ABSTRACT
Microbial particles can readily be released into the air from different types of man-made sources such as waste operations. Microbiological emissions from different biological sources and their dispersion may be an issue of concern for area planning and for nearby residents. This study was designed to determine the concentrations and diversity of microbiological emissions from four different man-made source environments: waste center with composting windrows, sewage treatment plant, farming environment, and cattle manure spreading. Samples of airborne particles were collected onto polyvinyl chloride filters at three distances along the prevailing downwind direction, from each source environment during a period of approximately 1 week. These samples were analyzed for 13 species or assay groups of fungi, bacterial genus Streptomyces, and Gram-positive and -negative bacteria using quantitative polymerase chain reaction (PCR). Samples for determining the concentrations of viable fungi and bacteria were collected from all environments using a six-stage impactor. The results show that there were variations in the microbial diversity between the source environments. Specifically, composting was a major source for the fungal genera Aspergillus and Penicillium, particularly for Aspergillus fumigatus, and for the bacterial genus Streptomyces. Although the microbial concentrations in the sewage treatment plant area were significantly higher than those at 50 or 200 m distance from the plant area, in the farming environment or cattle manure spreading area, no significant difference was observed between different distances from the source. In summary, elevated concentrations of microbes that differ from background can only be detected within a few hundred meters from the source. This finding, reported earlier for culturable bacteria and fungi, could thus be confirmed using molecular methods that cover both culturable and nonculturable microbial material.
Concentrations and diversity of airborne microbes increase due to particle emissions from different biological waste treatment applications. However, these emissions cannot be separated from the background concentrations after more than a few hundred meters from the source. As part of a risk assessment, it may be necessary to confirm the behavior of microbial emissions from a specific source. Quantitative PCR is a useful tool for estimating total concentrations of different microbial species or groups as it detects both culturable and nonculturable microbial material.
INTRODUCTION
Microorganisms are present in plants, soil, and aquatic environments, as well as in different kinds of man-made environments, especially locations where organic material is being handled. Due to small size of microbial spores and cells, ranging between 1 and 100 μm, they can readily be released into the air and remain airborne for long periods of time.Citation1
Major man-made sources for microbial aerosols into the outdoor air include different types of waste handling processes,Citation2 large-scale composting,Citation3,Citation4 sewage treatment,Citation5,Citation6 and animal shelters.Citation7,Citation8 Such facilities process massive amounts of materials that contain a wide diversity of microbial types, genera, and species. These activities may be significant sources of potentially pathogenic microorganisms to the surrounding environment.Citation9–11 For example, Aspergillus fumigatus and actinomycetes have been shown to be present in emissions from composts.Citation3,Citation12,Citation13 Biological waste treatment processes may be located in the vicinity of residential areas, and because of the potential spread of microorganisms of fecal origin, they may be a cause of concern to nearby residents. High concentrations of airborne microbes in various occupational settings have been linked to adverse health effects experienced by the workers in the facility, but data concerning the emissions to nearby areas and their importance to the health and well-being of nearby residents remain scarce.Citation14–16 More data are needed to establish the basis for guidelines for protective zones between the sources and residential areas.
There have been discrepancies between studies reporting on the dispersion of microbial emissions from biological waste treatment sources. Recer et al.Citation12 concluded that bioaerosol emissions from a large yard-waste composting facility may increase microbial concentrations as far as 500 m in the downwind direction from the facility. However, most studies have reported much smaller distances where elevated microbial levels can be analyzed. Sanchez-Monedero et al.Citation17 detected an approximately 100-fold increase in the concentrations of viable A. fumigatus and mesophilic bacteria 40 m downwind of a composting site during different activities in large-scale green waste composting plant in northern England. However, no significant differences to background levels were found any longer at distances of 200 and 300 m from the operational area. Similar conclusions were made by Grisoli et al.Citation18 in their study from an open composting facility and a wastewater treatment plant in Northern Italy.
Microbial concentrations in environmental samples have traditionally been determined using culture based methods. However, these methods detect exclusively viable microbes that represent only a small portion of total microbial biomass.Citation19,Citation20 Therefore, DNA-based methods, especially quantitative polymerase chain reaction (qPCR), have been developed to evaluate total microbial levels in the air and other environmental sample matrices.Citation13,Citation21–23 qPCR can be used for the detection of different microbial species or groups, including both viable and nonviable microbial material. The advantages of qPCR are its speed of analysis and the possibility to expand sampling times of airborne microbes to days, even weeks.
In order to conduct an assessment of the emissions dispersion, samples in this study were collected from four types of man-made source environments at three distances from the source using qPCR and culture techniques for microbial detection. The sampling campaigns were carried out in a waste center with composting windrows, a sewage treatment plant, a farming environment, and cattle manure spreading. Both concentrations and the diversity of the airborne microbial material were analyzed.
MATERIALS AND METHODS
Test Sites
Samples were collected from the four test sites near to the emission source and at two distances along the direction of the prevailing winds. Collection sites were selected according to predefined distances of 50 m and 150–200 m (with the exception of the waste center area), taking into account certain practical limitations for locating the sampling device (i.e., the topography of the ground, availability of electrical power outlets, etc.).
The first sampling campaign was focused on emissions from a large waste center area with composting facilities located in southern Finland. The samples were collected in September 2005. The waste center receives the municipal waste from a population of approximately 1 million. The total annual amount of waste material arriving to the waste center at the time the samples were collected was approximately 330,000 tons. The capacity of composting facilities was 30,000 t/yr (tons/year) biowaste from municipalities in surrounding areas. Two sampling sites were located in the waste center area; next to the maturation composting windrows and in the perimeter of the dumping ground, and the third site was located in the nearest population center, 2 km from the composting windrows.
