ABSTRACT
To explore environmentally benign solvents for the absorption of NO and NO2, a series of caprolactam tetrabutyl ammonium halide ionic liquids were synthesized. The solubility of NO and NO2 was measured at temperatures ranging from 298.2 to 363.2 K and atmospheric pressure, and the following trend in the solubility of NO and NO2 in ionic liquids with various halide anions was observed, respectively: F > Br > Cl and Br > Cl > F. Moreover, as the temperature increased from 308.15 to 363.15 K and the mole ratio of caprolactam increased from 2:1 to 6:1, the solubility of NO increased. Alternatively, the solubility of NO2 decreased as the temperature increased from 298.15 to 363.15 K, and the mole ratio of caprolactam increased from 2:1 to 6:1. The absorption and desorption of NO and NO2 was practically reversible in the ionic liquids, which was characterized by nuclear magnetic resonance. The method, which is at least partially reversible, offers interesting possibilities for the removal of NO and NO2.
Basic ionic liquids with amino groups were synthesized and used to capture CO2, SO2, and H2S, and to promote hydrogenation of CO2. In this paper, the authors used caprolactam tetrabutyl ammonium halide ionic liquid (IL) as absorbing medium in which NOx could be absorbed. NOx desorbed from the absorbent could be efficiently reduced by right catalysts at high temperature. The absorbed NO and NO2 gas could be desorbed at higher temperature, allowing the ionic liquids to be reused several times without loss of capability. It was believed that caprolactam tetrabutyl ammonium bromide (CPL-TBAB) ILs may be useful for NOx removal reagent for pollution control.
INTRODUCTION
The major source of NOx production from nitrogen-bearing fuels, such as certain coals and oil, is the conversion of fuel bound nitrogen to NOx during combustion. During combustion, the nitrogen bound in the fuel is released as a free radical and ultimately forms free N2, or NO. Fuel NOx can contribute as much as 50% of total emissions when combusting oil and as much as 80% when combusting coal. NOx reacts to form smog and acid rain. Moreover, NOx plays a critical role in the formation of tropospheric ozone. When NOx and volatile organic compounds (VOCs) react in the presence of sunlight, photochemical smog is formed, which is a significant form of air pollution, especially in the summertime. Children, people with lung diseases such as asthma, and people who work or exercise outdoors are particularly susceptible to the adverse effects of smog, including damage to lung tissue and a reduction in lung function. Thus, the reduction of NOx emissions is important. Different reducing agents such as H2, CO, C3H6, and C3H8 have been studied, and the results suggest that hydrogen is the most effective reductant in lean NOx traps.Citation1 Citation Citation Citation Citation5 Many NOx aftertreatment technologiesCitation6 Citation Citation Citation Citation10 have been extensively investigated in the scientific community, including NOx decomposition catalysts, lean NOx catalysts that use hydrocarbons as the reductant, lean NOx traps, and selective catalytic reduction (SCR) catalysts that use ammonia or urea as the reductant. Lean NOx traps normally function by storing NOx under lean conditions and reduce stored NOx to N2 during periodic rich excursions.Citation11
Ionic liquids (ILs) are low-melting salts with extremely low vapor pressures, high thermal and chemical stability, and tuneable solvent power for many organic and inorganic compounds. Thus, ionic liquids can be used as environmentally benign solvents for a number of applications, including gas solubility and separation, cellulose processing, catalysis, extraction, and high-temperature pyrochemical processing.Citation12 Citation13 For example, acidic ILs are efficient catalysts for many acid-catalyzed organic reactions.Citation14 Basic ILs with amino groups have been synthesised and used to capture CO2, SO2, and H2SCitation15 Citation16 and to promote the hydrogenation of CO2.Citation17 ILs can be potentially used as liquid absorbents for gases and as solvents for gas separations. Currently, the use of task specific ionic liquids for conventional alkanolamine solutions in the removal of acidic gases (CO2 and SO2) in gas sweetening processes is an active area of research.Citation18 Our team demonstrated that caprolactam (CPL) tetrabutyl ammonium bromide (TBAB) ionic liquids have a high affinity for SO2 Citation19 and H2SCitation20 and a low affinity for diatomic gases such as N2 and O2.
