ABSTRACT
The long-term environmental impact of municipal solid waste (MSW) landfilling is still under investigation due to the lack of detailed characterization studies. A MSW landfill site, popularly known as Dhapa, in the eastern fringe of the metropolis of Kolkata, India, is the subject of present study. A vast area of Dhapa, adjoining the current core MSW dump site and evolving from the raw MSW dumping in the past, is presently used for the cultivation of vegetables. The inorganic chemical characteristics of the MSW-contaminated Dhapa surface soil (covering a 2-km stretch of the area) along with a natural composite (geogenic) soil sample (from a small countryside farm), for comparison, were investigated using two complementary nondestructive analytical techniques, energy-dispersive X-ray fluorescence (EDXRF) for bulk analysis and low-Z (atomic number) particle electron probe X-ray microanalysis (low-Z particle EPMA) for single-particle analysis. The bulk concentrations of K, Rb, and Zr remain almost unchanged in all the soil samples. The Dhapa soil is found to be polluted with heavy metals such as Cu, Zn, and Pb (highly elevated) and Ti, Cr, Mn, Fe, Ni, and Sr (moderately elevated), compared to the natural countryside soil. These high bulk concentration levels of heavy metals were compared with the Ecological Soil Screening Levels for these elements (U.S. Environment Protection Agency) to assess the potential risk on the immediate biotic environment. Low-Z particle EPMA results showed that the aluminosilicate-containing particles were the most abundant, followed by SiO2, CaCO3-containing, and carbonaceous particles in the Dhapa samples, whereas in the countryside sample only aluminosilicate-containing and SiO2 particles were observed. The mineral particles encountered in the countryside sample are solely of geogenic origin, whereas those from the Dhapa samples seem to have evolved from a mixture of raw dumped MSW, urban dust, and other contributing factors such as wind, precipitation, weather patterns, farming, and water logging, resulting in their diverse chemical compositions and the abundant observation of carbonaceous species. Particles containing C and P were more abundant in the Dhapa samples than in the countryside soil sample, suggesting that MSW-contaminated soils are more fertile. However, the levels of particles containing potentially toxic heavy metals such as Cr, Mn, Ni, Cu, Zn, and/or Pb in the Dhapa samples were significant, corroborated by their high bulk concentration levels (EDXRF), causing deep concern for the immediate environment and contamination of the food chain through food crops.
Raw MSW dumping over the past decades has made the topsoil of the Kolkata landfill site nutrient-rich, with elevated levels of carbonaceous species and essential elements such as P, K, Ca, etc., compared to the co-characterized natural soil. This may apparently encourage vegetable cultivation. However, the higher concentrations of heavy metals such as Cr, Cu, Zn, Pb, etc., in the surface soil could adversely affect the food chain and the immediate environment. The associations of minor elements such as Na, Mg, K, Ca, Ti, Fe, etc., with aluminosilicate-containing soil particles may be indicative of the soil chemical environment. Some soil remedial measures for heavy metal contaminants have to be implemented.
INTRODUCTION
Municipal solid waste (MSW) management has been slowly but systematically evolving into an integrated solid waste management (ISWM) system over the past few decades. For the urban and the suburban municipal authorities, this integrated management system includes (i) segregation and collection of municipal waste at source; (ii) further reduction in volume by incineration of the high-calorie part for energy recovery; (iii) composting of the organic part for use as fertilizers; (iv) recycling; and (v) scientific pretreatment of the remaining bulk MSW followed by disposal at engineered sanitary landfill sites.Citation1 This ISWM action plan has to be backed up by thorough environmental impact assessment (EIA) to ensure its sustainability. Systematic and intensive investigations have been carried out in this direction, mostly in developed countries. For example, studies have included characterization of raw MSW, source-separated MSW, incineration ash, post-MBT (mechanical biological treatment) compost, mixed-waste compost, and the effects on public health and environment.Citation2–5 However, the characteristics of MSW are different among different countries and cities, as they are heavily dependent on the economic levels, social structures, and ethnic distributions of specific regions where MSWs are generated.Citation6 The characterization studies of MSW, therefore, need to be region-specific.
In developing countries such as India where the population has been increasing and industrialization and urban expansion continuing, the lack of updated infrastructure and public awareness has made the solid waste management (SWM) scenario grim.Citation7,Citation8 Economically and cognitively heterogeneous population distribution, especially in the major metropolitan cities, has further compounded the problem. Except for a few municipalities, the usual practice has been indiscriminate dumping or open landfilling of raw MSW in low lying areas, and even near catchments for bodies of water. The short- and long-term impact of this practice cannot be predicted without detailed knowledge of the pollutant species present in the MSW in a particular region. Besides the characterization of MSW with a particular emphasis on the chemical species involved, it is also important to perform systematic chemical characterization of soils that have been historically dumped with raw MSW. The characterization and chemical mapping of the soil evolving from solid waste will provide a vital tool in predicting the long-term environmental impact.
In this study, the surface soil at a unique MSW dumping site in Kolkata, India, where so-called man-made or anthropogenic soil has itself been evolving from long-term dumping of raw MSW, was investigated. The metropolitan city of Kolkata (area, 187 kmCitation2; population, 8 million) generates MSW at an average rate of 3000 tons per day.Citation9,Citation10 Since the middle of the 19th century, an area in the eastern fringe of the city, “Dhapa”, has been earmarked for the dumping of the MSW generated in the city and its suburbs. Dumping of MSW still continues in this area, although with the passage of time the core site of dumping activity has shifted more eastwards. The vast stretch of land adjoining the core area (around 2000 acres), which is presently used for farming, is bound by a bypass highway running north to south along the western border and the East Kolkata Wetlands (). In our previous study, it was observed that a composite MSW sample from the dump site in Dhapa contained the major elements: K, Ca, and Fe at approximate concentrations of 2–3%, 3–4%, and 2–3%, respectively; the minor element: Ti, 0.4–0.5%; and the trace elements: Mn, Cu, Zn, Rb, Sr, Zr, and Pb with approximate concentrations of 450, 100, 350, 80, 180, 250, and 350 mg/kg dry weight, respectively.Citation11 The topsoil at this site, which has evolved or is evolving with the decay of raw MSW, is hence expected to contain elevated levels of these elemental species, including heavy and toxic metals, above their respective background concentrations in natural soils of geogenic origin. The toxic elements may accumulate in the food chain, primarily through the vegetables grown on these garbage farms at Dhapa.Citation12–14 The toxic elements could also propagate further underground through leaching. A thorough survey of the elemental and chemical species constituting the topsoil is needed to understand the ecological implications and thereby facilitate remedial measures.
