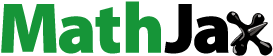
Abstract
Polymethylmethacrylate (PMMA) has been irradiated by 365 nm UV wavelength at different exposition times in air and room temperature. The macromolecular modifications induced in the polymer have been investigated using attenuated total reflectance (ATR) coupled to Fourier transform infrared (FTIR) spectroscopy, UV-Visible spectroscopy, surface profiler, wetting ability, and electronic microscopy. The observed chemical, optical and morphological changes occurred as a consequence of the UV irradiation suggest that chain scissions are induced by the UV radiations, with reduction of the IR and visible transmittance, wetting ability and surface roughness. The study of the degradation of PMMA by different times of exposure to UV radiation is investigated with the aim of understanding the effects produced over time on optical devices such as intraocular lenses, optical fibres, protective screens, and instrumental lenses.
1. Introduction
In 1949 Harold Ridley implanted in Britain the first intraocular lens (IOL) changing the cataract surgery and offering an optical correction of postsurgical aphakia (Citation1). Since this pioneering event the ophthalmic surgery improved dramatically due to the impressive technological progress related both to surgical instruments and techniques and IOL materials. Cataract surgery is the most performed procedure ever with several millions of procedures a year in the world and actual approach comprise a phacoemulsification technique with IOL implantation. For many years the IOLs were composed of polymethylmethacrylate (PMMA) an optically clear and rigid acrylic polymer made of the methyl ester of methacrylic acid. Although currently this material is present only in some types of IOLs, there are still a great number of patients who underwent cataract surgery with PMMA IOL implantation. PMMA is produced by the methyl methacrylic acid monomer (C5O2H8)n which is toxic, but the polymerized molecule of final PMMA is inert and its virtual non-reactivity suggest its use for long-term implantation (Citation2,Citation3). PMMA is a light, transparent and rigid thermoplastic polymer useful for many applications in Physics, Medicine, Biology, Microelectronics, Engineering and other fields (Citation4–6). The high transparency of PMMA to the visible region (∼ 92%) makes its use possible for many optical applications, ranging from extra and intra ocular lenses to protective windows for different environments, from homes to cars, to vacuum windows, eyeglasses, optical fibres, to protection of solar cells and different type of lamps (Citation7,Citation8). Many scintillator detectors, plastic containers, implantable biomaterials and insulating substrates for microelectronics are based on PMMA (Citation9,Citation10).
The continuum use of PMMA leads to aging of the material over time, not only due to thermal effects but above all due to its exposure to UV radiation coming from sunlight, ambient lighting lamps, tanning lamps, and other UV sources such as arc welding processes, exposure to X-ray, laser and ionizing radiations (Citation11–13). Lasers, for example, may induce thermal degradation by IR exposition and photochemical degradation by UV irradiation effects (Citation14).
The PMMA degradation is due to a change of its initial physical and chemical properties, in fact the UV irradiation may break chemical bonds generating radicals and producing scission effects, according to the literature (Citation15).
Degradation often leads to a reduction in optical transmittance, increased in absorbance and light scattering, yellowing of the polymer, mechanical embrittlement of the material and generation of microcracks (Citation15).
The purpose of the present work is to study the effect of UV exposition in air as a function of the time, up to 23 hours. Results are presented and discussed applying the investigation to PMMA sheets and to some extra- and intra-ocular lens routinely used in ophthalmology.
2. Materials and methods
PMMA has specific chemical and physical properties, such as excellent chemical and physical stability, is insoluble in water, resistant to salty water, has excellent resistance to wear, has high hardness and high resistance to chemical agents, it is biocompatible and represents a water equivalent material for ionizing radiations (Citation16).
Same properties of the polymer are reported in Table .
Table 1. Some physical properties of the PMMA.
The polymethylmethacrylate (PMMA) used in this paper is commercially available from Goodfellow (Citation17).
The exposure to ultraviolet radiation was performed according to ASTM G154 standard (Citation18) using the UV lamp Blak-Ray, model UVL-56, with a wavelength centered at 365 nm, corresponding to a photon energy of 3.38 eV (Citation19).
The fluence of the source applied to the PMMA samples was about 200 J/cm2.
The irradiations were performed in air, at room temperature (20 °C), 1 atm pressure, and 45% humidity. Samples were exposed at about 1.5 cm distance from the lamp in variation of the UV exposure times from 1 hr up to 23 hr only on a face.