Thus, a better understanding of the solubility of NO and NO2 in ILs is necessary. ILs based on different mole ratios of caprolactam and tetrabutyl ammonium halides (TBAX), including tetrabutyl ammonium fluoride (TBAF), tetrabutyl ammonium chloride (TBAC), and tetrabutyl ammonium bromide (TBAB), were prepared, and the solubilities of NO and NO2 were determined. The effects of different halides and mole ratios of CPL and TBAB on their capacity for NO and NO2 absorption were systematically investigated. The recovery of NO and NO2 and the recycling of ILs were also conducted by increasing the temperature and decreasing the pressure.
EXPERIMENTAL
Materials
White crystalline caprolactam powder (CAS No. 105-60-2, C6H11NO) with a purity of >99% was obtained from Shijiazhuang Refinery, Jiangsu, China. Tetrabutyl ammonium halides (C16H36NBr, C16H36NCl, and C16H36NF) with a purity of >99.5% were purchased from Jintan Huadong Chemical Research Institute, China. The amount of water in the ILs was determined using the Karl-Fischer technique.
Absorption Measurements
The ionic liquids were dried under vacuum for at least 24 hr at 323.15 K prior to use, and the water content was less than 100 ppm. NO and NO2 with a purity of 99.9% were supplied by Beijing Analytical Instrument Factory (Beijing, China). The NO and NO2 absorption apparatus was prepared according to procedures reported elsewhere.Citation21 NO or NO2 gas was bubbled at a flow rate of 10 mL·min−1 through predetermined amounts of IL (about 5 g), which was loaded into a glass vessel. After 2 hr, the weight of the vessel and NO or NO2 did not change, and equilibrium was achieved. NO and NO2 removed from the vessel were treated with sodium hydroxide. The valves were closed, and the glass vessel was weighed using a balance with an accuracy of ±0.0001 g to determine the mass of absorbed NO and NO2. Lastly, the solubility of NO and NO2 in the ILs was calculated.
Recycling of CPL-TBAX ILs
To desorb NO or NO2, the glass tube was partly immersed in an oil bath with a temperature controller and a vacuum indicator. During desorption, the weight of IL was determined periodically at different temperatures. When NO or NO2 gas was no longer released at the set temperature, the IL was weighed. The results indicated that the solubility of NO or NO2 in IL was almost zero at 373.2 K and 10.1 kPa. However, the absorption and desorption times were dependent on the exposed surface area of the IL; thus, considerable improvements could be achieved. Consecutive absorption (308.2 K, 101.3 kPa) and desorption (383.2 K, 10.1 kPa vacuum) of NO or NO2 gas in recycled IL were measured as a function of the absorption time. The standard deviation of the ratios was equal to ±0.03.
RESULTS AND DISCUSSION
Effect of Halide on the Solubility of NO and NO2 in CPL-TBAX ILs
The solubilities of NO and NO2 in different CPL-TBAX ILs (2:1, mole ratio) at temperatures ranging from 313.15 to 343.15 K were determined at 101.3 kPa, respectively ( and ). The solubility of NO and NO2 was the highest in CPL-TBAF IL (2:1, mole ratio) and CPL-TBAB IL (2:1, mole ratio), respectively. The mole fraction solubility of NO2 was 0.809 at 298.15 K and 0.676 at 333.15 K, which was higher than that of NO2 in 1-ethyl-3-methylimidazolium bis(trifluoromethylsulfonyl) imide (0.01399 at 298.15 K and ).Citation22 The solubility of NO and NO2 in ionic liquids with various halide anions displayed the following trend, respectively: F > Br > Cl and Br > Cl > F. Because fluorine and chlorine are more toxic than bromine, CPL-TBAB IL was selected as the absorbent.
Effect of Temperature on the Solubility of NO and NO2 in CPL-TBAB ILs
CPL-TBAB IL was initially colorless. After absorbing NO or NO2, the color of CPL-TBAB IL changed to pale yellow () or brown (),respectively.