Figure 1. Satellite map of the sampling site for MSW-contaminated soils, Dhapa (sampling points: Dh1–Dh6). Inset: Kolkata metropolitan area map with countryside (Csl) sampling point (courtesy: Wiki-mapia and KMDA).
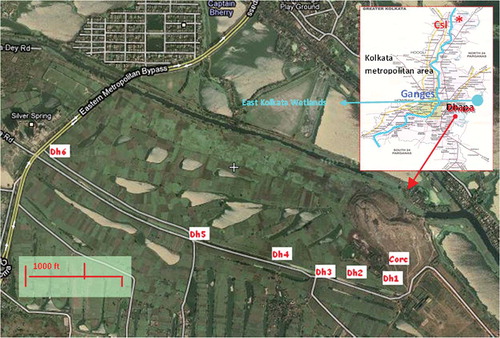
The current dumping site was taken as the starting “core” of the soil sampling. Surface soil samples were collected at certain progressive intervals starting near the core and ending near the bypass highway about 2000 m west from the core (samples Dh1–Dh6; see ). A composite surface soil sample was also collected from a small countryside farm around 50 km away from the city. The basic or general soil type in the lower Gangetic belt or Delta region of the State of West Bengal, India (which includes the urban and suburban areas around Kolkata city as well as the countryside sampling area in consideration here), is alluvial soil with the naturally formed topsoil having a “sandy loam” texture.Citation15 Hence, the countryside soil, considered as a control soil or a natural benchmark, was analyzed along with the MSW-contaminated soils in the present study.
Two different nondestructive (without chemical perturbation of the constituents) analytical methods for bulk elemental and single-particle analyses were employed to acquire firsthand qualitative and quantitative knowledge about the inorganic chemical constituents of the soil samples. Firstly, energy-dispersive X-ray fluorescence (EDXRF), which is a proven tool for determining bulk elemental concentrations in soil samples,Citation16,Citation17 was used to obtain the absolute mass fractions of the elements in the MSW-contaminated samples and also the control soil sample. The 109Cd-Si(Li) EDXRF setup detects elements with characteristic X-rays in the range 3–20 keV, viz. K–Mo (K X-rays) and Pb, Hg, etc. (L X-rays). Secondly, quantitative energy-dispersive electron probe X-ray microanalysis (ED-EPMA), known as low-Z (atomic number) particle EPMA, was applied for the single-particle characterization of MSW-contaminated and countryside soil samples. By the application of low-Z particle EPMA, the chemical compositions, including low-Z elements such as C, N, and O, of individual particles can be elucidated. Because low-Z particle EPMA can clearly identify carbonate, sulfate, and nitrate particles, it has been proven to be useful for the characterization of diverse environmental aerosol samples.Citation18–22 The combined application of these two techniques for the characterization of river sediments polluted with heavy metals has been reported to be quite successful.Citation23–25 In this work, soils collected at MSW-contaminated and apparently noncontaminated countryside sites were investigated using these two nondestructive techniques for the detailed characterization of their inorganic chemical compositions.
EXPERIMENTAL
Samples
Six surface-soil samples (Dh1–Dh6) were collected at six locations in Dhapa (22°32′N, 88°24′E) between June 2007 and January 2008, starting near the present core of MSW dumping and ending near the eastern metropolitan bypass highway, stretching 2000 m (). An apparently noncontaminated composite surface-soil sample (Csl) was collected during January 2008 from a small countryside farm located approximately 50 km away from Kolkata (22°58′N, 88°29′E). All the soil samples from Dhapa (Dh1–Dh6) were dark brown in color and somewhat sticky (this may be due to elevated presence of humic or organic substances along with the mineral particles), with good binding capacity. The Csl soil (a composite sample) was light yellowish brown in color, weakly granular, and loose when dry; it looked like sandy loam textured topsoil, the typical soil type of this region.Citation15
The samples were subjected to air drying and then sieved using a 9-mesh (<2 mm Ø) sieve. Each soil sample was scaled down in size by the use of a quartering technique to obtain a representative sample for each location, which was divided into two parts. One part of each sample was kept in a polyethylene container and stored in a desiccator before pulverization and pelletization for EDXRF analysis. The second parts were put in polyethylene carriers, sealed, and stored in a desiccator for low-Z particle EPMA study.
For EDXRF, each representative sample was ground in an agate ball-mill and sieved using a 250-mesh (<63 µm Ø) sieve. The samples were then dried in oven at 105 °C for 24 hr. A pellet of around 2 g weight and 25.4 mm Ø was prepared for each sample in a hydraulic press at 15 ton pressure, thus obtaining an average areal density of ∼395 mg/cm2 for each pellet. Similarly, pellets of National Institute of Standards and Technology (NIST) Standard Reference Material (SRM)-2586, consisting of “soil contaminated with lead from paint”, were also prepared for quality control. The pellets were kept in separate plastic containers and stored in a desiccator. The bulk elemental constituents of the palletized soil samples were analyzed using EDXRF.
For low-Z particle EPMA, each representative soil sample was ground to a fine powder and spread over silver foil (Goodfellow Inc. Llangollen, UK; 99.95% purity, 0.025 mm thickness) by simply contacting particles with the foil prior to low-Z particle EPMA measurements. Approximately 270 individual particles for each soil sample, totaling 1900 particles, were analyzed.
