ABSTRACT
In China, the areas that are undergoing rapid urban growth are faced with increasingly more complicated air pollution problems. Sources of air pollution need to be identified and their contributions quantified. In this study, PM2.5 (particulate matter with aerodynamic diameters ≤2.5 μm), PM2.5–10 (particulate matter with aerodynamic diameters 2.5–10 μm), organic carbon (OC), and elemental carbon (EC) concentrations were measured from April to July 2009 at four selected areas in Xiamen (the downtown area, an industrial park, a suburb, and one remote site). The contributions of carbonaceous aerosols to PM2.5 and PM2.5–10 were 20–30% and 10–20%, respectively, indicating that finer particles contained more carbonaceous aerosols. The EC concentrations in PM2.5 at the downtown, industrial, suburb, and remote sites were 2.16 ± 0.61, 2.05 ± 0.45, 1.69 ± 0.54, and 0.65 ± 0.43 μg m−3, respectively, showing a decrease from the urban and industrial hotspots to the surrounding areas. These data show that carbonaceous aerosols emitted from the combustion of fossil fuels in urban and industrial hotspots influence air quality at the regional scale. Higher levels of PM2.5 and PM2.5–10 were observed at the suburb site compared to the urban and industrial sites. Peak EC concentrations in PM2.5 were observed during the morning and evening rush hours. However, peak PM2.5 levels at the suburb site were observed around noon, which coincides with construction work hours, instead of the morning and evening rush hours when emissions from combustion dominated. These findings indicate that both fuel combustion and construction have exacerbated air pollution in coastal and urban areas in China.
Over the past two decades, China has been experiencing arguably the most rapid, dramatic, and far-reaching process of urbanization in human history. Despite the rising concern for air quality in coastal urbanized areas, emission sources of air pollutants have yet to be distinguished. Herein the authors demonstrate that emissions from construction and combustion have intensified air pollution in coastal areas. This paper addresses an important environmental issue that the public and policy makers should consider for the management of air pollution.
INTRODUCTION
Ambient particulate matter with aerodynamic diameters <2.5 μm (PM2.5) and between 2.5 and 10 μm (PM2.5–10) imposes high risks to the human respiratory system and is responsible for many diseases.Citation1,Citation2 Increased mortality and morbidity in communities with elevated particulate matter concentrations have been reported in a variety of epidemiological studies.Citation2–4 Besides these human impacts, trace elements associated with ambient particulate matter can be deposited on soils and in coastal waters and have potentially adverse consequences for ecosystem health.Citation5
Anthropogenic emissions are largely responsible for the enhancement of particulate matter concentrations and air pollution. Major anthropogenic sources include fossil fuel combustion in industry, transportation, and habitation; biomass burning in agriculture and deforestation; and human activities such as construction, mining, and agriculture.Citation6,Citation7 Elemental carbon (EC) is directly emitted from fossil and biomass combustion and undergoes little chemical transformation; therefore, it is a good indicator of primary combustion emission. Organic carbon (OC) is another important particulate component of combustion emission. OC can be emitted directly in particulate form (primary) or formed in the atmosphere from semivolatile and low-volatility products of chemical reactions involving reactive organic gases (secondary). Soils disturbed during construction, mining, and agriculture contribute to the inorganic component (which is mineral dominated) of particulate matter.
The intensive study of air pollution in well-developed megacities in China, such as Beijing, Shanghai, Guangzhou, and Hong Kong, is due to recent improvement in environmental management.Citation8–10 However, the rapid urbanization of China has led to new environmental problems in suburban areas. The sustainability of both urban and rural areas is affected by the dynamic and ever-changing flows of commodities, capital, natural resources, people, and pollution in the suburbs. Suburban areas experience a dynamic mix of functions and land uses, increasing population and building densities, landscape modification, and exposure to various pollutants.Citation11
To assess the sources of anthropogenic emissions in coastal and urban areas of China, we conducted this study in Xiamen. Daily average levels of PM2.5, PM2.5–10, OC, and EC were measured from samples collected in four areas of Xiamen. Intensive monitoring work was conducted in the suburban area to highlight the impact of urbanization on air pollution.
