All trans retinoic acid (ATRA) has demonstrated curative activity in acute promyelocytic leukemia (APL), a distinct subtype of acute myeloid leukemia (AML), that is characterized by a chromosomal translocation t(15;17) involving the retinoic acid receptor alpha (RARα) and promyelocytic leukemia (PML) genes [Citation1]. When ATRA is used in combination with arsenic trioxide, most APL patients achieve complete remission [Citation2]. However, success with this relatively nontoxic strategy has not fully translated to other AML subtypes. Early clinical trials of ATRA with anthracycline-based chemotherapy or arsenic trioxide provided conflicting results with potential benefits being restricted to distinct AML subtypes [Citation3,Citation4]. ATRA in combination with arsenic trioxide has been shown to induce apoptosis in NPM1-mutated AML cell [Citation5]. A 21 gene-expression model (ATRA-21) has been developed to predict AML sub-type specific sensitivity to ATRA [Citation6]. Recently it was shown that addition of ATRA to decitabine resulted in higher remission rates in elderly AML patients [Citation7]. These findings suggest that ATRA-based combination therapies have the potential of being clinically effective in non-APL AML and merit investigation of novel, less toxic combination strategies with ATRA, especially for treating older patients who may be ineligible for toxic induction chemotherapy. Previously we reported that the anti-proliferative effects of ATRA in AML and APL cell lines were potentiated when topoisomerase (topo) IIβ was depleted or when topo IIα/topo IIβ were catalytically inhibited by the bis (2,6 dioxypiperazine) derivative, ICRF-193 [Citation8]. Since the clinically approved topo II catalytic inhibitor, dexrazoxane (Dex), is frequently used as an effective cardioprotectant in pediatric AML patients treated with anthracycline-based therapy and shown to lower treatment related mortality [Citation9,Citation10], in this study we examined the effectiveness and mechanisms of the combination of ATRA with Dex, in AML patient blast cells and established AML cell lines.
Since ICRF-193 is more potent than Dex we first evaluated the effect of the combination of ICRF-193 plus ATRA on apoptosis of blast cells from 19 AML patients, with subtypes that were distinct from APL. Treatment of blast cells obtained from AML patients who provided written informed consent in accordance with the Cleveland Clinic institutional review board revealed that the combination of Dex plus ATRA led to enhanced apoptosis as compared to that induced by ICRF-193 or ATRA alone (). Since ICRF-193 inhibits the activity of topo IIα and topo IIβ we determined the expression of these two isoforms in patient AML blast cells. Expression of topo IIβ was 25 ± 8-fold greater than topo IIα (Supplementary Figure 1(A)), suggesting that topo IIβ is likely a major target of ICRF-193 in AML cells. In subsequent studies we evaluated the effects of Dex, the clinically approved topo II inhibitor, in combination with ATRA in (a) blast cells from an AML patient (AML 10) with adequate sample, (b) KG1 cell line, a prototypic non-APL AML cell line that is resistant to ATRA-induced differentiation and (c) HL-60 cell line that is sensitive to ATRA-induced differentiation, despite the absence of the t(15;17) translocation. In all three AML cells, apoptosis was significantly higher in the