The second sampling campaign was conducted in October 2006 in southern Finland in an area of a municipal sewage treatment plant that was a source of odor complaints from the nearby inhabitants (n = 20,000). Three different sampling sites were chosen: one next to the primary effluent container, one in the population center 50 m northeast of the plant area and the third in the population center 200 m northeast of the plant area.
The third sampling campaign was conducted in a farming environment over two time periods, June and November in 2007. The farm was located in eastern Finland. Sampling sites included an area next to a cowshed that housed 70 cows and areas 50 and 150 m downwind from the shed. During the sampling campaign in June, the cows spent most of the time in the pastures with the exception of the milking periods during which they were brought into the cowshed. During the November sampling, the cows were housed in the cowshed due to the cold temperatures and frequent rain.
Another sampling campaign was conducted during and after the spreading of 50 t/ha (tons/hectare) cattle manure sludge onto the 200 × 50 m field. The campaign was conducted in southern Finland in June 2006. Samples were collected in the prevailing downwind direction at three different distances from the spreading area: 10, 50, and 200 m. Background samples were collected at the same sampling sites 2 days prior to the manure spreading.
Air Sampling
Air samples were collected onto a 25-mm polyvinyl chloride (PVC) filter (0.8 µm pore size; Millipore, Bedford, MA, USA) using a button aerosol sampler (SKC Inc., Eighty Four, PA, USA).Citation24 The sampling height at each site was 1.5 to 2.5 m above ground level. The total sampling time during each period was from 6 to 7 days, except in the farming environment during November when sampling was conducted for 3 days. Filters were changed every 2 days in the waste center and daily in the remaining environments. The latter process allowed us to determine daily variation in microbial concentrations. For each sampling period, the flow rate was adjusted (with the test filter in place) to 4 L/min using a gas flow calibrator (Buck M-30; A.P. Buck Inc., Orlando, FL, USA). The flow rate was measured after each sampling during the change of filter. Because in some cases there was a reduction of the flow rate observed during the sampling period, the results are mainly expressed using the mass collected. The collected mass from the airborne dust samples was determined by weighing the filters with a microbalance (MT-5; Mettler-Toledo AG, Greifensee, Switzerland; 1 µg sensitivity) prior to and shortly following the sampling period. Filters for qPCR analyses were kept at −20 °C until analyzed.
Samples of viable bacteria and fungi were collected daily from each source environment. A six-stage impactorCitation25 (Andersen 10–800; Graseby Andersen, Atlanta, GA, USA) was used with a flow rate 28.3 L/min and with tryptone-yeast-glucose agar (TYG; Difco, Detroit, USA) for bacteria and 2 % malt-extract agar (MEA; Biokar, Beauvais, France) for fungi. The fungal and bacterial media were supplemented with chloramphenicol and natamycin in order to suppress bacterial and fungal growth, respectively. The sampling time ranged from 30 sec to 5 min depending on the environment and season. The assessment of the required sampling time was based on expected concentrations measured in the preceding study.Citation13
DNA Extraction and Quantitative PCR Analyses
DNA Extraction
Filter samples were folded into a cylinder with the sample facing inwards and placed into a sterile 2-mL extraction tube (Simport Plactics, Beloeil, Canada) containing 0.3 g glass beads (G-1277; Sigma-Aldrich, Munich, Germany). A volume of 205 μL of Qiagen Buffer AE (Hilden, Germany) was added to each tube. Ten microliter of Geotrichum candidum in 0.5% Tween 80 (2 × 108 conidia/mL) were added as a source of reference DNA. The tubes were shaken for one minute in a Mini Bead-Beater (Biospec Products, Bartlessville, USA) at maximum speed followed by centrifugation (Biofuge pico; Kendro, Hanau, Germany) for 3 min at 13,000 rpm. The liquid above the beads was removed and transferred to sterile 1.5 mL eppendorf tube. A volume of 200 mL of Buffer AE was added to each tube, followed by brief mixing and centrifugation (3 min at 13,000 rpm). The liquid was transferred into the same Eppendorf tube and stored at −80 °C.
qPCR Analyses
DNA samples were analyzed for 15 different species or assay groups of fungi and three assay groups for bacteria using previously published primers and probesCitation26,Citation27 (http://www.epa.gov/microbes/moldtech.htm) with minor modifications of previously reported methods.Citation22,Citation26–28 The analyzed species and groups of fungi included Aspergillus fumigatus/Neosartorya fischeri (Afumi), Aspergillus niger/awamori/foetidus/phoenicis (Anigr), Aureobasidium pullulans (Apull), Cladosporium cladosporioides (Cclad1), Cladosporium herbarum (Cherb), Cladosporium sphaerospermum (Cspha), Eurotium amstelodami/chevalieri/herbariorum/rubrum/repens (Eamst), Epicoccum nigrum (Enigr), Penicillium brevicompactum/stoloniferum (Pbrev), Penicillium chrysogenum (Pchry), Penicillium variabile (Pvarb2), Wallemia sebi (Wsebi), universal Penicillium/Aspergillus/Paecilomyces variotii (PenAsp) group, Cladosporium spp. (Clado), Streptomyces spp. (Strepto), and Gram-positive and -negative bacteria (Gram+/−).
Reaction mixtures of 25 μL were prepared in 96-well optical reaction plates (Applied Biosystems, Foster City, USA) or 0.1-mL tubes (Clado and Gram+/−; Corbett Research, Sydney, Australia). The reaction mixtures contained 1× concentrated Universal Master Mix (Applied Biosystems, Warrington, UK; fungal assays excluding Clado, and Strepto) or Dynamo Probe qPCR Kit (Finnzymes, Espoo, Finland; Clado and Gram+/− assays); forward and reverse primers in a final concentration 1 μM each (fungal assays except Clado), 0.3 μM each (Clado), 0.4 μM each (Strepto), or 0.8 μM each (Gram+/−), and TaqMan probe at a final concentration 80 nM (fungal assays excluding Clado), 300 nM (Clado), or 200 nM (Strepto and Gram+/−). Primers and probes were purchased from Oligomer (Espoo, Finland) and Applied Biosystems. Bovine serum albumin (BSA; New England BioLabs, Ipswich, USA) was added to all reactions to a final concentration 0.2 mg/mL. A total amount of 2 μL sample DNA was added to the reactions. Waste center area samples were analyzed in a total volume of 12.5 μL with 2.5 μL of sample DNA.