EDXRF
The EDXRF spectrometer consists of a 109Cd radioisotope X-ray source (AEA Technologies; 4 mm Ø and 115 MBq effective activity) and a Si(Li) detector (Canberra; 8 µm Be window, 30 mm2 active area, 5 mm effective thickness, and 145 eV resolution at 5.9 keV). The radioisotope source emits pseudomonochromatic Ag Kα X-rays with an effective energy of 22.61 keV. The source holder with a graded collimator, the sample holding plate, and the graded collimator on the detector side are mounted in a mechanical arrangement with a definite geometry.Citation14 The angles subtended by the incident and emergent radiations with respect to the sample surface were determined experimentally to be nearly 45° each, as described in detail elsewhere.Citation14,Citation26 The detection medium was air with an X-ray detection range of 3–20 keV. All the spectra for the soil samples and SRM-2586 were taken with a preset time of 60,000 sec.
The spectrum deconvolution was done using the AXIL program in the QXAS package.Citation27,Citation28 The backscatter fundamental parameter (BFP) algorithm of the QXAS package was used for quantification of the soil samples.Citation26 For the determination of the absolute mass fractions of the constituent elements, matrix corrections for absorption and enhancement effects involving both the fluorescent elements and the elements with undetected characteristic X-rays below 3 keV, forming the dark matrix, are needed. In the BFP method,Citation23 the ratio of coherent to incoherent scattered radiation intensities are used to find the dark matrix.Citation29,Citation30 The required fluorescent instrumental constants were estimated by irradiating different standard elemental foils,Citation14 pellets of pure compounds, and/or thin films of pure elements on Mylar substrates.Citation11 Scattering constants (coherent and incoherent) were obtained by measurement of the characteristic scattered peaks generated by irradiating standard pellets of the compounds (of mostly low-Z elements with known stoichiometry) that have similar thicknesses to the samples.Citation14 All the calibration measurements were done under identical geometrical conditions as for the samples. The EDXRF spectra (for samples and standards) were deconvoluted after subtracting the instrumental backgrounds. The backgrounds were measured for the blank sample plate in an identical geometry and over an identical measuring time.
For quality control, the NIST SRM-2586 sample pellet was repeatedly analyzed spanning the duration of the entire experiment. The mean values of the elemental concentrations and the relative standard deviation (RSD) values obtained from five repeated measurements are given in the second and third columns of , respectively. The relative deviations (RDs) between the measured and the certified values are less than 10% for the elements, except for Ni (RD = −16.0%), Y (RD = 53.3%), and Nb (RD = 73.3%), whose mass fractions are quoted just as information values without any attributed error. The detection limits in the soil matrix for a spectrum collection time of 60,000 sec and also a more conservative “method detection limit” (MDL), which was estimated as 3 times the RSD, were estimated from the analyzed NIST SRM-2586 sample pellet (). The low precision (RSD ∼36%) and high RD for Ni is due to its high detection limit (DL) (∼39 mg/kg) and relatively low concentration in the SRM (75 mg/kg). The high RDs for Y and Nb, despite their low DL values, are due to their characteristic X-ray energies being near the exciting radiation energy, and may be attributed to systematic errors, which arise from the peak overlaps of Y Kα with Pb Lγ and Rb Kβ, and Nb Kα with Y Kβ. Also their concentrations in the SRM are very low. The unknown soil samples were subsequently analyzed using the same calibrations.
Table 1. EDXRF-quantified concentrations, relative deviations, and detection limits of elements in NIST SRM-2586
Low-Z Particle EPMA
For the characterization of soil samples, a quantitative single-particle analytical technique, named low-Z particle EPMA, was applied to obtain morphological and elemental compositional information on individual soil particles. The measurements were carried out in a JEOL JSM-6390 scanning electron microscope (SEM) equipped with an Oxford Link SATW ultrathin window EDX detector. The resolution of the detector is 133 eV for Mn Kα X-rays. X-ray spectra were recorded under the control of Oxford INCA Energy software. An accelerating voltage of 20 kV and a beam current of 1.0 nA were used for all measurements. To obtain a statistically significant number of counts in the X-ray spectra while limiting the beam damage on the sensitive soil particles, a typical measuring time of 15 sec was used. A more detailed discussion of the measurement conditions is given elsewhere.Citation31 The net X-ray intensities of the chemical elements were obtained by nonlinear, least squares fitting of the collected spectra using the AXIL program.Citation32 The elemental concentrations of the individual particles were determined from their X-ray intensities by the application of a Monte Carlo calculation combined with reverse successive approximations.Citation31,Citation33 The quantification procedure provided results with an accuracy of within 12% relative deviation between the calculated and nominal elemental concentrations for various standard particles.Citation33 The low-Z particle EPMA method can provide quantitative information on the chemical compositions, and particles can be classified based on their chemical species. The analytical procedure for determining the chemical species of individual particles and assigning those particles to specific types is described in more detail elsewhere.Citation34,Citation35
RESULTS AND DISCUSSION
EDXRF for Bulk Elemental Analysis of Soil Samples
The overlaid EDXRF spectra of two MSW-contaminated soil samples, Dh1 (near the core of dumping) and Dh6 (near the roadside), are compared with that of the noncontaminated soil sample (Csl) in . Characteristic X-ray peaks for elements K, Ca, Ti, Cr, Mn, Ni, Fe, Cu, Zn, Rb, Sr, Zr, Nb, Mo, and Y (K X-rays); and Pb (L X-rays) are clearly detected. All the Pb L X-ray lines are visible for sample Dh6, indicating the presence of Pb in high concentration.
Figure 2. EDXRF spectra of two MSW-contaminated soil samples (Dh1 and Dh6) and the noncontaminated countryside soil sample (Csl).