METHODS
Research Area
Xiamen, which is located in the southeastern coastal area of China, is a special economic zone that connects the Pearl River Delta, the Yangtze River Delta, and Taiwan (). The whole region has a southern subtropical monsoon climate, with an annual mean temperature of 20.8 °C. During the winter monsoon season, the northeastern airflow from inland China brings cold and dry air to Xiamen, whereas the summer monsoon brings the southwesterly airflow, brisk wind, and marine air mass. Annual precipitation is 1143.5 mm, of which 80% occurs from March to September and the maximum occurs from June to August (summer monsoon maximum).
Daily Samples and OC and EC Measurements
From April to July 2009, a total of 42 aerosol samples were collected in four sampling areas in Xiamen: the downtown area (N24°26′, E118°5′; N24°29′, E118°6′), an industrial park (N24°29′, E118°2′), a suburb (N24°37′, E118°3′), and a remote site (N24°49′, E118°9′). PM2.5 and PM2.5–10 were deposited on quartz filters (Whatman, Quartz Microfibre Filters, Kent, UK) mounted on an atmospheric sampler. A volumetric air flow rate of 100 L min−1 was applied during sampling intervals of 24 hr, and an air volume of 144 mCitation3 was sampled. Quartz filters were precombusted at 450 °C for 4 hr. The filters were weighed (0.1 mg accuracy) before and after sampling after conditioning in an electronic desiccator at 25 °C and relative humidity of 50% for 24 hr. The sample filters were stored in a refrigerator before analysis.
A circular filter (1.6 cm diameter) was cut from the sample filter and analyzed with an automated semicontinuous thermal-optical transmittance carbon analyzer (Sunset Laboratory, Inco, Portland, OR, USA). OC was released from volatilization of particulate carbon thermally and oxidized to carbon dioxide (CO2) that was simultaneously monitored by a nondispersive infrared detector. EC in the filter was thermally oxidized to CO2 following the OC volatilization. Because some OC in the filter was pyrolitically converted to EC instead being directly volatilized, a diode laser and photodetector monitored the transmittance of light through the filter during analysis to correct for the pyrolysis of OC. See GeronCitation12 for detailed descriptions of the measurements of OC and EC concentrations. Replicate analyses of sucrose standard and filter samples showed an uncertainty of ∼10% for OC and EC measurements. The concentrations of PM2.5, PM2.5–10, EC, and OC were reported as mass in a unit volume of air (μg m−3).
Intensive Monitoring of PM2.5, OC, and EC at the Suburb Site
In China, the public has become concerned about the worsening air quality that is associated with the rapid expansion of urbanized areas. The suburban area in Xiamen, which covers an area of ∼215 kmCitation2, has experienced rapid expansion of urbanized area (from 27.8 to 63.6 km2) during the past two decades. PM2.5 was measured on the roof of a 30-m-high building in the suburban area of Xiamen using a real-time 1400-D tapered element oscillating microbalance (TEOM) monitor (Thermo Scientific, Franklin, MA, USA). The size fraction of PM2.5 was obtained using 2.5-μm cyclone inlets, inlet humidity and temperature control systems, and dedicated sampling lines in the TEOM monitor.Citation13 A total of 2500 measurements of 30-min averaged concentrations of PM2.5 were made from March to May 2009. The uncertainty was about 1% for the 30-min averaged concentration values.
Two-hour averaged concentrations of OC and EC in PM2.5 also were measured using an automated semicontinuous thermaloptical analyzer that was installed on the 30-m-high building. Air was pumped at a flow rate of 8 L min−1 through the 2.5-μm cyclone inlet of the analyzer. Gas-phase organic compounds in the airstream were removed by a parallel-plate carbon impregnated fiber denuder (Sunset Laboratory Inc., Portland, OR, USA). PM2.5 in the airstream was deposited on a 1.6-cm circular quartz filter and automatically analyzed for OC and EC concentrations every 2 hr. A total of 300 measurements were recorded from 19 July to 16 August 2009. The detection limits for the analyzer were ∼0.2 mg carbon.