Figure 3. The color change of caprolactam tetrabutyl ammonium bromide ionic liquids with different concentrations of NOx. (a) Caprolactam tetrabutyl ammonium bromide ionic liquid; (b) after bubbling NO for 20 min; (c) after bubbling NO2 for 20 min.
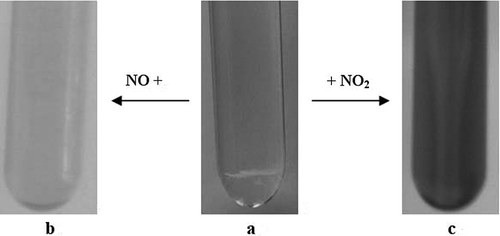
The solubilities of NO in different mole ratios of CPL-TBAB ILs were determined at temperatures ranging from 308.15 to 363.15 K and 101.3 kPa (). The results indicated that the solubility of NO in the ILs increased as the temperature increased from 308.15 to 363.15 K. The solubility of NO in 4:1 and 6:1 CPL-TBAB IL (mole ratio) increased sharply with an increase in temperature. The highest mole fraction solubility of NO was observed in 4:1 CPL-TBAB IL (mole ratio) (0.170 at 353.15 K).
Figure 4. The mole fraction solubility (x) of NO as a function of temperature at different mole ratios of CPL and TBAB.
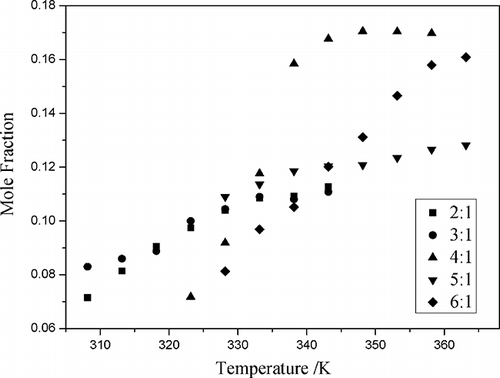
The solubility of NO2 in CPL-TBAB ILs at mole ratios of 2:1 to 6:1 and temperatures of 298.15 to 363.15 K was determined at 101.3 kPa (). The results revealed that the solubility of NO2 decreased sharply as the temperature increased. The mole fraction solubility of NO2 was 0.809 at 298.15 K and 0.676 at 333.15 K in 2:1 CPL-TBAB IL (mole ratio).
Figure 5. The mole fraction solubility (x) of NO2 as a function of temperature at different mole ratios of CPL and TBAB.
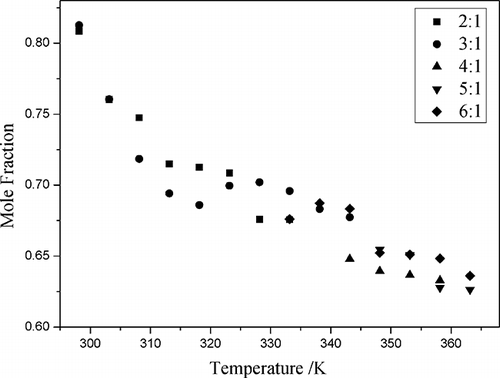
To examine how the solubility of the NOx and how the stability of the ionic liquids will be affected by the other species present in exhaust gas, the solubility of carbon dioxide, oxygen, and nitrogen were measured and in the following order: NO2 > CO2 > NO ≈ O2 ≈ N2 (). Unburned hydrocarbons were almost not dissolved in the ionic liquids. With chemical changes, the gas solubility in ionic liquids is related to its degree of ionization, which could be easily explained by the principle that the similar substance is more likely to be dissolved by each other. Water and IL were almost miscible. But when the mixture of NO2 and water vapor was added into the IL, the IL was broken and could not be reused. So, the exhaust gas should be dried before treating by ionic liquids.
Reusability of CPL-TBAB ILs
The absorption and desorption of NOx in 2:1 and 3:1 CPL-TBAB IL were measured. Absorption and desorption were dependent on the exposed surface area of the ILs, and four consecutive absorption cycles are shown in As shown in the figure, the adsorption time of the IL had a minor effect on the solubility of NO, and NO desorption rates greater than 90% were obtained. The desorption of NO2 was lower than that of NO under the same conditions. However, the ILs could be reused many times without any loss of absorption capability.