combination treatment ( and Supplementary Figure 2(A) and 2(B)), with a statistically significant interaction between Dex and ATRA indicating that these two drugs synergize to induce cell death. Although apoptosis induced by Dex plus ATRA was significantly higher than that induced by Dex alone, both Dex and Dex plus ATRA led to comparable decreases in cell growth in KG1 cells (Supplementary Figure 2(C) and 2(D)). This finding suggests that the combination of Dex plus ATRA would be clinically more effective than Dex alone, since the combination, in addition to arresting growth of tumor cells, would lead to tumor cell death. Enhanced apoptosis observed in the presence of Dex plus ATRA was associated with significantly increased caspase 8, 9 and 3/7 activities with the combination treatment in KG1 cells (), suggesting activation of both the intrinsic and extrinsic apoptotic pathways. The combination of Dex plus ATRA also led to significantly increased reactive oxygen species (ROS) accumulation compared to treatment with Dex or ATRA alone in all three AML cells ()
Figure 1. Topo II catalytic inhibitors augment induction of apoptosis, ROS and caspase activities in patient AML blast cells and AML cell lines by ATRA. (A) Blast cells from AML patients treated with 0.25 µM ICRF-193, 1.0 µM ATRA or the combination for 120 h were stained with Hoechst/PI to determine percentage of apoptotic nuclei. (B) Patient AML blast cells (AML 10) and the AML cell lines KG1 and HL-60 treated with 10 μM (AML 10 cells) or 20 μM Dex (KG1 and HL-60 cells), 1.0 μM ATRA or the combination for 72 h were analyzed for apoptosis. (C) KG1 cells treated with 10 μM Dex, 1.0 μM ATRA or the combination for 96 h were analyzed for caspase, 8, 9 and 3/7 activities. (D) Patient AML blast cells (AML 10) and the AML cell lines KG1 and HL-60 treated with 10 μM Dex, 1.0 μM ATRA or the combination for 72 h (AML 10 and KG1 cells) or 96 h (HL-60) were analyzed for ROS. Statistical significance was determined by Two-way ANOVA with SNK posthoc test when appropriate. *p ≤ .05, **p ≤ .01, ***p ≤ .001. A statistically significant interaction, suggestive of synergism, between ± ATRA and ± Dex was observed for apoptosis in all AML cells (p < .001 for AML 10, p = .002 for KG1 and p < .001 for HL-60). However, a statistically significant interaction was not observed for caspase activities and ROS, although caspase activities and ROS were significantly higher in the combination treatment than that observed in treatment with drugs alone.
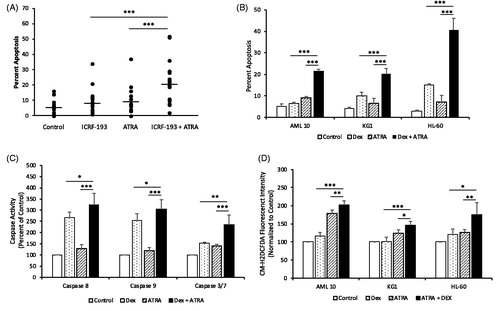
Previously we had shown that downregulation of the antioxidant protein peroxiredoxin (PRDX) 2 may be involved in increased ATRA-induced apoptosis and ROS in topo IIβ-depleted cells, since exogenous expression of PRDX2 partially reversed apoptosis and ROS accumulation [Citation8]. Surprisingly, treatment with Dex did not result in reduced expression of PRDX2, despite decreases in topo IIβ levels (data not shown). However, expression of the homologous antioxidant protein, PRDX1, a key player in ROS catabolism, was decreased in the presence of ATRA or Dex plus ATRA, albeit to the same extent (), This finding suggests that while downregulation of PRDX1 by ATRA likely regulates intracellular ROS levels, enhanced ROS accumulation observed in the presence of Dex plus ATRA is not due to a further reduction in PRDX1 expression.