RESULTS AND DISCUSSION
Daily Levels of PM2.5, PM2.5–10, OC, and EC
Table 1 shows the daily levels of PM2.5, PM2.5–10, EC, and OC and their ratios at the downtown, industrial, suburb, and remote sites of Xiamen. The mean concentrations of PM2.5 at these sites ranged between 37 and 44 μg m−3, and these values were considerably higher than those of PM2.5–10 (20–32 μg m−3). The highest levels of PM2.5 and PM2.5–10 were detected at the suburb site, not in the urban and industrial areas. Mean levels of PM2.5 in Xiamen were lower than those in Beijing (40–90 μg m−3)Citation14 but higher than those in Europe (12–35 μg m−3).Citation15
Mean EC concentrations in PM2.5 were 2.16 ± 0.61, 2.05 ± 0.45, 1.69 ± 0.54, and 0.65 ± 0.43 μg m−3 at the downtown, industrial, suburb, and remote sites, respectively. These data show a decrease from the urban and industrial hotspots to the suburban and rural areas. The higher level of EC at the urban and industrial sites indicates significant emissions from fuel combustion in traffic, habitation, and industry. Anthropogenic emissions from the urban and industrial areas dispersed to the surrounding areas, as indicated by the decreasing levels of EC in relation with increasing distance from the urban and industrial hotspots.
The OC concentrations in PM2.5 ranged from 4.03 to 5.78 μg m–3, and they were higher than those in PM2.5–10 (1.63–2.68 μg m−3). PM2.5–10 contained a considerable amount of OC but much lower concentrations of EC (average of 0.21 ± 0.16 μg m−3 for the four sampling areas). About 75% of total particulate carbon in the air pollution was in PM2.5, suggesting that the majority of carbonaceous aerosols are contained in fine size fraction. This result excludes the possibility that combustion emission is a primary source of the coarse particles.
Mean ratios of OC/EC in PM2.5 at the downtown, industrial, suburb, and remote sites were 2.07 ± 1.37, 2.90 ± 1.04, 3.06 ± 1.29, and 12.69 ± 12.37, respectively. The lowest mean occurred in the downtown area, whereas the remote site had both the highest mean and the largest variability for OC/EC ratios in different samples. The dominance of traffic emissions in the downtown area accounted for carbonaceous aerosols with low OC/EC ratios.Citation16 The high mean value and wide variability of the OC/EC ratios in samples from the remote site likely represent wildfire emission, the formation of secondary organic carbon (SOC), and/or direct emission from biogenic origins. Uncontrolled fire or open burning for the disposal of solid wastes in the remote area may emit carbonaceous aerosols with high OC/EC ratios.Citation17 Biogenic availability of gaseous organic precursors in the remote area could have promoted the formation of SOC.Citation12 Direct emission of biogenic materials, such as pollen (OC dominant), also may gave contribution to the coarse particulate matter in the remote area, as OC/EC ratios were higher in PM2.5–10 than in PM2.5.
The levels of PM2.5 and OC tended to decrease from April to July, whereas EC concentrations were relatively constant. The decreased levels of OC and PM2.5 may have been due to increased washout from greater rainfall and/or dispersion from marine airflow during the summer monsoon maximum. Backward-trajectory analysis in Xiamen (data not shown) indicated shifting of continental to marine airflows from April to July. The constant level of EC suggested a balance between emission and dispersion of primary carbonaceous aerosol during the summer monsoon season. Marine airflow should promote air pollution dispersion, but a certain amount of EC in the atmosphere may have a long lifetime.