Characterization of CPL-TBAB IL
The chemical shifts of CPL-TBAB IL and CPL-TBAB IL saturated with NO and NO2 are shown in the 1H nuclear magnetic resonance (NMR) spectra in As shown in the figure, the effect of hydrogen bonding was insufficient and could not be detected in the 1H NMR spectra. Based on these results, NOx must be physically absorbed in the IL, and chemical bonding between IL and NOx does not occur.
CONCLUSIONS
In summary, 2:1 CPL-TBAX (mole ratio) ILs could physically absorb large amounts of NO and NO2 at 298.2 to 363.2 K. Moreover, 2:1 CPL-TBAB (mole ratio) IL could absorb large amounts of NO (0.170, mole fraction solubility) and NO2 (0.809, mole fraction solubility) at 298.15 K. To have a maximum NO and NO2 absorption capacity, the optimum temperature and mole ratio of caprolactam and tetrabutyl ammonium bromide were room temperature and 2:1 (mole ratio), respectively. NO and NO2 absorbed in the IL remained in the molecular state, and chemical reactions during absorption were not observed. Absorbed NO and NO2 gases could be desorbed from the ILs at higher temperatures, allowing the ILs to be reused several times without observing a loss of capability. NOx could be reduced by the appropriate catalysts at higher temperatures. Thus, CPL-TBAB ILs may be useful as a NOx removal reagent for pollution control. The aforementioned approach will be studied in future work.
ACKNOWLEDGMENTS
This research is supported by the Natural Science Foundation of China (no. 201106033), the Natural Science Foundation of Hebei (no. B2011208017 and 10276736), and Hebei Education Department (no. 2010237).
REFERENCES
- Park , C. , Kim , C. , Kim , K. , Lee , D. , Song , Y. and Moriyoshi , Y. 2010 . The Influence of Hydrogen-Enriched Gas on the Performance of Lean NOx Trap Catalyst for a Light-Duty Diesel Engine . Int. J. Hydrogen Energy , 35 : 1789 – 1796 .
- Liu , Z. and Anderson , A. 2004 . Influence of Reductant on the Regeneration of SO2-Poisoned Pt/Ba/Al2O3 NOx Storage and Reduction Catalyst . J. Catal. , 228 : 243 – 253 .
- Jozsa , P. , Jobson , E. and Larsson , M. 2004 . Reduction of NOx Stored at Low Temperatures on a NOx Adsorbing Catalyst . Top. Catal. , 30 : 177 – 180 .
- James , D. , Fourre , E. and Ishii , M. 2003 . Catalytic Decomposition/Regeneration of Pt/Ba(NO3)2 Catalysts: NOx Storage and Reduction . Appl. Catal. B Environ. , 45 : 147 – 159 .
- Abdulhamid , H. , Fridell , E. and Skoglundh , M. 2004 . Influence of the Type of Reducing Agent; H2, CO, C3H6 and C3H8; on the Reduction of Stored NOx in a Pt; BaO; Al2O3 Model Catalyst . Top. Catal. , 30 : 161 – 168 .
- Chen , M. , Du , C.Y. , Zhang , J. , Wang , P.P. and Zhu , T. 2011 . Effect, Mechanism and Recovery Of Nitrogen Oxides Poisoning on Oxygen Reduction Reaction at Pt/C Catalysts . J. Power Sources , 196 : 620 – 626 .
- Saravanan , N. and Nagarajan , G. 2009 . An Insight on Hydrogen Fuel Injection Techniques with SCR System for NOx reduction in a Hydrogen-Diesel Dual Fuel Engine . Int. J. Hydrogen Energy , 34 : 9019 – 9132 .
- Rodríguez-Fernández , J. , Tsolakis , A. , Cracknell , R. , Clark , R. and Combining GTL Fuel . 2009 . Reformed EGR and HC-SCR After Treatment System To Reduce Diesel NOx Emissions—A Statistical Approach . Int. J. Hydrogen Energy , 34 : 2789 – 2799 .