PCR amplifications were performed using an Applied Biosystems ABIPrism model 7000 sequence detection system or Rotor-Gene 3000 (Corbett Research; Clado and Gram+/− assays). The thermal cycles for fungi except Clado consisted of 2 min at 50 °C, 10 min at 95 °C (initial denaturation), followed by 40 cycles of 15 sec at 95 °C for template denaturation and 1 min at 60 °C for probe and primers annealing and template extension. For Strepto, the cycles were as described above, but the annealing step was performed at 58 °C. The cycling program of the Cladosporium and Gram+/− qPCR was as follows: initial denaturing step 15 min at 95 °C, followed by 35 cycles of the denaturing step 15 sec at 95 °C and the annealing/extension step of 60 sec at 60 °C for Clado and 45 sec at 64 °C for Gram+/−.
Quantification was performed using the comparative cycle threshold method according to Brinkman et al.Citation28 and Haugland et al.Citation29 Geotrichum candidum spores were added prior to DNA extraction as an exogenous internal control and analyzed with their own primer/probe set to normalize the results for differences in the DNA extraction efficiency and PCR inhibition.
Positive and negative controls were included every time that the analyses were performed. The positive-control samples containing spores or cells from all species to be analyzed were prepared for fungi and bacteria separately. DNA from positive control samples was isolated and subjected to qPCR analyses along with the other samples. At least two negative controls including nuclease-free water instead of sample DNA were performed on each qPCR run.
Culturable Bacteria and Fungi
Culture plates from the impactor samples were removed and incubated for 7 days at 25 °C. Total numbers of bacterial and fungal colonies were counted. Fungal genera were identified morphologically using an optical microscope. Bacterial samples (i.e., TYG plates) were incubated for an additional 7 days after which the actinomycete colonies were counted. Colony counts were corrected using the positive hole correction factor. Results were expressed as colony forming units (cfu) per cubic meter of air (cfu/m3).
Statistical Analyses
Nonparametric statistical analyzes were used because the concentrations of microbes were not normally distributed. Daily variation and differences between sampling sites were tested by the nonparametric Kruskal-Wallis test and further analyzed by Dunn's test. Seasonal differences in the farming environment were tested using Mann-Whitney test. Statistical analyses were performed with the program SPSS statistics version 17.0 (SPSS, Chicago, IL, USA).
RESULTS
Microbial Concentrations across Test Sites
The most common fungal group in all environments was Cladosporium spp., even though microbial concentrations and diversity varied between different waste handling processes. The concentration range of total Cladosporium spp. based on qPCR was 0–3.0 × 107 cells/mg or 0–5.2 × 105 cells/m3 and that of viable Cladosporium spp. based on culture methods was 0–1.2 × 104 cfu/m3. The genera Penicillium and Aspergillus were also frequently detected ranging from 9.9 × 101 to 5.2 × 105 cells/mg or 1.5 × 100 to 4.2 × 104 cells/m3 in total counts and from 0 to 6.2 × 103 cfu/m3 in viable counts. Bacteria were present at high concentrations in the farming environment (104–108 cells/mg or 102–106 cells/m3) and at somewhat lower levels in the vicinity of the sewage treatment plant (103–105 cells/mg or 102–104 cells/m3).
Waste Center Area
The results of the microbial qPCR analyses in three different locations, i.e., composting windrows, dumping ground, and the nearby residential area, are shown in and in . The most prevalent fungal group at all three sampling sites was Cladosporium spp. The two Cladosporium species analyzed, C. herbarum and C. cladosporioides, were commonly present in the samples, but these two species accounted for less than 1% of the total Cladosporium spp. There were no significant differences between the sampling sites with respect to concentrations of Cladosporium spp. or the two Cladosporium species analyzed. Total concentrations of Penicillium/Aspergillus/P. variotii group were two orders of magnitude higher adjacent to the composting windrows (P < 0.05) than in the perimeter of the dumping ground. The same trend was observed for all separately analyzed Penicillium and Aspergillus species, specifically for A. fumigatus, A. niger/awamori, and P. variabile (). Results also indicated that concentrations of Eurotium spp., Wallemia sebi, and Streptomyces spp. were highest next to the composting windrows.
Table 1. Median, minimum, and maximum concentrations of fungal and bacterial groups from different types of emission sources, determined with qPCR and expressed as cells/m3
Figure 1. Microbial concentrations within and in the vicinity of the waste centre as determined by qPCR analyses. The columns represent geometric mean concentrations and 95% confidence intervals of three measurements at each site. The sampling sites were next to the composting windrows, on the dumping ground and in the nearby population center. Notes: Clado = Cladosporium spp.; Cherb = Cladosporium herbarum; Cclad1 = Cladosporium cladosporioides; PenAsp = Penicillium/Aspergillus/Paecilomyces variotii; Afumi = Aspergillus fumigatus/Neosartorya fischeri; Anigr = Aspergillus niger/awamori/foetidus/phoenicis; Pbrev = Penicillium brevicompactum/stoloniferum; Pchry = Penicillium chrysogenum; Pvarb2 = Penicillium variabile; Apull = Aureobasidium pullulans; Eamst = Eurotium amstelodami/chevalieri/herbariorum/rubrum/repens; Enigr = Epicoccum nigrum; Wsebi = Wallemia sebi; Strepto = Streptomyces spp.