The 1 mm thick PMMA sample used for this investigation is reported in Figure a. Figure b reports the emission spectrum of emitted photons vs wavelength, indicating that the irradiation uses photons with wavelength ranging between about 355 and 385 nm, centred at 365 nm.
The lamp emission spectrum was detected with an UV Vis spectrophotometer (AvaSpec-ULS2048CL-EVO) operating with high sensitivity in the wavelength range 250–800 nm.
The CXRO code (Citation20) permits to determine the penetration depth of these radiations in PMMA, which, fixing the transmission reduction to 10% of its maximum intensity, is of about 100 microns. Thus, the polymer damage concerns mainly the most superficial layers exposed to UV.
The effects of the ultraviolet radiation on the PMMA samples were investigated by several characterization techniques: the surface profilometer (Tencor P-10) to measure the roughness with a depth resolution of 1 nm; the sessile water drop to measure the contact angle; the attenuated total reflectance (ATR) coupled to Fourier transform infrared (FTIR) (Jasco 4600) spectroscopy, to study the chemical changes in sample before and after UV irradiating at different exposure time. The FTIR was recorded in the range from 4000 to 450 cm−1, while UV-VIS optical spectroscopy (Jasco 470) investigated the changes in the visible transmittance in the wavelength region 200–850 nm. Further morphological analysis involved the use of Scanning Electron Microscopy (SEM), which was performed using the Zeiss Cross Beam 540 microscope.
3. Results
The PMMA polymeric monomer is representable by the chemical structure reported in Figure . It evinces that different groups of C, H and O are present in the polymer and that they may vibrate and absorb at different IR wavelengths.
Figure reports a typical ATR-FTIR spectrum of pure and virgin PMMA. The ATR-FTIR transmittance spectrum of pure PMMA reported in Figure is confirmed by a vast literature (Citation21).
This spectrum shows the presence of CH2 rocking mode peaks at 746, 846, and 991 cm−1. An asymmetric stretching peak appears at 1143 cm−1, which can be attributed to the C-O-C group. The C-C-O stretching vibrations in the methyl carbonyl group is observed at about 1280 cm−1, while the peak at 1440 cm−1 is due to the C-H deformation, according to the literature (Citation2).
A high peak, indicating an excessive absorbance, is observed at 1722cm−1, which is due to the stretching vibration of the carbonyl group C = O. Moreover, at high wavenumber, appears a transmittance peak at about 2949 cm−1 resulting from the C-H bonds absorbance.
To convert a value from percent transmittance (%T) to absorbance, we used the following equation:
(1)
(1) The absorbance spectrum for the virgin PMMA is reported in Figure .
The absorbance spectrum evinces typical groups of PMMA, such as the strong peak located at 1722 cm–1 attributed to the C = O carbonyl group, the C = C stretching, CH3 symmetrical deformation, and CH2 twisting modes observed at 1660, 1376 and 1242 cm–1, respectively.
A strong peak due to O–CH3 is located at 1446 cm–1. The doublet peak of C–O stretching mode of PMMA can be observed at 1100–1210 cm–1, with a strong peak at 1147 cm–1, according to the literature (Citation22).
From this spectrum it is possible to evaluate the oxidation index of the analysed samples. Generally, the peak ratio between the carbonyl group C = O at 1722cm−1 and the methylene group CH2 at 746 cm−1 can be taken into consideration to evaluate the oxidation index in the polymer as:
(2)
(2) The oxidation index in the pristine PMMA is about 6.69.
The ATR-FTIR spectroscopy shows significant differences between the pristine and the irradiated PMMA which enhance with the UV exposition time.
Figure reports a comparison between different FTIR transmittance spectra obtained in PMMA pristine and samples exposed for different times, from 1 hr up to 23 hrs to the 365 nm UV radiation. It is possible to observe that the 1 mm PMMA sheet transmittance for wavenumber ranging between 1000 and 3500 cm−1 decreases with the UV exposition time. Moreover, for times up to about 3 hr a structured double peak is generated at about 2333 cm−1 showing a clear increment with the exposition time. According to the literature (Citation23), this peak corresponds to the stretching mode of the O = C = O carbon dioxide. It indicates that the UV radiation break PMMA chemical bonds generating reactive radicals with formation of CO2 bonded to the polymeric matrix. This peak does not appear in samples irradiated for long times of the order of 10–23 hr.