Intensified Air Pollution Accompanies Urbanization
The contributions of carbonaceous mass to PM2.5 (CM/PM2.5; CM = EC + 1.6 × OC)Citation18 () varied from 20% to 30%; for PM2.5–10 the contributions were 10–20%. The lowest values occurred at the suburb site. Samples from the suburban area had the lowest percentages of carbonaceous matter but the highest levels of PM2.5 and PM2.5–10. The particulate air pollution in the suburbs may have some specific origins in addition to fuel combustion.
Table 1. Daily levels of PM2.5, PM2.5–1 0, EC, OC and their ratios for samples from the downtown, industrial, suburb, and remote sites in Xiamen
shows the temporal trends of the intensive monitoring data for concentration of PM2.5 and EC and OC at the suburb site of Xiamen. These temporal trends were generally consistent with those seen in the daily data from the four sampling areas in Xiamen (), which suggests a regional dispersion via atmospheric circulation. The lower level of OC measured with intensive monitoring (1.79 ± 0.50 μg m−3) compared to daily measurements (4.68 ± 1.71 μg m−3) at the suburb site may be explained by the decrease in OC concentration that occurred from April to July. The small differences in EC observed between intensive monitoring (1.62 ± 0.21 μg m−3) and daily measurement (1.76 ± 0.76 μg m−3) data were reasonable because of the relatively constant temporal trend of EC in Xiamen.
Figure 2. Temporal trends of the intensive monitoring data for 30-min averaged concentration of PM2.5 (a) and 2-hr averaged concentration of EC and OC (b) at the suburb site of Xiamen.
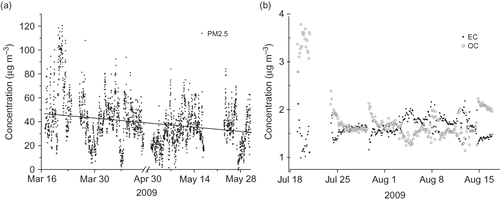
shows average diurnal profiles of PM2.5, EC, and OC for the intensive monitoring at the suburb site. EC concentrations in PM2.5 peaked during the morning and evening traffic rush hours. This diurnal pattern in EC concentration at the suburb site was similar to that in urban areas,Citation19 which indicates that this pattern is due to the contribution of traffic emission. The diurnal OC pattern differed from that of EC: OC concentrations were higher at night and around noon. Photochemical reactions and noncombustion emission may partly explain the diurnal variation in OC.Citation20 PM2.5 levels at the suburb site peaked at late morning and afternoon, thus this pattern differed from that of both EC and OC. The diurnal PM2.5 peaks at the suburb site were not due to either primary or secondary emissions of carbonaceous aerosol but instead can be explained by soil dust particles. The suburban areas, front of urban expansion, had undergone extensive landscape modification and construction for urbanization that made the emission of soil dust particles during working interval in daytime. The extensive loading of fine and coarse particulate matter in the suburbs may be largely due to the emission of soil dust particles triggered by landscape modification and massive construction work for urbanization.
Figure 3. Average diurnal profiles of PM2.5 (a), EC (b), and OC (c) for the intensive monitoring at the suburb site. Part a was generated from data of 30-min averaged concentrations of PM2.5 from March to May 2009; parts b and c were from data of 2-hr averaged concentrations of EC and OC from 24 July to 16 August 2009.
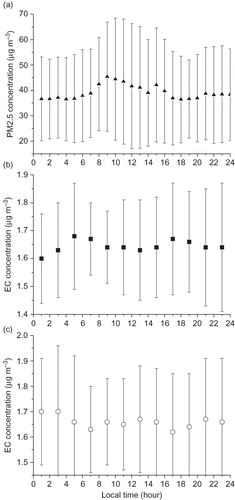
Over the past two decades, China has been experiencing arguably the most rapid, dramatic, and far-reaching process of urbanization (from 17.4% to 41.8%) in human history.Citation11 Although concerns about the adverse impact of urbanization on eco-environmental systems have increased, the suburban areas associated with intensive urbanization generally have been ignored in environmental management plans. The extent of landscape modification has led to deforestation, overpopulation, topsoil degradation and erosion, and air pollution. These factors affect not just large cities such as Beijing, where widespread respiratory illness has focused attention on the problem, but also suburban areas, which have received little attention.