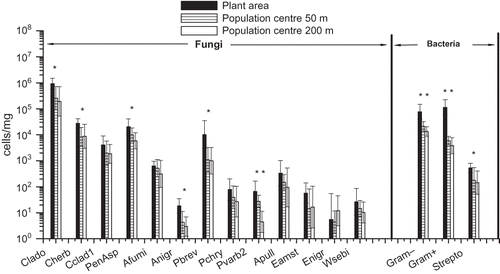
Culturable bacterial concentrations were highest next to the composting windrows and in the perimeter of the dumping ground, but were significantly lower at the population center (). This trend was also observed with respect to viable actinomycetes (P = 0.012; median concentrations of 39 cfu/m3 adjacent to the composting windrows, 18 cfu/m3 in the perimeter of the dumping ground, and 0 cfu/m3 at the population center). Concentrations of viable fungi were also the highest next to the composting windrows and in the perimeter of the dumping ground but the differences to the residential area were not statistically significant.
Figure 2. Total concentration of viable bacteria and fungi in three sampling points downwind from the emission source. The columns represent the geometric mean of 2–10 measurements with an Andersen six-stage impactor. Sampling point 1 (black column) is next to the emission source and 2 and 3 as increasing distances. In the waste center area, sampling point 1 is in next to the composting windrows, sampling point 2 in perimeter of the dumping ground, and sampling point 3 in a population center. *P < 0.05; **P < 0.01.
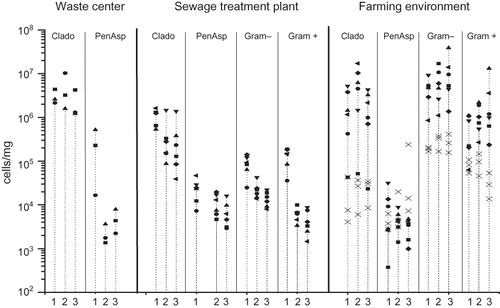
Sewage Treatment Plant
The concentrations of microbial groups and species analyzed with qPCR at three distances (10, 50, 200 m) from the sewage treatment plant are presented in and in . The concentrations were highest around the plant area for all microbes with the exception of E. nigrum. Differences between sampling sites were statistically significant for Cladosporium spp., C. herbarum, Penicillium/Aspergillus/P. variotii group, A. niger/awamori/foetidus/phoenicis, P. brevicompactum/stoloniferum, P. variabile, Gram-positive and -negative bacteria, and for Streptomyces spp. The concentration gradient was clearest for P. variabile and for Gram-positive and -negative bacteria (P < 0.01 for each). Viable bacterial concentrations at the plant area were about four times higher than they were in 50 or 200 m from the plant area (), but no difference was observed between these two sampling sites.
Farming Environment
In the farm, samples were taken next to the cowshed and at 50 and 150 m along the prevailing downwinds. With respect to fungi, the highest concentrations were detected for Cladosporium spp. Concentrations of Gram-positive and -negative bacteria were also high ( and B and ). The prevalent fungal groups in the samples were Penicillium/Aspergillus/P. variotii group, P. brevicompactum/stoloniferum, C. herbarum, C. cladosporioides, A. pullulans, and among bacteria, Streptomyces spp. No statistically significant differences were observed in microbial concentrations between sampling sites in the farming environment.
Figure 4. Geometric mean concentrations and 95% confidence intervals of fungal species/groups, Gram-positive and -negative bacteria and the bacterium Streptomyces spp. in the farming environment next to the cowshed and in two locations at different distances on the prevailing downwinds from the cowshed, determined with qPCR (n = 6 at each site in June and n = 3 at each site in November). J = June; N = November. For qPCR assay abbreviations, see *P < 0.05; **P < 0.01.
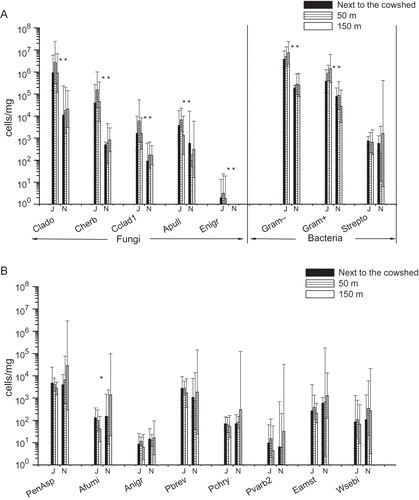
The concentrations of Cladosporium spp., two Cladosporium species, A. pullulans, E. nigrum, and Gram-positive and -negative bacteria were significantly higher in June than in November ( and ), but the concentration of A. fumigatus was higher in November (). For other microbial species and groups analyzed with qPCR, no major differences between months were observed. The significantly higher concentrations in June versus November were consistent for viable fungi and bacteria ().
Cattle Manure Sludge Spreading
The concentrations of microbial species and groups were determined before, during, and 1–5 days after the spreading of cattle manure. The highest concentrations determined with qPCR were observed for Cladosporium spp. and for Gram-positive and -negative bacteria ( and B and ). Significant differences in concentrations between days were observed for Cladosporium spp. (P = 0.031), C. herbarum (P = 0.024), C. cladosporioides (P = 0.019), A. pullulans (P = 0.038), E. nigrum (P = 0.034), W. sebi (P = 0.024), Streptomyces spp. (P = 0.022), and for Gram-positive and -negative bacteria (P = 0.031 and 0.027, respectively). With respect to these microbial species and groups, the concentrations increased slightly after 0 to 1 day of manure spreading and significantly after 1 to 3 days of manure spreading from the concentrations before spreading. Generally, the concentrations decreased slightly from 3 to 5 days after spreading, but not to the level prior to the spreading process. The trend in viable bacterial concentrations was very similar to that described above with one exception, which was detected in the samples taken 4–5 days post spreading in a location 150 m from the spreading site. There were no statistically significant differences between the test distances from the spreading area.