The spectra comparison above reported indicate that the mean peaks decrease in intensity for long UV exposition time, such occur clearly for the peaks due to CH2 at 2949 cm−1, C = O at 1722 cm−1, O-CH3 at 1446 cm−1, C-O-C at 1143 cm−1 and at 987 cm−1, and CH2 at 746 cm−1. It means that the high UV absorbed dose produces scission effects on these functional groups creating unstable radicals which decrease their content in the first irradiated superficial layers of the polymer, according to the literature (Citation15,Citation24). This type of photon damage generates a significant decreasing of the IR transmittivity in the entire PMMA sheet.
The oxidation index in the irradiated PMMA decreases with the exposition time to UV. For example, using Equation (2) applied to the longer exposition time (23 hr) it results of about 2.6, indicating a strong reduction of the oxygen species in the surface irradiated layers.
Figure reports the results obtained using the UV-VIS transmittance spectroscopy in the visible and near UV and IR wavelength range for pristine and very exposed polymer. The pristine PMMA spectrum is confirmed by the literature (Citation25).
Figure 6. UV-VIS spectroscopy comparison between the pristine (green line) and the higher time UV exposed (red line) PMMA samples.
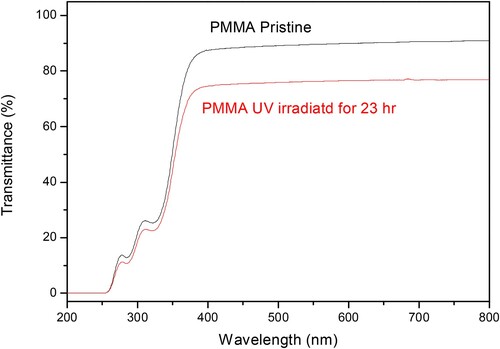
Such spectra indicate that the 1 mm thick PMMA pristine has a UV transmittance very low, less than 15%, for UV radiations with wavelength minor than 300 nm, and that it rises rapidly to about 90% for wavelengths between about 300 and 400 nm up to reach a value of about 92% at about 800 nm.
The reported spectra comparison shows that the transmittance in the pristine PMMA is significantly reduced for UV exposition at the maximum time of 23 hr. A global transmittance reduction from about 90% to about to about 75% is obtained at a wavelength around to 500 nm.
The roughness of the PMMA sheet surface was investigated using the surface profiler providing a scan length analysis on 500 microns with a depth sensitivity of 1 nm. Figure reports a typical roughness measure for the pristine (a) and the 23 hr higher time exposed (b) PMMA sample surface. The red curves represent the polynomial fit of the surface. The roughness measure concerns Rq, the quadratic mean, or root mean square average of profile height deviations from the mean line. The comparison of Figure shows a clear reduction of about 50% of the average Rq roughness by the UV irradiation at 23 hr in air. In facts, it changes his average value from the initial value of about 50 nm to about 25 nm. Thus, although the irradiation involves only the molecular species of the PMMA surface layer, it makes the surface of the polymer less wrinkled, as if it had been affected by mechanical wear, significantly modifying the morphology of the first layers.
Figure 7. Roughness of the pristine PMMA (a) and of the 23 hr UV irradiated PMMA (b) with the polynomial fits of the surface (red lines).
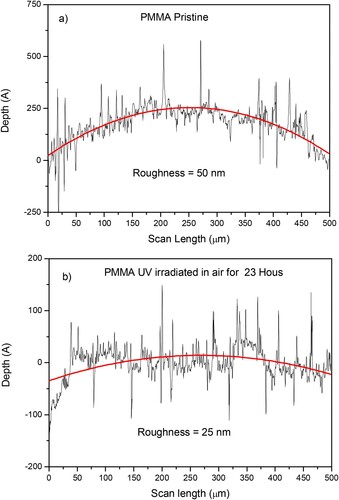
This last result pointed us to further study of the possible modifications of the surface of the irradiated polymer. In this regard, measurements of the wettability of water were carried out as the same times of UV exposure previously reported. Figure shows the results on the measure of the contact angle for the PMMA surface in pristine (a), and after UV irradiation for 1 hr (b), 3 hr (c) and 23 hr (d). The contact angle of 68° measured in the pristine sample increases up to about 77° after an irradiation of 23 hr in air. Thus the 365 nm UV irradiation decreases the hydrophilic properties of the pristine PMMA surface making the surface less wettable.