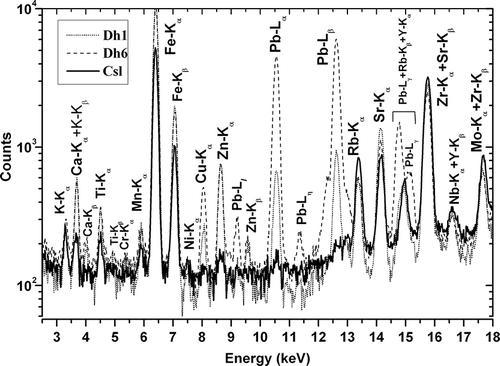
The bulk elemental concentrations of the surface soil samples in the MSW-contaminated site as well as the countryside soil sample are shown in . The average concentrations of the major elements such as K, Ca, and Fe in the MSW-contaminated soil samples (Dh1–Dh6) are 1.6%, 2.7%, and 4.7%, respectively, with a relative standard deviation within 8%. The concentrations of these elements in the countryside soil sample (Csl) are 1.7%, 0.7%, and 2.3%, respectively. As the concentrations of major Ca and Fe elements are always higher in samples Dh1–Dh6 than in sample Csl, those major elements must come from the MSW. Many elements such as K, Ca, Ti, Fe, Ni, Rb, Sr, and Zr show similar concentrations in samples Dh1–Dh6. However, the elemental concentrations of Cr, Mn, and Cu vary among samples Dh1–Dh6. The concentration of Pb in the roadside sample (Dh6) (4636 ± 60 mg/kg) is an order of magnitude higher than the rest of the contaminated site samples (Dh1–Dh5) (average = 484 ± 63 mg/kg).
Table 2. EDXRF quantified elemental concentrations of MSW-contaminated soil samples Dh1–Dh6 and a composite countryside soil sample Csl, and the corresponding enrichment factors of chemical elements in contaminated soil samples with respect to those in the countryside soil sample Csl
All the investigated elements, except K, Ni, Rb, and Zr, clearly show higher concentrations in samples Dh1–Dh6 than in sample Csl. To scale the degree of enhancement of these elements in the MSW-contaminated soil, the ratio of the concentration of an element in each contaminated soil sample (Dh1–Dh6) to that of the same element in the noncontaminated soil sample Csl is represented by R1–R6 in . Some metals such as Cu, Zn, and Pb are found to be enriched by almost an order of magnitude or more in samples Dh1–Dh6 compared to the natural soil Csl. The elevated concentrations of Cu, Zn, and Pb were reported to be common in mixed-MSW composts, recyclable urban garbage, and compost-amended soils around the world.Citation5,Citation17,Citation36 This common feature may be attributed to progressive mineralization and natural composting of the raw MSW constituting the bulk of the soil at Dhapa.Citation5 However, the degree of contamination, the enrichment factors, and the chemical species in MSW-contaminated soils will be different from different localities, societies, ethnic behaviors, climates, and economic statuses. The Pb level in the roadside sample (Dh6) is 2 orders of magnitude higher than that in sample Csl. The Ca and Cr levels in samples Dh1–Dh6 are elevated 4 times compared to those in sample Csl; Ti, Mn, Fe, and Sr are 2–3 times enriched. K, Rb, and Zr are not enriched, suggesting that those elements are mainly of natural soil origin.
The sources of elevated Cr in the MSW-contaminated surface soils (Dh1–Dh6) might be traced back to erstwhile leather processing units that had existed in and around this area. The other sources may be scrap metals, textiles, and fine metal particles, which are major components of MSW. The elevated levels of Cu in samples Dh1–Dh6 may be attributed to various sources such as scrap metals, building materials, leather, fine metal particles, etc. Samples Dh1–Dh6 may be enriched in Zn by wood, scrap metals, rubber, and fine metal particles. The probable sources of Pb are wood, crockery, paint, plastics, leather, and fine particles.Citation2 The levels of Ca in the contaminated soils are high because of the influence of building scraps, crockery, bones, etc., dumped indiscriminately along with garbage. However, because Ca is an essential element, it may be a positive contaminant. The high amounts of Cu and Pb in the roadside sample (Dh6) are most probably due to vehicular emission from the bypass highway, corrosion of construction materials used for building the urban structures on the roadside, and/or emissions from Pb smelting units located nearby. To ascertain the sources, further rigorous investigation coupled with source apportionment is required.
There are several ecological receptors that commonly come into contact with the contaminated soils or ingest biota that live in or on these soils at Dhapa. The primary receptors in direct contact with the soil are the plants growing on this soil, the soil microbes, and the soil invertebrates, such as earthworm and insects. The secondary receptors, which may be in direct and/or indirect contact with the soil, are human beings (farmers, laborers, rag pickers, scavengers, vegetable consumers, etc.), mammals (cattle, domestic animals, etc.), birds, reptiles, and amphibians. In order to qualitatively assess the level of exposure risk for the receptors from the heavy metals observed in the contaminated soil samples, we compared the elemental concentrations in samples Dh1–Dh6 with the Ecological Soil Screening Levels (Eco-SSLs)Citation37 set by the United States Environment Protection Agency (U.S. EPA). From the results shown in , the following observations can be made:
Table 3. The quantified concentrations of heavy metals in samples Dh1–Dh6 and Csl and Ecological Soil Screening levels (Eco-SSLs) set by EPA
Cr: The total Cr concentrations in the MSW-contaminated soil samples are higher than the cumulative Eco-SSL values of Cr(III) and Cr(VI) for the wildlife receptors (Eco-SSL categories c and d). As Cr(VI) species is more toxic than Cr(III), the level of each Cr species needs to be determined in future investigations. Also, the natural-soil Cr content in this region seems to be high for wildlife receptors, as seen from its concentration in Csl. However, no significant uptake of Cr in radish and cauliflower plants was previously reported.Citation12,Citation14 | |||||
Mn: The Mn concentrations in samples Dh1–Dh6 are lower than wildlife Eco-SSL values (categories c and d); however, they may be of concern for plants and soil invertebrates (categories a and b). | |||||
Ni: Concentration levels of Ni in samples Dh1–Dh6 are not dangerously high when compared to the Eco-SSL values for all receptor categories and are comparable to that in sample Csl. However, the uptake of Ni has been observed in both radishes and cauliflowers grown at Dhapa.Citation12,Citation14 | |||||
Cu and Zn: The concentrations of Cu and Zn in samples Dh1–Dh6 are significantly higher (by about an order of magnitude) than the corresponding Eco-SSL values for all four categories of receptors, suggesting that soil remediation would be of primary concern for Cu and Zn. The concentrations of these two elements in sample Csl can be considered within safe limits. | |||||
Pb: For the roadside soil sample Dh6, the Pb concentration is exceptionally high at about 4500 mg/kg and of grave concern for all the four receptor categories. The levels of Pb in samples Dh1–Dh5 are still quite high for plant and wildlife receptors (Eco-SSL categories a, c, and d). Soil invertebrates like earthworms, which are vital for soil evolution, may still be able to survive at these concentrations of Pb (although the concentrations of Cu and Zn may prove otherwise). The Pb concentration in sample Csl is low enough to be considered safe. |
There exist no local or Indian soil screening levels for heavy metals similar to the ones shown above for U.S. EPA. Hence, Eco-SSL values based on regional risk assessment studies such as chemical sequential extraction, plant bioassays, phytoextraction, etc., will be required in the future to implement remedial measures more effectively.Citation5
Low-Z Particle EPMA for the Analysis of Individual Soil Particles
Overall 1900 individual particles from seven soil samples (about 270 particles for each sample) were analyzed using low-Z particle EPMA. Typical secondary electron images (SEIs) of individual soil particles, obtained from MSW-contaminated and countryside soil samples, are shown in , where the chemical species present in each particle are noted. The elements in a particle are judged to be present when the signal-to-noise (S/N) ratios of their characteristic X-ray peaks are larger than 3. In order to demonstrate the classification of particles based on their SEIs and X-ray spectral data, the atomic elemental concentrations and identified chemical species for the particles in the field image of are shown in . For the notation of the chemical species present in the particles, a unique notation is devised; that is, aluminosilicate/C indicates that the aluminosilicate and carbonaceous species are major (greater than 10 atomic%), C/Ca(CO3,NO3)/SiO2 indicates that the particle is composed of an internal mixture of carbonaceous CaCO3, Ca(NO3)2, and SiO2. AlSi[Na,Mg,K] indicates that the particle is composed of aluminosilicate (notated as AlSi), with minor levels of Na, Mg, and K.