Figure 5. Concentrations of fungi and bacteria before, during, and 1–5 days after cattle manure spreading in three prevailing downwind distances from the spreading area, determined with qPCR. The four columns in a group represent the four sampling times; from left to right: before, 0–1 days after, 1–3 days after, and 3–5 days after manure spreading. For qPCR assay abbreviations, see
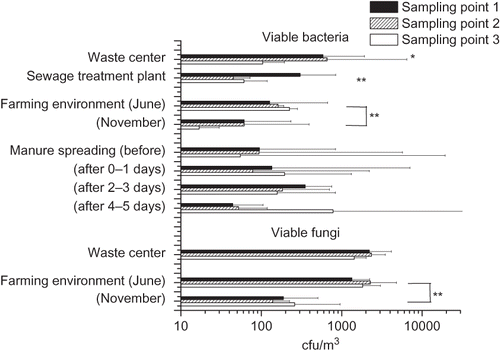
Daily Variation of Concentrations
In the waste center area and its surroundings, the largest day-to-day variation was observed for Penicillium/Aspergillus/P. variotii group (). In the sampling site next to the composting windrows, the concentration varied from 1.7 × 104 up to 5.2 × 105 cells/mg. However, the variation was not statistically significant. In sewage treatment area and the surroundings, notable daily variation was detected for Cladosporium spp. in 50 m (range 8.7 × 104 to 1.5 × 106 cells/mg) and 200 m (range 3.9 × 104 to 1.4 × 106 cells/mg). With respect to the other microbial groups, the variation did not exceed an order of magnitude difference at any sampling point. In the farming environment, the daily variation in June was larger than that in the waste center or the sewage treatment plant areas and surroundings. This was especially the case for Cladosporium spp. in all sampling sites (range 2.3 × 104 to 1.7 × 107 cells/mg), Penicillium/Aspergillus/P. variotii group in the site next to the cowshed (range 3.7 × 102 to 3.2 × 104 cells/mg), and Gram-negative (range 1.4 × 106 to 3.9 × 107 cells/mg) and -positive (range 2.4 × 105 to 1.3 × 107 cells/mg) bacteria at 150 m from the cowshed. The variation was less obvious in November, with the exception of the values in the Penicillium/Aspergillus/P. variotii group in 150 m from the cowshed.
Figure 6. Daily variation in the concentrations of Cladosporium spp. (Clado), Penicillium/Aspergillus/P. variotii group (PenAsp), and Gram-positive and -negative bacteria measured with qPCR. Different symbols in the vertical lines illustrate the concentrations measured on each individual day. The numbers (1–3) in the x-axis represent the three distances from the emission source. In the farming environment, the concentrations measured in November are marked with a cross (×).
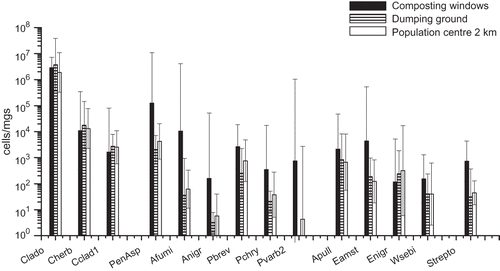
DISCUSSION
There are a wide variety of natural sources for airborne fungi and bacteria, but many human activities can cause significantly increased microbial concentrations in outdoor air. Examples of man-made sources include waste handling processes, large-scale composting, wastewater treatment, and various types of agricultural activities. Microbial emissions from these sources can represent a major concern for people living near to the source. Probably the greatest cause of alarm is the possibility of the spread of infectious and pathogenic microorganisms. Although this study was not specifically focused on detection of infectious agents, one could predict that their transmission from the sources would probably follow the patterns demonstrated here. These measurements represent a basis for future risk assessment of such emissions.
Microbial Diversity in Different Environments
Cladosporium was the most common fungal genus observed in our study. This genus is generally considered to be one of the most prevalent fungi in outdoor air.Citation30–32 Airborne concentrations of Cladosporium spp. varied between 0 and 5.2 × 105 cells/m3 (median 2.4 × 104 cells/m3) when analyzed with qPCR and between 0 and 1.2 × 104 cfu/m3 (median 3.7 × 102 cfu/m3) when using culture-based method. Other common fungal genera included Penicillium and Aspergillus in combined concentrations 1.5–4.2 × 104 cells/m3 (qPCR) and 0–6.2 × 103 cfu/m3 (culture). Viable culture results were generally 1 to 2 orders of magnitude lower than the concentrations determined using qPCR. This observation is consistent with our preliminary study. Culture methods determine only the number of viable microbes and fail to assess the nonviable and/or nonculturable microbial presence. The results in this study suggest that viable microbes represent only a small percentage of total microbial material in the outdoor air.
According to our results, composting is a major source for A. fumigatus and Streptomyces spp. This is in support of previous studies concerning microbiological emissions from composting facilities.3,12 The results also demonstrated that total concentrations of Penicillium/Aspergillus genera and all of the analyzed species in these genera were higher in samples collected next to the composting windrows in comparison to those taken in the perimeter of the dumping ground or a population center. Interestingly, total viable bacteria concentrations were significantly higher both next to the composting windrows and in the perimeter of dumping ground compared to the situation near to the population center, whereas high total Streptomyces spp. concentrations were detected only in emissions around composting windrows. These results indicate that composting is a significant source for fungal genera of Penicillium and Aspergillus and actinomycetes. Studies about the microbial communities present in composts support this conclusion.Citation33–35 However, the concentrations of Cladosporium spp. and two Cladosporium species were rather similar at all sampling sites. This suggests that composting is not a significant source for Cladosporium spp. The concentrations of the species C. herbarum and C. cladosporioides were less than 1% of the total concentration of Cladosporium spp. Although these species are considered as being some of the most common Cladosporium species, these results suggest that other species may actually predominate. In fact, there are approximately 28–40 Cladosporium speciesCitation36 and the specificity of the primers and/or probe used in Cladosporium spp. analyses is difficult to assess at this point.