Finally, the investigation was concluded with a SEM observation of the PMMA surface to observe the morphology differences between the pristine surface and that irradiated for long times (23 hr), as reported in Figure a and b, respectively. Also, such results confirm that the surface appears to be slightly flattened by the long UV irradiation times and that it appears more hydrophobic than the virgin one.
4. Conclusions
The study performed on the PMMA under UV irradiation at 365 nm wavelength in air, as a function of the irradiation time, has demonstrated that the surface polymer layers, up to 1 micron depth, reduces the polymer transparency to UV, visible and near IR radiations. The transmittivity of the PMMA foil, in facts, is significantly reduced after 23 hr UV irradiation time in air, at which oxidation, and different functional groups of oxygen are produced in the polymer surface. However, such PMMA modifications are detected mainly in the first superficial layers where UV strongly interact with the polymer molecules and not involve the entire polymer thickness. In fact, the 365 nm UV penetration depth in PMMA is of about 100 microns.
The polymer molecules are damaged by the 3.38 eV photon flux irradiation as demonstrated by the FTIR spectra modifications.
Such photons, in facts, induce photochemical effects producing molecular bond breaking, scissions, formation of radicals and rearrangement of the surface molecular bonding. These effects decrease the transparency to the visible and IR wavelengths resulting in forced aging of the polymer which increases its opacity and the visible light absorption and reduces the functional groups present in his surface, the roughness and the wettability.
The PMMA degradation increased with the irradiation time following to high numbers of chain cleavage.
The results obtained analysing the 1 mm thick pure PMMA sheet can be interpreted as the effects produced by UV radiation on PMMA-based intraocular lenses and other optical devices which show aging over time that can be accelerated by possible exposure to short wavelength radiation. The exposure to hot welding processes, diagnostic X-rays, tanning lamps and ionizing radiation sources can alter the optical and surface properties of these materials with premature aging which the literature reports not only as optical but also chemical properties, electrical and mechanical. These results are preliminary to the more intense investigation, which is in progress, and which will be performed directly on IOL devices under different UV irradiations to demonstrate their degradation with the time, as will be reported in the next paper.
References
- Apple, D.J.; Sims, J. Harold Ridley and the Invention of the Intraocular Lens. Surv. Ophthalmol. 1996, 40 (4), 279–292.
- Ali, U.; Bt, K.J.; Karim, A.; Buang, N.A. A Review of the Properties and Applications of Poly (Methyl Methacrylate) (PMMA). Polym. Rev. 2015, 55 (4), 678–705.
- Mainster, M.A. Spectral Transmittance of Intraocular Lenses and Retinal Damage from Intense Light Sources. Am. J. Ophthalmol. 1978, 85 (2), 167–170.
- Salimgareeva, V.N.; Kolesov, S.V. Plastic Scintillators Based on Polymethyl Methacrylate: A Reveiw. Instrum. Exp. Techn. 2005, 48, 273–282.
- Werner, L. Intraocular Lenses: Overview of Designs, Materials, and Pathophysiologic Features. Ophthalmology 2021, 128 (11), e74–e93.
- Pituru, S.M.; Greabu, M.; Totan, A.; Imre, M.; Pantea, M.; Spinu, T.; Tancu, A.M.C.; Popoviciu, N.O.; Stanescu, I.I.; Ionescu, E. A Review on the Biocompatibility of PMMA-Based Dental Materials for Interim Prosthetic Restorations with a Glimpse Into Their Modern Manufacturing Techniques. Materials 2020, 13 (13), 2894.
- Gozum, N.; Safgonul Unal, E.; Altan-Yaycioglu, R.; Gucukoglu, A.; Ozgun, C. Visual Performance of Acrylic and PMMA Intraocular Lenses. Eye 2003, 17, 238–242.
- Ding, D.; Lanzetta, L.; Liang, X.; Min, G.; Giza, M.; Macdonald, T.J.; Haque, S.A. Ultrathin Polymethylmethacrylate Interlayers Boost Performance of Hybrid tin Halide Perovskite Solar Cells. Chem. Commun. 2021, 57, 5047–5050.