Figure 2. Downregulation of the antioxidant protein PRDX1 by ATRA is not enhanced by Dex, whereas expression of the deoxynucleotide-triphosphate (dNTP) hydrolase, SAMHD1 is increased in the combination treatment of Dex and ATRA. (A) Cell extracts of primary AML cells treated with 0.25 µM ICRF 193, 1.0 µM ATRA or the combination daily for 96 h (AML 10 and HL-60 cells) or with 20 μM Dex, 1.0 µM ATRA or the combination for 72 h (KG1 cells) were prepared in RIPA buffer. An aliquot (10-20 μg) was subjected to Western blot analysis for PRDX1. (B) Differentially expressed genes between Dex plus ATRA vs. ATRA treatment in KG1 and AML 10 cells determined by microarray analysis. Graph of log2fold change in KG1 and AML 10 treated cells for a key subset of genes. (C) SAMHD1 mRNA expression analysis by qPCR in AML 10 cells treated in the absence or presence of 10 μM Dex, 1.0 μM ATRA or the combination of 10 μM Dex and 1.0 μM ATRA for 72 h (D) SAMHD1 mRNA expression analysis by qPCR in KG1 and HL-60 cells treated in the absence or presence of 20 μM Dex, 1.0 μM ATRA or the combination of 20 μM Dex and 1.0 μM ATRA for 72 h. (E) Western blot analysis for expression of SAMHD1 protein in AML 10, KG1 and HL-60 cells. (F) Western blot analysis of SAMHD1 protein in AML 10, KG1 and HL-60 cells treated in the absence or presence of 10 μM (AML 10 cells) or 20 μM (KG1 and HL-60 cells) Dex, 1.0 μM ATRA or the combination for 72 h. Statistical significance was determined by Two-way ANOVA with SNK posthoc test when appropriate. *p ≤ .05, ** p ≤ .01.
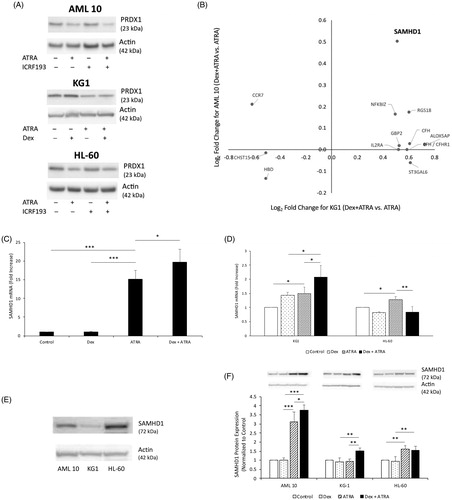
Since activation of the MAPK/ERK pathway by ATRA promotes myeloid differentiation [Citation11], we determined whether ERK phosphorylation may also be involved in apoptosis induced by ATRA or the combination of Dex plus ATRA. Phosphorylation of ERK was not observed following treatment with ATRA, ICRF-193/Dex or the combination in any patient AML samples tested or KG1 cells (Supplementary Figure 3(A) and 3(B)), which do not undergo differentiation by ATRA. However, in HL-60 cells which differentiate in the presence of ATRA, ERK phosphorylation was increased following ATRA treatment (Supplementary Figure 3(B)), which was attenuated in combination treatment with Dex. The MAPK/ERK kinase inhibitor, PD98059, inhibited ATRA-induced differentiation (Supplementary Figure 3(C)) but had no effect on apoptosis induced by ATRA or the combination of Dex plus ATRA in HL-60 cells (Supplementary Figure 3(D)). These results suggest that while ERK phosphorylation is involved in ATRA-induced differentiation, apoptosis occurs independently of differentiation and is not mediated by Dex, ATRA or the combination.
To further characterize the mechanisms for the potentiating effects of Dex on ATRA-induced apoptosis and ROS accumulation, we performed microarray analysis of mRNA isolated from KG1 and AML 10 cells treated with Dex, ATRA or the combination. Our results revealed that while several genes were differentially expressed in ATRA treated AML 10 and KG1 cells (FDR < 0.05), as compared to untreated cells, treatment with Dex alone resulted in differential expression of very few genes (FDR < 0.05). Since Dex potentiates ATRA-induced apoptosis and intracellular ROS accumulation, we compared differentially expressed genes between Dex plus ATRA treatment vs. ATRA treatment. In KG1 cells 12 differentially expressed genes (DEG) were identified with an FDR < 0.05 ( and Supplementary Table 1), but no significant interaction between Dex and ATRA treatments was observed. However, in AML 10 cells no DEG were detected with an FDR < 0.05. This was attributed to a higher variability observed with AML 10 cells which reduced statistical power. Therefore, we used the 12 genes from the KG1 analysis as a gene filter for common differential expression with AML 10 and found that the expression of only sterile α motif and HD domain-containing protein 1 (SAMHD1) was also upregulated about 1.5-fold with an unadjusted p-value < .05 ( and Supplementary Table 1). This result was confirmed by qPCR ().