Wastewater treatment is a potential microbial source to the surrounding environment. Our results indicated that the concentrations of Cladosporium spp. were at their highest at the sewage treatment plant area and a concentrations gradient was seen downwind from the source. Therefore, sewage treatment can be viewed as a source of Cladosporium spp. However, wastewater treatment was most significantly a source of bacteria, especially Gram-positive bacteria. In addition, even though Penicillium and Aspergillus genera and species could be connected to wastewater treatment, they were not as dominant in the sewage treatment plant area as they were in the composting area.
Animal housing and associated microbial emissions may also be an issue from a public health perspective. However, microbial concentrations in the farming environment in this study were quite similar to those observed in normal rural areas during the same seasons in our preliminary study.Citation13 This suggests that modern cowsheds are not a significant source of microbial contaminants into the outdoor air.
It is a common practice to use manure sludge as a source of nutrients on farmland and the spreading of this sludge can represent a significant source of microbial emissions. Our results demonstrated that manure spreading was followed by increased concentrations of airborne Cladosporium and bacteria. Although the levels were somewhat higher on the first day after spreading, a statistically significant increase was observed only 1–3 days after the spreading. This observation does not have any evident explanation, but since manure sludge is very watery, it is possible that the aerosol only started to develop when the surface dried out.
Dispersion of Microbes to the Environment
An important goal in the study was to determine how far from the emission source microbes disperse in detectable concentrations. Compared to the situation within sewage treatment plant itself, microbial concentrations had declined dramatically already at 50 m distance from the plant, and were only marginally higher than baseline at 50–200 m from the site. This is in line with most other studies concerning the distances of microbial dispersion from biological waste treatment applications.Citation17,Citation18
In the farming environment we did not see any significant differences in microbial levels between different distances from the cowshed. The concentrations remained within the range of background rural areas.Citation13 The highest individual concentrations of bacteria were observed at the site 150 m from the cowshed. The samples were taken from prevailing downwind direction predicted for the 1-day sampling period. The 150 m sampling point was usually located next to the route along which the cows walked on their way to pasture, which may have caused the increased bacterial concentrations.
Furthermore, we did not see any significant variation when comparing the microbial concentrations between different distances from the manure spreading area. Even though the samples were taken in the detection of the prevailing wind, both the wind direction and weather conditions varied during the whole sampling period. This may have influenced the results such that the distances that the microbes were transported cannot be precisely assessed.
In conclusion, our results suggest that microbial emissions from biological waste treatment processes are not dispersed at significant concentrations over more than a few hundred meters. However, there are several different factors that affect the microbial dispersion and their release from the source matrix. These factors include plant or source type,Citation37–39 stage of the process,Citation2,Citation10 different activities,Citation3,Citation4,Citation40,Citation41 and wind direction.Citation18,Citation42,Citation43 As the variation of the concentrations may be remarkable, and sporadic high peaks may occur, no exact estimates of the microbial emissions can be made. Precautionary policies in area planning may be justified, especially in the case of the spread of potential pathogens.
Analysis Methods
Although qPCR is commonly used for microbial analysis in environmental samples, there are few earlier qPCR studies investigating microbial outdoor air concentrations. We used filter sampling and were able to conduct both DNA based microbial qPCR analyses and measurement of viable microbes. The sampling time for filters can be much longer, hours as compared to 1–15 min sampling with the impactor. In this study, filter samples were collected for 1 or 2 days. Even though daily variation was in some cases extensive, a long sampling time would be expected to even out the differences.
The results demonstrated that the qPCR method is a promising tool to estimate microbial emissions from different biological sources. However, there is still much to learn before this method can be routinely used in order to determine the microbial quality of outdoor air. Special attention may be needed to avoid any possible bias due to the unspecificity of qPCR assays.
CONCLUSIONS
Microbial diversity varies between different sources of biological particle emissions. The following conclusions can be drawn from our study:
• | Composting is a major source of the fungal genera Penicillium and Aspergillus, especially A. fumigatus, and among the bacteria, actinomycetes such as Streptomyces spp. | ||||
• | Wastewater treatment plants are a source of both culturable and non-culturable bacteria, especially Gram-positive bacteria, and less so for fungi, as seen from the qPCR results. | ||||
• | Cattle farming does not contribute significantly to the background levels of airborne microbes in rural areas. | ||||
• | Spreading of wet manure sludge may represent a source of bacteria and fungi after the surface of the area sprayed has dried. | ||||
• | Cladosporium is the most prevalent fungal genus in the outdoor air in countries with a cool climate, as seen from the qPCR results. Since its main sources are natural, it cannot be used as an indicator organism of microbial emissions from man-made sources. | ||||
• | Microbial emissions from various sources of biological waste handling can be observed at distances no more than few hundred meters at maximum, after which they cannot be differentiated from the background concentrations. | ||||
• | qPCR is a promising tool to estimate microbial emissions from different biological sources. |
ACKNOWLEDGMENTS
This study was financially supported by the Ministry of Social Affairs and Health, Academy of Finland (grant no. 111177), Ekokem Oy Ab, and Graduate School in Environmental Health. The authors want to thank Asko Vepsäläinen for his excellent help in statistical analyses. The collaboration with the local environmental authorities and the personnel in the facilities included in the study is greatly appreciated.
REFERENCES
- Kasprzyk , I. 2008 . Aeromycology—Main Research Fields of Interest during the Last 25 Years . Ann. Agric. Environ. Med. , 15 : 1 – 7 .
- Kiviranta , H. , Tuomainen , A. , Reiman , M. , Laitinen , S. , Nevalainen , A. and Liesivuori , J. 1999 . Exposure to Airborne Microorganisms and Volatile Organic Compounds in Different Types of Waste Handling . Ann. Agric. Environ. Med. , 6 : 39 – 44 .