Table 4. Elemental concentrations (in atom%) and identified chemical species of particles shown in
Figure 3. Typical SEIs of individual soil particles obtained from (a) a MSW-contaminated soil sample (Dh6) and (b) the countryside soil sample (Csl).
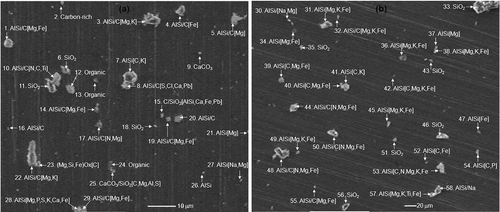
As shown in , all the particles look angular and bright, which is characteristic of mineral soil particles.Citation18,Citation19 In general, natural soil particles result from weathering and eroding of crustal rocks, and the major constituents of soil are similar to those of rock-forming minerals. The most common minerals constituting earth's crust are silicates. Quartz (SiO2) and feldspar are the two most common silicate minerals, and pyroxenes, olivine, mica, and clay minerals are also silicates. However, due to the diversity of the chemical compositions of the silicate minerals, in this study, all the silicate particles except SiO2 are classified as “aluminosilicate-containing particles”. The aluminosilicate-containing particles include aluminosilicate, aluminosilicate/C, and aluminosilicate/misc groups, where the aluminosilicate particles are composed of silicon and aluminum oxides. The aluminosilicate/C particles contain aluminosilicate and carbonaceous species and aluminosilicate/misc is composed of aluminosilicate, with minor elements such as C, Na, Mg, S, Cl, K, Ca, Ti, and/or Fe (see ). A few aluminosilicate/misc particles include transition and heavy metals such as Cr, Mn, Ni, Cu, Zn, and/or Pb as minor elements; for example, particle 15 in . Three particle types such as calcite (CaCO3), dolomite (CaMg(CO3)2), and an internal mixture of CaCO3 with SiO2, Ca3(PO4)2, and/or Fe oxides, for example, particles 9 and 25 in , belong to the “calcium carbonate (CaCO3)-containing particle” group. In many cases, “silicon dioxide (SiO2)-containing particles” include pure SiO2 and are frequently mixed with carbonaceous species, for example, particles 6, 11, and 18 in . In addition, “carbonaceous particles” are significantly observed in MSW-contaminated soil samples. Because hydrogen cannot be detected, the low-Z particle EPMA has a limitation on the specific speciation of organic particles. Thus, the particles are simply classified either as organic or carbon-rich when the sum of the carbon and oxygen contents of the particles is larger than 90% atomic fraction. When the carbon content of the particle is, somewhat arbitrarily, 3 times larger than the oxygen content, the particle is designated as carbon-rich; for example, particle 2 in (see ). Particles that could not be classified into any of the above types were placed in an “other species” group. They include FeOx, FeOx/Ca3(PO4)2, FeOx/C/Mn, FeOx/Zn, (Si,Fe)Ox, (Mg,Si)Ox, (Si,Fe,Ca)Ox, (Mg,Si,Fe)Ox, (Ca,Pb)SO4/C, and MnOx particles.
All the particles analyzed were classified into the above-mentioned categories. The relative abundances of such particle groups encountered in the soil samples vary among samples, as shown in . The most common particle type encountered in the soil samples is “aluminosilicate-containing particles”, encountering number frequencies of 65–79%. The particles classified as aluminosilicate species are mainly composed of Al2O3 and SiO2, with minor levels of the elements Na, Mg, K, Ca, Ti, and/or Fe. The elemental concentration of the minor elements of aluminosilicate-containing particles are in the range 1–9.8 atomic%. As shown in , Na, Mg, K, Ca, Ti, and/or Fe elements are frequently observed in the aluminosilicate-containing particles (average values are greater than 4%), whereas the frequency at which S, Cl, Cr, Mn, Ni, Cu, Zn, and/or Pb are observed in the aluminosilicate-containing particles is low (average values are less than 1%) for all the soil samples. However, the contents of minor elements such as Na, Mg, K, Ca, Ti, and/or Fe in aluminosilicate-containing particles vary among the soil samples (). Among the minor elements, Mg and Fe are the most frequently encountered elements (average values: 66.2% and 60.9%, respectively) in aluminosilicate-containing particles, followed by K, Ca, Na, and Ti (average values: 38.1%, 30.5%, 8.6%, and 4.6%, respectively). Mg, K, and Fe in aluminosilicate-containing particles are more abundant in the countryside soil sample than in Dh1–Dh6 soil samples. Although there is not much difference in the total contents of aluminosilicate-containing particles between the countryside and MSW-contaminated soil samples, the aluminosilicate/misc particles are more abundant in the countryside soil sample than in samples Dh1–Dh6 (average values: 76.7% and 53.6%, respectively) and the aluminosilicate/C particles are present only in the MSW-contaminated soil samples (average value: 11.6%), indicating that this carbonaceous species came from MSW ( and ).