SAMHD1 is a deoxynucleotide-triphosphate (dNTP) hydrolase (dNTPase) that plays a key role in dNTP homeostasis. Overexpression of SAMHD1 in the AML cell line, THP-1, inhibits cell proliferation and induces apoptosis [Citation12,Citation13]. Thus, increased levels of SAMHD1 could result in depletion of the dNTP pool and impede replication/repair of DNA that may be damaged due to increased intracellular ROS. Determination of the expression of SAMHD1 in the three AML cells revealed that expression of this protein varied between these cell types, KG1 < AML10 < HL-60 (). Treatment of KG1 and AML 10 blast cells with Dex plus ATRA led to 1.5-fold increased expression of SAMHD1 protein () as compared to treatment with ATRA alone. In the AML 10 cells ATRA alone led to significant induction of SAMHD1 protein, compared to control cells and cells treated with Dex. ATRA led to a similar increase in SAMHD1 protein in HL-60 cells, which was not further enhanced in the presence of Dex (). These results suggest that SAMHD1 may not be directly involved in enhanced apoptosis observed in the presence of Dex plus ATRA. However, when topo II function is compromised by Dex, increased activity of SAMHD1 may assist in the increased apoptotic response. In that regard a specific threshold of SAMHD1 may be important, which can be achieved due to increases in SAMHD1 activity by ATRA and/or Dex plus ATRA.
It has been shown that transcriptional activation of genes by several intracellular receptors, including retinoic acid and estrogen receptor complex, requires a ROS- and topo IIβ-mediated dsDNA break at transcriptional start sites that facilitates controlled DNA damage/repair, thereby allowing for bending of DNA and access to the transcriptional machinery [Citation14,Citation15]. Therefore, a likely mechanism for enhanced Dex plus ATRA-induced apoptosis involves impairment of ATRA-induced DNA damage/repair cycle, such that the initial ROS-induced DNA damage by ATRA at transcriptional start sites of ATRA-regulated genes cannot be repaired when topo IIβ is degraded by Dex and dNTP pools are depleted following increased SAMHD1 activity, resulting in increased apoptosis. The increased ROS observed with Dex plus ATRA could result from persistent DNA damage that can further influence apoptosis, or could be a result of increased mitochondrial caspase activities.
In summary the present results support clinical evaluation of the combination of Dex plus ATRA in patients with relapsed refractory AML and ATRA-resistant APL, given that Dex and ATRA are both clinically approved. Dex, which is an effective cardioprotectant for anthracycline-based chemotherapy in AML patients, offers a potentially less toxic option when combined with ATRA for treating AML patients, especially older patients who cannot tolerate toxic chemotherapeutic regimens. Since the clinical efficacy of ATRA, either alone or in combination with other chemotherapeutic agents, in AML may be limited to specific AML-subtypes, identification of Dex plus ATRA responsive AML subtypes (assisted by the ATRA-21 responsive score) would allow for selection of the appropriate patient population to benefit from this combination therapy.
Author contributions
E.J.N., Y.C.P., R.N.G., and M.K.G. designed the study, performed and analyzed the data and wrote the manuscript; K.E.K. conducted and analyzed data, N.S. conducted and analyzed the microarray data; W.D.J. analyzed the microarray data; J.P.M. selected patient AML samples and provided patient care; all authors read and reviewed the manuscript.
Supplemental Material
Download MS Word (796.6 KB)Disclosure statement
Wendell D. Jones is an employee of Q2 Solutions/EA Genomics. All other authors declare no conflict of interest.