- Taha , M.P.M. , Drew , G.H. , Longhurst , P.J. , Smith , R. and Pollard , S.J.T. 2006 . Bioaerosol Releases from Compost Facilities: Evaluating Passive and Active Source Terms at a Green Waste Facility for Improved Risk Assessments . Atmos. Environ. , 40 : 1159 – 1169 .
- Fischer , G. , Albrecht , A. , Jackel , U. and Kampfer , P. 2008 . Analysis of Airborne Microorganisms, MVOC and Odour in the Surrounding of Composting Facilities and Implications for Future Investigations . Int. J. Hyg. Environ. Health , 211 : 132 – 142 .
- Cyprowski , M. , Sowiak , M. , Soroka , P.M. , Buczynska , A. , Kozajda , A. and Szadkowska-Stanczyk , I. 2008 . Assessment of Occupational Exposure to Fungal Aerosols in Wastewater Treatment Plants . Med. Pr. , 59 : 365 – 371 .
- Sanchez-Monedero , M. A. , Aguilar , M. I. , Fenoll , R. and Roig , A. 2008 . Effect of the Aeration System on the Levels of Airborne Microorganisms Generated at Wastewater Treatment Plants . Water Res. , 42 : 3739 – 3744 .
- Ko , G. , Simmons , O.D. 3rd , Likirdopulos , C.A , Worley-Davis , L. , Williams , M. and Sobsey , M.D. 2008 . Investigation of Bioaerosols Released from Swine Farms Using Conventional and Alternative Waste Treatment and Management Technologies . Environ. Sci. Technol. , 42 : 8849 – 8857 .
- Radon , K. , Danuser , B. , Iversen , M. , Monso , E. , Weber , C. , Hartung , J. , Donham , K.J. , Palmgren , U. and Nowak , D. 2002 . Air Contaminants in Different European Farming Environments . Ann. Agric. Environ. Med. , 9 : 41 – 48 .
- Fracchia , L. , Pietronave , S. , Rinaldi , M. and Martinotti , M.G. 2006 . The Assessment of Airborne Bacterial Contamination in Three Composting Plants Revealed Site-Related Biological Hazard and Seasonal Variations . J. Appl. Microbiol. , 100 : 973 – 984 .
- Prazmo , Z. , Krysinska-Traczyk , E. , Skorska , C. , Sitkowska , J. , Cholewa , G. and Dutkiewicz , J. 2003 . Exposure to Bioaerosols in a Municipal Sewage Treatment Plant . Ann. Agric. Environ. Med. , 10 : 241 – 248 .
- Gibbs , S.G. , Green , C.F. , Tarwater , P. M. and Scarpino , P. V. 2004 . Airborne Antibiotic Resistant and Nonresistant Bacteria and Fungi Recovered from Two Swine Herd Confined Animal Feeding Operations . J. Occup. Environ. Hyg. , 1 : 699 – 706 .
- Recer , G.M. , Browne , M.L. , Horn , E.G. , Hill , K.M. and Boehler , W. F. 2001 . Ambient Air Levels of Aspergillus fumigatus and Thermophilic Actinomycetes in a Residential Neighborhood Near a Yard-Waste Composting Facility . Aerobiologia , 17 : 99 – 108 .
- Kaarakainen , P. , Meklin , T. , Rintala , H. , Hyvärinen , A. , Kärkkäinen , P. , Vepsalainen , A. , Hirvonen , M.R. and Nevalainen , A. 2008 . Seasonal Variation in Airborne Microbial Concentrations and Diversity at Landfill, Urban and Rural Sites . Clean , 36 : 556 – 563 .
- Domingo , J.L. and Nadal , M. 2009 . Domestic Waste Composting Facilities: A Review of Human Health Risks . Environ. Int. , 35 : 382 – 389 .
- Fischer , G. and Dott , W. 2003 . Relevance of Airborne Fungi and Their Secondary Metabolites for Environmental, Occupational and Indoor Hygiene . Arch. Microbiol. , 179 : 75 – 82 .
- Pillai , S.D. and Ricke , S.C. 2002 . Bioaerosols from Municipal and Animal Wastes: Background and Contemporary Issues . Can. J. Microbiol. , 48 : 681 – 696 .
- Sanchez-Monedero , M.A. , Stentiford , E.I. and Urpilainen , S.T. 2005 . Bioaerosol Generation at Large-Scale Green Waste Composting Plants . J. Air Waste Manag. Assoc. , 55 : 612 – 618 .
- Grisoli , P. , Rodolfi , M. , Villani , S. , Grignani , E. , Cottica , D. , Berri , A. , Picco , A.M. and Dacarro , C. 2009 . Assessment of Airborne Microorganism Contamination in an Industrial Area Characterized by An Open Composting Facility and a Wastewater Treatment Plant . Environ. Res. , 109 : 135 – 142 .
- Niemeier , R.T. , Sivasubramani , S.K. , Reponen , T. and Grinshpun , S.A. 2006 . Assessment of Fungal Contamination in Moldy Homes: Comparison of Different Methods . J. Occup. Environ. Hyg. , 3 : 262 – 273 .
- Toivola , M. , Nevalainen , A. and Alm , S. 2004 . Personal Exposures to Particles and Microbes in Relation to Microenvironmental Concentrations . Indoor Air , 14 : 351 – 359 .
- Rintala , H. and Nevalainen , A. 2006 . Quantitative Measurement of Streptomycetes Using Real-Time PCR . J. Environ. Monit. , 8 : 745 – 749 .
- Meklin , T. , Haugland , R.A. , Reponen , T. , Varma , M. , Lummus , Z. , Bernstein , D. , Wymer , L.J. and Vesper , S.J. 2004 . Quantitative PCR Analysis of House Dust Can Reveal Abnormal Mold Conditions . J. Environ. Monit. , 6 : 615 – 620 .