Table 5. Encountering frequencies of minor chemical elements in aluminosilicate-containing particles
Table 6. Relative abundances of aluminosilicate-containing particles encountered in the soil samples
SiO2 particles, the major component of sand, are the second most frequently encountered particles in the soil samples, as shown in , with relative abundances of 9.4–22.6%. The relative frequency of encountering SiO2 in sample Csl is higher (22.6%) than in samples Dh1–Dh6 (9.4–15.3%). Soil particles encountered in MSW-contaminated and countryside soil samples are clearly different; that is, the countryside soil sample contains only aluminosilicate- and SiO2-containing particles, whereas the MSW-contaminated samples are not limited to these two particle types. The MSW-contaminated and countryside soil samples contain mainly mineral particles. However, those mineral particles for sample Csl are solely of geogenic origin, whereas those for samples Dh1–Dh6 seem to be evolving from a mixture of raw dumped MSW, urban dust (sources: street sweeping, vehicular movement, etc.),Citation10 and various other contributing factors such as wind, precipitation, weather patterns, farming, and water logging, resulting in their diverse chemical compositions and the observation of abundant carbonaceous species. Significant amounts of CaCO3-containing and carbonaceous particles (average values: 6.3% and 5.9%, respectively) were encountered for samples Dh1–Dh6, whereas they were absent in sample Csl. Those CaCO3-containing and carbonaceous particles seem to be from ash, limestone, and chalk dust in dumped MSW. Also, the particles in the “other species” group were frequently encountered in samples Dh1–Dh6 with relatively low abundances (average value: 2.8%), whereas they were absent in sample Csl.
Carbon (organic matter) and phosphorus elements are important for the fertility of soil and exposure to heavy metals in the food chain is also of concern, and thus these elements in the soil samples were investigated again; that is, in the classification process, the particles having detectable but very minor contents of chemical elements such as C, P, and heavy metals (see ) could not be classified into the particle types already listed. Therefore, particles with detectable C, P, and heavy metals among all 1900 particles were counted. Particles containing carbon and phosphorous were observed more abundantly in MSW-contaminated soil samples than in the countryside soil sample (average values: 49.5% vs. 29.5%; 15.4% vs. 4.8%, respectively) (see ), suggesting that MSW-contaminated soils are more fertile. A previous work also reported that the chemical properties of MSW landfill soil are good for vegetation, compared to background soil with poor soil fertility.Citation38 However, particles containing potentially toxic heavy metals such as Cr, Ni, Mn, Cu, Zn, and/or Pb were significantly observed in samples Dh1–Dh6 (0.7–10.1%), whereas such particles were absent in sample Csl (). The metal-containing particles exist either as major particle types, that is, metal oxide/sulfate, or as minor elements. The elemental concentrations of metals such as Ti, Cr, Fe, Mn, Ni, Cu, Zn, and/or Pb vary from 0.2 to 29.9 atomic% among particles. It is worth noting that Pb was the most frequently encountered metal in sample Dh6, agreeing well with the observations from EDXRF.
Table 7. Encountering frequencies (in %) of C- and P-containing particles in soil samples
Table 8. Encountering frequencies (in %) of metal-containing particles in the soil samples.Footnote a
SUMMARY
In this work, MSW-contaminated and apparently noncontaminated countryside soil samples were analyzed using two complementary nondestructive techniques: EDXRF and low-Z particle EPMA. EDXRF analysis estimates the bulk-soil elemental concentrations, and low-Z particle EPMA elucidates the major and minor inorganic chemical species present in individual particles.
EDXRF results show that the concentrations of the major bulk constituent elements K, Ca, and Fe are approximately similar along the entire 2000 m stretch of MSW-contaminated soil (Dh1–Dh6). K, Rb, and Zr have not been enhanced in comparison to the natural countryside soil (Csl), indicating their geogenic origin. There are variations in the minor and trace element concentrations, viz. Cr, Cu, and Pb, in the MSW-contaminated soils. The bulk concentrations of K, Rb, and Zr remain almost unchanged in all the soil samples. The Dhapa soil is found to be polluted with heavy metals such as Cu, Zn, and Pb (highly elevated) and Ti, Cr, Mn, Fe, Ni, and Sr (moderately elevated), compared to the natural countryside soil.
The low-Z particle EPMA results show that the aluminosilicate-containing particles are the most abundant, followed by SiO2, CaCO3-containing, and carbonaceous particles in samples Dh1–Dh6, whereas in sample Csl only aluminosilicate-containing and SiO2 particles are observed. Both the MSW-contaminated and countryside soil samples mainly contain mineral particles. However, those mineral particles for sample Csl are solely of geogenic origin, whereas those for samples Dh1–Dh6 seem to be evolving from a mixture of raw dumped MSW, urban dust (from street sweeping, vehicular movement, etc.),Citation10 and various other contributing factors such as wind, precipitation, weather patterns, farming, and water logging, resulting in their diverse chemical compositions and observation of abundant carbonaceous species. Particles containing carbon and phosphorous were observed more abundantly in the Dh1–Dh6 soil samples than in the Csl soil sample, suggesting that MSW-contaminated soils are more fertile. However, particles containing potentially toxic heavy metals such as Ti, Cr, Mn, Ni, Cu, Zn, and/or Pb were significantly observed in samples Dh1–Dh6.
