–348 2018. 09.047.
- A. Farhat, H. Benmoussa, R. Bachoual, Z. Nasfi, W. Elfalleh, M. Romdhane and J. Bouajila, Efficiency of the optimized microwave assisted extractions on the yield, chemical composition and biological activities of Tunisian Rosmarinus officinalis L. Essential Oil. Food and Bioproducts Processing, 105, 224–233 2017.
- H. Mellouk, A. Meullemiestre, Z. Maache-Rezzoug, B. Bejjani, A. Dani and S.A. Rezzoug, Valorization of industrial wastes from French maritime pine bark by solvent free microwave extraction of volatiles. Journal of Cleaner Production, 112, 4398–4405 2016.
- A. Filly, A.S. Fabiano-Tixier, C. Louis, X. Fernandez and F. Chemat, Water as a green solvent combined with different techniques for extraction of essential oil from lavender flowers. Comptes Rendus Chimie, 196, 707–717 2016.
- M.R. Thakker, J.K. Parikh and M.A. Desai, Microwave assisted extraction of essential oil from the leaves of Palmarosa: Multi-response optimization and predictive modelling. Industrial Crops and Products, 86, 311–319 2016.
- B. Chongmelaxme, R. Sruamsiri, P. Dilokthornsakul, T. Dhippayom, C. Kongkaew, S. Saokaew, A. Chuthaputti and N. Chaiyakunapruk, Clinical effects of Zingiber cassumunar (Plai): A systematic review. Complementary Therapies in Medicine, 35, 70–77 2017.
- K. Jamir and K. Seshagirirao, Purification, biochemical characterization and antioxidant property of ZCPG, a cysteine protease from Zingiber montanum rhizome. International Journal of Biological Macromolecules, 106, 719–729 2018.
- R. Sanatombi and K. Sanatombi, Biotechnology of Zingiber montanum (Koeniq) Link ex A. Dietr.: A review. Applied Research on Medicinal and Aromatic Plants, 4, 1–4, 2017.
- S. Bua-In and Y. Paisooksantivatana, Essential oil and antioxidant activity of cassumunar ginger (Zingiberaceae: zingiber montanum (Koenig) Link ex Dietr.) collected from various parts of Thailand. Kasetsart Journal (Natural Science), 43, 467–475 2009.
- B. Manochai, P. Paisooksantivatana, H. Choi and J.H. Hong, Variation in DPPH scavenging activity and major volatile oil components of cassumunar ginger, Zingiber montanum (Koenig), in response to water deficit and light intensity. Scientia Horticulturae, 1264, 462–466 2010.
- U.R.P. Sukatta, P. Punjee, S. Chidchendey and V. Keeratinijakal, Chemical composition and physical properties of oil from cassumunar ginger (Zingiber cassumunar Roxb.) obtained by hydro distillation and hexane extraction. Kasetsart Journal (Natural Science), 43, 212–217 2009.
- R. Jeenapongsa, K. Yoovathaworn, K.M. Sriwatanakul, U. Pongprayoon and K. Sriwatanakul, Anti-inflammatory activity of (E)-1-(3,4-dimethoxyphenyl) butadiene from Zingiber cassumunar Roxb. Journal of Ethnopharmacology, 872, 143–148 2003.
- A. Panthong, D. Kajanapothi, W. Niwatananant, P. Tuntiwachwuttikul and V. Reutrakul, Anti-inflammatory activity of compound D {(E)-4-(3′,4′-dimethoxyphenyl)but-3-en-2-ol} isolated from Zingiber cassumunar Roxb. Phytomedicine: International Journal of Phytotherapy and Phytopharmacology, 34, 207–212 1997.
- U. Pongprayoon, P. Tuchinda, P. Claeson, T. Sematong, V. Reutrakul and P. Soontornsaratune, Topical antiinflammatory activity of the major lipophilic constituents of the rhizome of Zingiber cassumunar. Part II: Hexane Extractives. Phytomedicine, 34, 323–326 1997.
- Y. Lu, C. Sun, Y. Wang and P. Pan, Preparative isolation and purification of two phenylbutenoids from the rhizomes of Zingiber cassumunar by upright counter-current chromatography. Journal of Chromatography A, 1089, 258–262 2005.