- Vesper , S.J. , Wymer , L.J. , Meklin , T. , Varma , M. , Stott , R. , Richardson , M. and Haugland , R.A. 2005 . Comparison of Populations of Mould Species in Homes in the UK and USA Using Mould-Specific Quantitative PCR . Lett. Appl. Microbiol. , 41 : 367 – 373 .
- Kalatoor , S. , Grinshpun , S.A. and Willeke , K. 1995 . New Aerosol Sampler with Low Wind Sensitivity and Good Filter Collection Uniformity . Atmos. Environ. , 29 : 1105 – 1112 .
- Andersen , A.A. 1958 . New Sampler for the Collection, Sizing, and Enumeration of Viable Airborne Particles . J. Bacteriol. , 76 : 471 – 484 .
- Pietarinen , V.M. , Rintala , H. , Hyvarinen , A. , Lignell , U. , Karkkainen , P. and Nevalainen , A. 2008 . Quantitative PCR Analysis of Fungi and Bacteria in Building Materials and Comparison to Culture-Based Analysis . J. Environ. Monit. , 10 : 655 – 663 .
- Kärkkäinen , P. , Valkonen , M. , Hyvärinen , A. , Nevalainen , A. and Rintala , H. 2010 . Determination of Bacterial Load in House Dust Using New Quantitative PCR, Chemical Markers and Culture . J. Environ. Monit. , 12 : 759 – 768 .
- Brinkman , N.E. , Haugland , R.A. , Wymer , L.J. , Byappanahalli , M. , Whitman , R.L. and Vesper , S.J. 2003 . Evaluation of a Rapid, Quantitative Real-Time PCR Method for Enumeration of Pathogenic Candida Cells in Water . Appl. Environ. Microbiol. , 69 : 1775 – 1782 .
- Haugland , R.A. , Varma , M. , Wymer , L.J. and Vesper , S.J. 2004 . Quantitative PCR Analysis of Selected Aspergillus, Penicillium and Paecilomyces Species . Syst. Appl. Microbiol. , 27 : 198 – 210 .
- Fang , Z. , Ouyang , Z. , Hu , L. , Wang , X. , Zheng , H. and Lin , X. 2005 . Culturable Airborne Fungi in Outdoor Environments in Beijing, China . Sci. Total. Environ. , 350 : 47 – 58 .
- Adhikari , A. , Sen , M.M. , Gupta-Bhattacharya , S. and Chanda , S. 2004 . Airborne Viable, Non-Viable, and Allergenic Fungi in a Rural Agricultural Area of India: A 2-Year Study at Five Outdoor Sampling Stations . Sci. Total Environ. , 326 : 123 – 141 .
- Bugajny , A. , Knopkiewicz , M. , Piotraszewska-Pajaw , A. , Sekulska-Stryjakowska , M. , Stach , A. and Filipiak , M. 2005 . On the Microbiological Quality of the Outdoor Air in Poznan, Poland . Polish J. Environ. Studies , 14 : 287 – 293 .
- McCarthy , A.J. and Williams , S.T. 1992 . Actinomycetes as Agents of Biodegradation in the Environment—A Review . Gene , 115 : 189 – 192 .
- Ryckeboer , J. , Mergaert , J. , Coosemans , J. , Deprins , K. and Swings , J. 2003 . Microbiological Aspects of Biowaste during Composting in a Monitored Compost Bin . J. Appl. Microbiol. , 94 : 127 – 137 .
- Anastasi , A. , Varese , G.C. and Marchisio , V.F. 2005 . Isolation and Identification of Fungal Communities in Compost and Vermicompost . Mycologia , 97 : 33 – 44 .
- EMLab P&K, L. Cladosporium sp http://www.emlab.com/app/fungi/Fungi.po?event=fungi&type=primary&species=13 (http://www.emlab.com/app/fungi/Fungi.po?event=fungi&type=primary&species=13)
- Bauer , H. , Fuerhacker , M. , Zibuschka , F. , Schmid , H. and Puxbaum , H. 2002 . Bacteria and Fungi in Aerosols Generated by Two Different Types of Wastewater Treatment Plants . Water Res. , 36 : 3965 – 3970 .
- Tolvanen , O.K. and Hanninen , K.I. 2007 . Occupational Hygiene in two Combined-Drum-and-Tunnel Composting Plants Managing Source Separated Biowaste and Sludge . J. Environ. Health , 69 : 64 – 71 .
- Zucker , B.A. , Trojan , S. and Muller , W. 2000 . Airborne Gram-Negative Bacterial Flora in Animal Houses . J. Vet. Med. B , 47 : 37 – 46 .
- Adhikari , A. , Reponen , T. , Lee , S.A. and Grinshpun , S.A. 2004 . Assessment of Human Exposure to Airborne Fungi in Agricultural Confinements: Personal Inhalable Sampling versus Stationary Sampling . Ann. Agric. Environ. Med. , 11 : 269 – 277 .
- Maricou , H. , Verstraete , W. and Mesuere , K. 1998 . Hygienic Aspects of Biowaste Composting: Airborne Microbial Concentrations as a Function of Feedstock, Operation and Season . Waste Manag. Res. , 16 : 304 – 311 .
- Hryhorczuk , D. , Curtis , L. , Scheff , P. , Chung , J. , Rizzo , M. , Lewis , C. , Keys , N. and Moomey , M. 2001 . Bioaerosol Emissions from a Suburban Yard Waste Composting Facility . Ann. Agric. Environ. Med. , 8 : 177 – 185 .
- Duan , H. , Chai , T. , Cai , Y. , Zhong , Z. , Yao , M. and Zhang , X. 2008 . Transmission Identification of Escherichia coli Aerosol in Chicken Houses to Their Environments Using ERIC-PCR . Sci. China C. Life Sci. , 51 : 164 – 173 .