- Toops , J. , Bunting , G. , Nguyen , K. and Gopinath , A. 2007 . Effect of Engine Based Thermal Aging on Surface Morphology and Performance of Lean NOx Traps . Catal. Today , 123 : 285 – 292 .
- Forzatti , P. and Lietti , L. 2010 . The Reduction of NOx Stored on LNT and Combined LNT-SCR Systems . Catal. Today , 155 : 131 – 139 .
- Takahashi , N. , Shinjoh , H. and Iijima , T. 1996 . The New Concept 3-Way Catalyst for Automotive Lean-Burn Engine: NOx Storage and Reduction Catalyst . Catal. Today , 27 : 63 – 69 .
- Liu , F.S. , Li , Z. , Yu , S.T. , Cui , X. and Ge , X.P. 2010 . Environmentally Benign Methanolysis of Polycarbonate to Recover Bisphenol A and Dimethyl Carbonate in Ionic Liquids . J. Hazard. Mater. , 174 : 872 – 875 .
- Anderson , J.L. , Dixon , J.K. and Brennecke , J.F. 2007 . Solubility of CO2, CH4, C2H6, C2H4, O2, and N2 in 1-Hexyl-3-Methylpyridinium Bis(Trifluoromethylsulfonyl)Imide: Comparison to Other Ionic Liquids . Acc. Chem. Res. , 40 : 1208 – 1216 .
- Cole , A.C. , Jensen , J.L.I. , Ntai , K.L. , Tran , T. , Weaver , K.J. , Forbes , D.C. and Davis , J.H. Jr . 2002 . Novel Brönsted Acidic Ionic Liquids and Their Use as Dual Solvent-Catalysts . J. Am. Chem. Soc. , 124 : 5962 – 5963 .
- Bates , E.D. , Mayton , R.D. , Ntai , I. and Davis , J.H. Jr . 2002 . CO2 Capture by a Task-Specific Ionic Liquid . J. Am. Chem. Soc. , 124 : 926 – 927 .
- Li , W.J. , Zhang , Z.F. , Han , B.X. , Hu , S.Q. , Song , J.L. , Xie , Y. and Zhou , X.S. 2008 . Switching the Basicity of Ionic Liquids by CO2 . Green Chem , 10 : 1142 – 1145 .
- Zhang , Z.F. , Xie , Y. , Li , W.J. , Hu , S.Q. , Song , J.L. , Jiang , T. and Han , B.X. 2008 . Hydrogenation of Carbon Dioxide Is Promoted by a Task-Specific Ionic Liquid . Angew. Chem. Int. Ed. , 47 : 1127 – 1129 .
- Crowhurst , L. , Lancaster , N.L. , Arlandis , J.M.P. and Welton , T. 2004 . Manipulating Solute Nucleophilicity with Room Temperature Ionic Liquids . J. Am. Chem. Soc. , 126 : 11549 – 11555 .
- Guo , B. , Duan , E.H. , Ren , A.L. , Wang , Y. and Liu , H.Y. 2010 . Solubility of SO2 in Caprolactam Tetrabutyl Ammonium Bromide Ionic Liquids . J. Chem. Eng. Data , 55 : 1398 – 1401 .
- Guo , B. , Duan , E.H. , Zhong , Y.F. , Gao , L. , Zhang , X.S. and Zhao , D.S. 2011 . Absorption and Oxidation of H2S in Caprolactam Tetrabutyl Ammonium Bromide Ionic Liquid . Energy Fuel , 25 : 159 – 161 .
- Yuan , X.L. , Zhang , S.J. and Lu , X.M. 2007 . Hydroxyl Ammonium Ionic Liquids: Synthesis, Properties, and Solubility of SO2 . J. Chem. Eng. Data , 52 : 596 – 599 .
- Broder , T.L. , Silvester , D.S. , Aldous , L. , Hardacre , C. and Compton , R.G. 2007 . Electrochemical Oxidation of Nitrite and the Oxidation and Reduction of NO2 in the Room Temperature Ionic Liquid [C2mim][NTf2] . J. Phys. Chem. B , 111 : 7778 – 7785 .