CONCLUSIONS
The data in this study indicate that fuel combustion and construction work for urbanization are two major emission sources for particulate air pollution in coastal and urban areas of China. From April to July 2009, the EC and OC concentrations in PM2.5 were 1.8 ± 0.8 and 4.7 ± 1.7 μg m−3, respectively; these values were much higher than those in PM2.5–10 (0.2 ± 0.2 and 1.9 ± 0.7 μg m−3, respectively). Carbonaceous mass contributed 25% ± 7% to PM2.5 but only 13% ± 6% to PM2.5–10, which suggests that carbonaceous aerosols are more abundant in the finer size ranges of particles. EC concentrations decreased from the downtown and industrial sites to the suburb and remote sites. Thus, anthropogenic emission from fuel combustion in the urban and industrial hotspots had a significant effect on regional air pollution. The significantly higher level of construction emission during the daytime caused the highest levels of PM2.5 and PM2.5–10 in the suburbs compared to other areas. Although emission sources might not be fully evaluated in this study, the results suggest that anthropogenic emissions of carbonaceous aerosols and soil dust particles from rapid urbanization in China have largely intensified particulate air pollution. Moreover, the intensive emissions from combustion and construction in Asia likely has significance for the global atmosphere.Citation21
ACKNOWLEDGMENTS
Mr. Wenquan Wu and Jieru Zhang helped on field work. We acknowledge Dr. Ke Du for useful comments and revisions. This research was financially supported by National Natural Science Foundation of China (no. 40905065), Ministry of Education of China, and the Knowledge Innovation Program of the Chinese Academy of Sciences (no. KZCX2-YW-422-4). The authors acknowledge the NOAA Air Resources Laboratory (ARL) for the provision of the HYSPLIT transport and dispersion model and/or READY Web site (http://www.arl.noaa.gov/ready.html) used in this publication.
REFERENCES
- Pope , C.A. III and Dockery , D.W. 2006 . Health Effects of Fine Particulate Air Pollution: Lines That Connect . J. Air Waste Manage. Assoc. , 56 : 709 – 742 .
- de Kok , T.M.C.M. , Driece , H.A.L. , Hogervorst , J.G.F. and Briedé , J.J. 2006 . Toxicological Assessment of Ambient and Traffic-Related Particulate Matter: A Review of Recent Studies . Mutat. Res. , 613 : 103 – 122 .
- Pope , C.A. III. 2000 . Epidemiology of Fine Particulate Air Pollution and Human Health: Biologic Mechanisms and Who's at Risk? . Environ. Health Perspect. , 108 : 713 – 723 .
- Schwarze , P.E. , Øvrevik , J. , Låg , M. , Refsnes , M. , Nafstad , P. , Hetland , R.B. and Dybing , E. 2006 . Particulate Matter Properties and Health Effects: Consistency of Epidemiological and Toxicological Studies . Hum. Exp. Toxicol. , 25 : 559 – 579 .
- Jurado , E. , Dachs , J. , Duarte , C.M. and Simó , R. 2008 . Atmospheric Deposition of Organic and Black Carbon to the Global Oceans . Atmos. Environ. , 42 : 7931 – 7939 .
- Chow , J.C. , Watson , J.G. , Lowenthal , D.H. , Solomon , P.A. , Magliano , K.L. , Ziman , S.D. and Richards , L.W. 1993 . PM10 and PM2.5 Compositions in California's San Joaquin Valley . Aerosol Sci. Technol. , 18 : 105 – 128 .
- Gustafsson , Ö , Kruså , M. , Zencak , Z. , Sheesley , R.J. , Granat , L. , Engström , E. , Praveen , P.S. , Rao , P.S.P. , Leck , C. and Rodhe , H. 2009 . Brown Clouds over South Asia: Biomass or Fossil Fuel Combustion . Science , 323 : 495 – 498 .