References
- Kakizuka A, Miller WH, Jr, Umesono K, et al. Chromosomal translocation t(15;17) in human acute promyelocytic leukemia fuses RAR alpha with a novel putative transcription factor, PML. Cell. 1991;66(4):663–674.
- Lo-Coco F, Avvisati G, Vignetti M, et al. Retinoic acid and arsenic trioxide for acute promyelocytic leukemia. N Engl J Med. 2013;369(2):111–121.
- Schlenk RF, Döhner K, Kneba M, et al. Gene mutations and response to treatment with all-trans retinoic acid in elderly patients with acute myeloid leukemia. Results from the amlsg trial aml hd98b. Haematologica. 2009;94(1):54–60.
- Burnett AK, Hills RK, Green C, et al. The impact on outcome of the addition of all-trans retinoic acid to intensive chemotherapy in younger patients with nonacute promyelocytic acute myeloid leukemia: overall results and results in genotypic subgroups defined by mutations in NPM1, FLT3, and CEBPA. Blood. 2010;115(5):948–956.
- Martelli MP, Gionfriddo I, Mezzasoma F, et al. Arsenic trioxide and all-trans retinoic acid target NPM1 mutant oncoprotein levels and induce apoptosis in NPM1-mutated AML cells. Blood. 2015;125(22):3455–3465.
- Bolis M, Terao M, Pattini L, et al. The ATRA-21 gene-expression model predicts retinoid sensitivity in CEBPA double mutant, t(8;21) and inv(16) AML patients. Blood Cancer J. 2019;9(10):76.
- Lubbert M, Grishina O, Schmoor C, et al. Valproate and retinoic acid in combination with decitabine in elderly nonfit patients with acute myeloid leukemia: results of a multicenter, randomized, 2 × 2, phase II trial. J Clin Oncol. 2020;38:257–270.
- Chikamori K, Hill JE, Grabowski DR, et al. Downregulation of topoisomerase IIbeta in myeloid leukemia cell lines leads to activation of apoptosis following all-trans retinoic acid-induced differentiation/growth arrest. Leukemia. 2006;20(10):1809–1818.
- Reichardt P, Tabone MD, Mora J, et al. Risk-benefit of dexrazoxane for preventing anthracycline-related cardiotoxicity: re-evaluating the European labeling. Future Oncol. 2018;14(25):2663–2667.
- Getz KD, Sung L, Alonzo TA, et al. Effect of dexrazoxane on left ventricular systolic function and treatment outcomes in patients with acute myeloid leukemia: a report from the children’s oncology group. J Clin Oncol. 2020;38(21):2398–2406.
- Yen A, Roberson MS, Varvayanis S, et al. Retinoic acid induced mitogen-activated protein (MAP)/extracellular signal-regulated kinase (ERK) kinase-dependent MAP kinase activation needed to elicit HL-60 cell differentiation and growth arrest. Cancer Res. 1998;58(14):3163–3172.
- Kodigepalli KM, Li M, Liu SL, et al. Exogenous expression of SAMHD1 inhibits proliferation and induces apoptosis in cutaneous T-cell lymphoma-derived Hut78 cells. Cell Cycle. 2017;16(2):179–188.
- Kodigepalli KM, Bonifati S, Tirumuru N, et al. SAMHD1 modulates in vitro proliferation of acute myeloid leukemia-derived THP-1 cells through the PI3K-Akt-p27 axis. Cell Cycle. 2018;17(9):1124–1137.
- Ju B-G, Lunyak VV, Perissi V, et al. A topoisomerase IIbeta-mediated dsDNA break required for regulated transcription. Science. 2006; 312(5781):1798–1802.
- Perillo B, Ombra MN, Bertoni A, et al. DNA oxidation as triggered by H3K9me2 demethylation drives estrogen-induced gene expression. Science. 2008;319(5860):202–206.