- Charasseangpaisarn, T.; Wiwatwarrapan, C.; Thunyakitpisal, P.; Srimaneepong, V. Development of Poly(Methyl Methacrylate)/Poly(Lactic Acid) Blend as Sustainable Biomaterial for Dental Applications. Sci. Rep. 2023, 13, 16904.
- Kim, S.; Seoung, Y.H.; Kim, J.H.; Kim, K.; Kim, G.; Kang, S.C.; Kim, H.; Kim, B.; Kothan, S.; Kaewjaeng, S.; Nam, U.W. Characteristics of Photopolymerized Tissue Equivalent Plastic Scintillator in High Dose Rate Radiotherapy. Radiat. Phys. Chem. 2023, 203 (Part A), 110600.
- Alobaidani, A.D.; Furniss, D.; Johnson, M.S.; Endruweit, A.; Seddon, A.B. Optical Transmission of PMMA Optical Fibres Exposed to High Intensity UVA and Visible Blue Light. Opt. Lasers Eng. 2010, 48 (5), 575–582.
- Fragalà, M.E.; Compagnini, G.; Torrisi, L.; Puglisi, O. Ion Beam Assisted Unzipping of PMMA. Nucl. Instrum. Methods Phys. Res. B 1998, 141, 169–173.
- Torrisi, L. Ion Irradiation of Polymethylmetacrylate (PMMA). Radiat. Effects Defects Solids 1998, 145, 285–296.
- Torrisi, L.; Lorusso, A.; Nassisi, V.; Picciotto, A. Characterization of Laser Ablation of Polymethylmethacrylate at Different Laser Wavelengths. Radiat. Effects Defects Solids 2008, 163 (3), 179–187.
- de Castro Monsores, K.G.; da Silva, A.O.; de Sant’ Ana Oliveira, S.; Passos Rodrigues, J.G.; Pondé Weber, R. Influence of Ultraviolet Radiation on Polymethylmethacrylate (PMMA). J. Mater. Res. Technol. 2019, 8 (5), 3713–3718.
- Physical Properties Table – Thermo Fisher Scientific. Actual web site 2023: D20826∼.pdf (thermoscientific.com).
- Goodfellow Products. Actual web site 2023: https://www.goodfellow.com/search?searchTerms=PMMA&sortCode=RELEVANCE.
- ASTM. Standard practice for operating fluorescent ultraviolet (UV) lamp apparatus for exposure of nonmetallic materials, ASTM G154-16, ASTM International, 2016, actual web site 2023: https://img.antpedia.com/standard/files/pdfs_ora/20200926/ASTM%20G154-16.pdf.
- Blak-Ray UV Lamp mod. UVL 56, actual web site 2023: https://www.fishersci.co.uk/shop/products/uvl-56-handheld-uv-lamp/11738221.
- CXRO. Actual web site 2023: https://henke.lbl.gov/optical_constants/.
- Raheem, D.; Majid, Z. Polymethyl Methacrylate-Collagen-Magnesium Hydroxyapatite Bone Cement Preparation for Orthopedic Application. Chin. J. Biomed Eng. 2020, 29 (3), 18–23.
- Salim, Y.S.; Zainudin, N.A.; Chan, C.H.; Chan, K. Correlation Between the Storage Time of the NRL and the Efficiency of PMMA Grafting to NR, Chapter 12, from: Key Elements in Polymers for Engineers and Chemists; Apple Academic Press, Inc.: Oakville, Canada, 2014.
- Schott, J.A.; Do-Thanh, C.L.; Shan, W.; Puskar, N.G.; Dai, S.; Mahurin, S.M. FTIR Investigation of the Interfacial Properties and Mechanisms of CO2 Sorption in Porous Ionic Liquids. Green Chem. Eng. 2021, 2 (4), 392–401.
- Evea, S.; Mohrb, J. Study of the Surface Modification of the PMMA by UV-Radiation. Proc. Eng. 2009, 1, 237–240.
- Reyes-Acosta, M.A.; Torres-Huerta, A.M.; Domínguez-Crespo, M.A.; Flores-Vela, A.I.; Dorantes-Rosales, H.J.; Andraca-Adame, J.A. Thermal, Mechanical and UV-Shielding Properties of Poly(Methyl Methacrylate)/Cerium Dioxide Hybrid Systems Obtained by Melt Compounding. Polymers 2015, 7 (9), 1638–1659.