An exceptionally high amount of Pb is observed in the roadside sample, Dh6, when analyzed with either analytical approach to investigation. The encountering frequency of Fe for the countryside soil particles is higher than that for the MSW-contaminated soil particles (by low-Z particle EPMA), whereas the bulk concentrations of Fe in the MSW-contaminated soils are twice as high as that in sample Csl (by EDXRF), suggesting that Fe is more evenly distributed in the natural soil particles. Elevated bulk-soil concentration levels of heavy metals (by EDXRF) are complemented by the observation of higher encountering frequencies of metals for MSW-contaminated soils (by low-Z particle EPMA). This suggests that the topsoil, which has been evolving mainly from dumped MSW, is highly contaminated with heavy metals. Thus, the existing practice of agriculture on these soils may carry the risk of contaminating the food chain.Citation36,Citation39 Investigations regarding the elemental phytoavailability and uptake pattern by different vegetable species grown on this site have already been performed.Citation12–14 A full understanding of the sources of heavy metals in MSW and the evolution process of this so-called anthropogenic soil will require a further detailed time scale study.Citation2,Citation5 Soil remedial measures for removal or immobilization of the heavy metals have to be considered.
ACKNOWLEDGMENTS
The authors are indebted to the contributions, constant encouragement, and support from Prof. Jyotsnamoyee Chatterjee, presently with Spark Steel and the Economy Research Center, Kolkata, India. The active help of Mr. S.C. Sarkar and other staff at the WBPCB, Kolkata, India, during the sample collection process at the dump site is gratefully acknowledged. The authors are thankful to Prof. R. Ranganathan of the ECMP division of the Saha Institute of Nuclear Physics, Kolkata, India, for kindly extending the ball-mill grinding facility in his laboratory for our soil sample preparation. The authors are also thankful to Dr. A.A. Markowicz, Dr. D. Wegrzynek, Dr. R. Padilla Alvarez, and Mr. E. Chinea Cano of IAEA XRF Laboratories, Siebersdorf, Austria, for their generous suggestions and support in the process of handling the QXAS package for EDXRF. The EDXRF laboratory work was supported by the Department of Atomic Energy, Government of India. This work was supported by Basic Science Research Program through the National Research Foundation of Korea (NRF) funded by the Ministry of Education, Science and Technology (2010-0018881).
REFERENCES
- Burnley , S. , Cooke , D. and Gladding , T. 2007 . Environmental Monitoring Modeling and Control: Block 4—Solid Wastes Management , 2nd , London : The Open University .
- Prudent , P. , Domeizel , M. and Massiani , C. 1996 . Chemical Sequential Extraction as Decision-Making Tool: Application to Municipal Solid Waste and its Individual Constituents . Sci. Total Environ. , 178 : 55 – 61 .
- Richard , T.L. and Woodbury , P.B. 1992 . The Impact of Separation on Heavy Metal Contaminants in Municipal Solid Wastes Composts . Biomass Bioenerg. , 3–4 : 195 – 211 .
- Gillett , J.W. 1992 . Issues in Risk Assessment of Compost from Municipal Solid Waste: Occupational Health and Safety, Public Health, and Environmental Concerns . Biomass Bioenerg. , 3–4 : 145 – 162 .
- Farrell , M. and Jones , D.L. 2009 . Heavy Metal Contamination of a Mixed Waste Compost: Metal Speciation and Fate . Bioresource Technol. , 100 : 4423 – 4432 . doi: 10.1016/j.biortech.2009.04.023
- Jain , A. , Kaur , H. and Khanna , S. 2005 . Computer Model for Municipal Solid Waste Treatment in Developing Countries . Environ. Sci. Technol. , 39 : 3732 – 3735 .
- Zhu , D. , Asnani , P.U. , Zurbrügg , C. , Anapolsky , S. and Mani , S. 2007 . Improving Municipal Solid Waste Management in India: A Sourcebook for Policy Makers and Practitioners , Washington , DC : World Bank .
- Sharholy , M. , Ahmad , K. , Mahmood , G. and Trivedi , R.C. 2008 . Municipal Solid Waste Management in Indian Cities—A Review . Waste Manage. , 28 : 459 – 467 .
- Chattopadhyay , S. , Dutta , A. and Ray , S. 2009 . Municipal Solid Waste Management in Kolkata, India—A Review . Waste Manage. , 29 : 1449 – 1458 .
- Hazra , T. and Goel , S. 2009 . Solid Waste Management in Kolkata, India: Practices and Challenges . Waste Manage. , 29 : 470 – 478 .
- Gupta , D , Chatterjee , J.M. , Ghosh , R. , Mitra , A.K. , Roy , S. and Sarkar . 2007 . M. EDXRF Analysis of Municipal Solid Waste Using 109Cd Source . Appl. Radiat. Isot. , 65 : 512 – 516 .
- Gupta , D , Chatterjee , J.M. , Ghosh , R. , Mitra , A.K. , Roy , S. and Sarkar , M. A . 2007 . Study of Elemental Uptake in Radish Grown Near a Municipal Waste Dumping Site by EDXRF Method . J. Radioanal. Nucl. Chem. , 274 : 389 – 395 .
- Raychaudhuri , S. , Salodkar , S. , Sudarshan , M. and Thakur , A.R. 2007 . Integrated Resource Recovery at East Calcutta Wetland: How Safe Is These? . Am. J. Agric. Biol. Sci. , 2 : 75 – 80 .
- Gupta , D , Chatterjee , J.M. , Ghosh , R. , Mitra , A.K. , Roy , S. and Sarkar , M. 2010 . Radioisotope Induced EDXRF Investigation of Elemental Uptake in Cauliflower Grown at MSW-Contaminated Site . X-ray Spectrom. , 39 : 364 – 371 .
- Tamhane , R.V. , Motiramani , D.P. , Bali , Y.P. and Donahue , R.L. 1964 . Soils: Their Chemistry and Fertility in Tropical Asia , New Delhi : Prentice-Hall of India .
- Goldstein , S.J. , Slemmons , A.K. and Canavan , H.E. 1996 . Energy Dispersive X-ray Fluorescence Methods for Environmental Characterization of Soils . Environ. Sci. Technol. , 30 : 2318 – 2321 .
- Anjos , M.J. , Lopes , R.T. , Jesus , E.F.O. , Assis , J.T. , Cesareo , R. , Barroso , R.C. and Barradas , C.A.A. 2002 . Elemental Concentration Analysis in Soil Contaminated with Recyclable Urban Garbage by Tube Excited Energy-Dispersive X-ray Fluorescence . Radiat. Phys. Chem. , 65 : 495 – 500 .