- Y. Ozaki, N. Kawahara and M. Harada, Anti-inflammatory effect of Zinger cassumunar Roxb. and its active principles. Chemical and Pharmaceutical Bulletin, 399, 2353–2356 1991.
- National Drug Committee, The National List of Essential Drugs, Department for Development of Thai Traditional and Alternative Medicine Ministry of Public Health Bangkok Thailand, 2015.
- A. Priprem, K. Janpim, S. Nualkaew and P. Mhakunakorn, Topical niosome gel of Zingiber cassumunar Roxb. extract for anti-inflammatory activity enhanced skin permeation and stability of compound D. AAPS PharmSciTech, 173, 631–639 2016.
- G. Marquina-Chidsey, B. Monro, I. Velázquez, D. Arias, E. Pérez, D.T. Santos and M. Vargas, Evaluation of supercritical fluid extraction of tacamahaco (Protium heptaphyllum) resin. Journal of Essential Oil Research, 296, 443–450 2017.
- B. Yingngam and A.H. Brantner, Factorial design of essential oil extraction from Fagraea fragrans Roxb. flowers and evaluation of its biological activities for perfumery and cosmetic applications. International Journal of Cosmetic Science, 373, 272–281 2015.
- B. Yingngam, K. Tantiraksaroj, T. Taweetao, W. Rungseevijitprapa, N. Supaka and A.H. Brantner, Modeling and stability study of the anthocyanin-rich maoberry fruit extract in the fast dissolving spray dried microparticles. Powder Technology, 325, 261–270 2018.
- Thai Herbal Pharmacopoeia Vol. 1, Department of Medical Sciences, Ministry of Public Health, Prachachon Co., Ltd. Bangkok Thailand, 1998.
- N. Sahraoui, M. Hazzit and C. Boutekedjiret, Effects of microwave heating on the antioxidant and insecticidal activities of essential oil of Origanum glandulosum Desf. obtained by microwave steam distillation. Journal of Essential Oil Research, 295, 1–10 2017.
- X. Li, W. Wang, M. Luo, C. Li, Y. Zu, P. Mu and Y. Fu, Solvent-free microwave extraction of essential oil from Dryopteris fragrans and evaluation of antioxidant activity. Food Chemistry, 1332, 437–444 2012.
- L. Muruganandam, A. Krishna, J. Reddy and G.S. Nirmala, Optimization studies on extraction of phytocomponents from betel leaves. Resource-Efficient Technologies, 34, 385–393 2017.
- J. Jiao, G. Qing-Yan, F. Yu-Iie, Z. Yuang-Gang, L. Meng, Z. Chun-Jian and L. Chun-Ying, Microwave-assisted ionic liquids treatment followed by hydro-distillation for the efficient isolation of essential oil from fructus forsythiae seed. Separation and Purification Technology, 107, 228–237 2013.
- C. Villa, B. Trucchi, A. Bertoli, L. Pistelli, A. Parodi, A.M. Bassi and B. Ruffoni, Salvia somalensis essential oil as a potential cosmetic ingredient: Solvent-free microwave extraction, hydrodistillation, GC-MS analysis, odour evaluation and in vitro cytotoxicity assays. International Journal of Cosmetic Science, 31, 55–61 2009.
- Thai Industrial Standard Institute, TISI1679–2541: Phlai Oil, Thai Industrial Standards Institute Publishing Corp, Bangkok, Thailand 2000.
- J. Wungsintaweekul, W. Sitthithaworn, W. Putalun, H.W. Pfeifhoffer and A. Brantner, Antimicrobial, antioxidant activities and chemical composition of selected Thai spices. Songklanakarin Journal of Science and Technology, 326, 589–598 2010.
- R.P. Adams, Identification of essential oil components by gas chromatography/mass spectrometry, 4th edn, Allured Publishing Corp, Carol Stream, IL, U.S.A 2007.
- A.K. Bordoloi, J. Sperkova and P.A. Leclercq, Essential oils of Zingiber cassumunar Roxb. from northeast India. Journal of Essential Oil Research, 114, 441–445 1999.