- Cao , J.J. , Lee , S.C. , Ho , K.F. , Zhang , X.Y. , Zou , S.C. , Chow , F.K. , Judith , C.J. and Watson , G. 2003 . Characteristics of Carbonaceous Aerosol In Pearl River Delta Region, China during 2001 Winter Period . Atmos. Environ. , 37 : 451 – 460 .
- Fang , G. , Wu , Y. , Chou , T. and Lee , C. 2008 . Organic Carbon and Elemental Carbon in Asia: A Review from 1996 to 2006 . J. Hazard. Mater. , 150 : 231 – 237 .
- Parrish , D.D. and Zhu , T. 2009 . Clean Air for Megacities . Science , 326 : 674 – 675 .
- Simon , D. 2008 . Urban Environments: Issues on the Peri-urban Fringe . Annu. Rev. Environ. Resour. , 33 : 167 – 185 .
- Geron , C. 2009 . Carbonaceous Aerosol over a Pinus Taeda Forest in Central North Carolina, USA . Atmos. Environ. , 43 : 959 – 69 .
- 13 DeGaetano , A.T. and Doherty , O.M. 2004 . Temporal, Spatial and Meteorological Variations in Hourly PM2.5 Concentration Extremes in New York City . Atmos. Environ. , 38 : 1547 – 1558 .
- Zhao , X. , Zhang , X. , Xu , X. , Xu , J. , Meng , W. and Pu , W. 2009 . Seasonal and Diurnal Variations of Ambient PM2.5 Concentration in Urban And Rural Environments in Beijing . Atmos. Environ. , 43 : 2893 – 2900 .
- Querol , X. , Alastuey , A. , Moreno , T. , Viana , M.M. , Castillo , S. , Pey , J. , Rodríguez , S. , Artiñano , B. , Salvador , P. , Sánchez , M. , Garcia Dos Santos , S. , Herce Garraleta , M.D. , Fernandez-Patier , R. , Moreno-Grau , S. , Negral , L. , Minguillón , M.C. , Monfort , E. , Sanz , M.J. , Palomo-Marín , R. , Pinilla-Gil , E. , Cuevas , E. , de la Rosa , J. and Sánchez de la Campa , A. 2008 . Spatial and Temporal Variations in Airborne Particulate Matter (PM10 and PM2.5) across Spain 1999–2005 . Atmos. Environ. , 42 : 3964 – 3979 .
- Jones , A.M. and Harrison , R.M. 2005 . Interpretation of Particulate Elemental and Organic Carbon Concentrations at Rural, Urban and Kerbside Sites . Atmos. Environ. , 39 : 7114 – 7126 .
- Shen , Z.X. , Cao , J.J. , Arimoto , R. , Zhang , R.J. , Jie , D.M. , Liu , S.X. and Zhu , C.S. 2007 . Chemical Composition and Source Characterization of Spring Aerosol over Horqin Sand Land in Northeastern China . J. Geophys. Res. , 112 ( D14 ) : D14315
- Turpin , B.J. and Lim , H.J. 2001 . Species Contributions to PM2.5 Mass Concentrations: Revisiting Common Assumptions for Estimating Organic Mass . Aerosol Sci. Technol. , 35 : 602 – 610 .
- Saha , A. and Despiau , S. 2009 . Seasonal and Diurnal Variations of Black Carbon Aerosols over a Mediterranean Coastal Zone . Atmos. Res. , 92 : 27 – 41 .
- Kroll , J.H. and Seinfeld , J.H. 2008 . Chemistry of Secondary Organic Aerosol: Formation and Evolution of Low-Volatility Organics in the Atmosphere . Atmos. Environ. , 42 : 3593 – 3624 .
- Randel , W.J. , Park , M. , Emmons , L. , Kinnison , D. , Bernath , P. , Walker , K.A. , Boone , C. and Pumphrey , H. 2010 . Asian Monsoon Transport of Pollution to the Stratosphere . Science , 328 : 611 – 613 .