- Kim , H. and Ro , C.-U. 2010 . Characterization of Individual Atmospheric Aerosols Using Quantitative Energy Dispersive–Electron Probe X-ray Microanalysis: A Review . Asian J. Atmos. Environ. , 4 : 115 – 140 .
- Kim , H. , Hwang , H. and Ro , C.-U. 2006 . Single-Particle Characterization of Soil Samples Collected at Various Arid Areas of China, Using Low-Z Particle Electron Probe X-ray Microanalysis . Spectrochim. Acta B , 61 : 393 – 399 .
- Kang , S. , Hwang , H. , Kang , S. , Park , Y. , Kim , H. and Ro , C.-U. 2009 . Quantitative ED-EPMA Combined with Morphological Information for the Characterization of Individual Aerosol Particles Collected in Incheon, Korea . Atmos. Environ. , 43 : 3445 – 3453 .
- Maskey , S. , Kang , T. , Jung , H. and Ro , C.-U. 2011 . Single-Particle Characterization of Indoor Aerosol Particles Collected at an Underground Shopping Area in Seoul, Korea . Indoor air , 21 : 12 – 24 .
- Geng , H. , Ryu , J. , Jung , H. , Chung , H. , Ahn , K.-H. and Ro , C.-U. 2010 . Single-Particle Characterization of Summertime Arctic Aerosols Collected at Ny-Ålesund, Svalbard . Environ. Sci. Technol. , 44 : 2348 – 2353 .
- Worobiec , A.D.M. , Dekov , V.M. , Laane , R.W.P.M. and Van Grieken , R.E. 2009 . EPXMA Survey of Shelf Sediments (Southern Bight, North Sea): A Glance beyond the XRD-Invisible . Microchem. J. , 91 : 21 – 31 .
- Osan , J. , Torok , S. , Alfoldy , B. , Alsecz , A. , Falkenberg , G. , Bake , S.Y. and Van Grieken , R.E. 2007 . Comparison of Sedimentary Pollution in the Rivers of the Hungarian Upper Tisza Region Using Non-destructive Analytical Techniques . Spectrochim. Acta B , 62 : 123 – 136 .
- Osan , J. , Kurunczi , S. , Torok , S. and Van Grieken , R.E. 2002 . X-ray Analysis of River Sediment of the Tisza (Hungary): Identification of Particles from a Mine Pollution Event . Spectrochim. Acta B , 57 : 413 – 422 .
- Wegrzynek , D. , Markowicz , A.A. and Chinea-Cano , E. 2003 . Application of the Backscatter Fundamental Parameter Method for In Situ Element Determination Using Portable Energy-Dispersive X-ray Fluorescence Spectrometer . X-Ray Spectrom. , 32 : 119 – 128 .
- International Atomic Energy Association (IAEA) . 2005 . Quantitative X-Ray Analysis System, IAEA Manual, Doc. Ver. 2.0 , IAEA : Vienna .
- Van Espen , P. , Nullens , H. and Adams , F. 1977 . A Computer Analysis of X-ray Fluorescence Spectra . Nucl. Instrum. Methods , 142 : 243 – 250 .
- Nielsen , K.K. 1977 . Matrix Corrections for Energy Dispersive X-ray Fluorescence Analysis of Environmental Samples with Coherent/Incoherent Scattered X-rays . Anal. Chem. , 49 : 641 – 648 .
- Van Espen , P. , Van't dack , L. , Adams , F. and Van Grieken , R. 1979 . Effective Sample Weight from Scatter Peaks in Energy Dispersive X-ray Fluorescence . Anal. Chem. , 51 : 961 – 967 .
- Ro , C.-U. , Osán , J. and Van Grieken , R. 1999 . Determination of Low-Z Elements in Individual Environmental Particles Using Windowless EPMA . Anal. Chem. , 71 : 1521 – 1528 .
- Veckemans , B. , Janssens , K. , Vincze , L. , Adams , F. and Van Espen , P. 1994 . Analysis of X-ray Spectra by Iterative Least Squares (AXIL): New Developments . X-ray Spectrom. , 23 : 278 – 285 .
- Ro , C.-U. , Osán , J. , Szalóki , I. , de Hoog , J. , Worobiec , A. and Van Grieken , R. 2003 . A Monte Carlo Program for Quantitative Electron-Induced X-ray Analysis of Individual Particles . Anal. Chem. , 75 : 851 – 859 .
- Ro , C.-U. , Osán , J. , Szalóki , I. , Oh , K.-Y. , Kim , H. and Van Grieken , R. 2000 . Determination of Chemical Species in Individual Aerosol Particles Using Ultrathin Window EPMA . Environ. Sci. Technol. , 34 : 3023 – 3030 .
- Ro , C.-U. , Kim , H. and Van Grieken , R. 2004 . An Expert System for Chemical Speciation of Individual Particles Using Low-Z Particle Electron Probe Microanalysis Data . Anal. Chem. , 76 : 1322 – 1327 .
- Woodbury , P.B. 1992 . Trace Elements in Municipal Solid Wastes Composts: A Review of Potential Detrimental Effects on Plants, Soil Biota, and Water Quality . Biomass Bioenerg. , 3–4 : 239 – 259 .
- USEPA. Ecological Soil Screening Levels http://www.epa.gov/ecotox/ecossl (http://www.epa.gov/ecotox/ecossl)
- Prabpai , S. , Charerntanyarak , L. , Siri , B and Moore , M.R. 2007 . Agronomic Properties and Heavy Metals Content in Soil Reclaimed from Municipal Solid Waste Land-Fill Development of a Knowledge-Based System for Foundry Waste Recycling . J. Solid Waste Technol. Manage. , 33 : 92 – 97 . doi:
- SamsØe-Petersen , L. , Larsen , E.H. , Larsen , P.B. and Bruun , P. 2002 . Uptake of Trace Elements and PAHs by Fruit and Vegetables from Contaminated Soils . Environ. Sci. Technol. , 36 : 3057 – 3063 .