Abstract
Cholesterol is regarded as a signaling molecule in regulating the metabolism and function of fat cells, in which 7-Dehydrocholesterol reductase (DHCR7) is a key enzyme that catalyzes the conversion of 7-dehydrocholesterol to cholesterol, however, the exact function of DHCR7 in goat adipocytes remains unknown. Here, the effect of DHCR7 on the formation of subcutaneous and intramuscular fat in goats was investigated in vitro, and the result indicated that the mRNA level of DHCR7 showed a gradual downward trend in subcutaneous adipogenesis, but an opposite trend in intramuscular adipogenesis. In the process of subcutaneous preadipocytes differentiation, overexpression of DHCR7 inhibited the expression of adipocytes differentiation marker genes (CEBP/α, CEBP/β, SREBP1 and AP2), lipid metabolism-related genes (AGPAT6, FASN, SCD1 and LPL), and the lipid accumulation. However, in intramuscular preadipocyte differentiation, DHCR7 overexpression showed a promoting effect on adipocyte differentiation marker genes (CEBP/α, CEBP/β, PPARγ and SREBP1) and lipid metabolism-related genes (GPAM, AGPAT6, DGAT1 and SCD1) expression, and on lipid accumulation. In summary, our work demonstrated that DHCR7 played an important role in regulating adipogenic differentiation and lipid metabolism in preadipocytes in goats, which is of great significance for uncovering the underlying molecular mechanism of adipocyte differentiation and improving goat meat quality.
Introduction
The growth and development of adipose tissue is one of the key factors affecting the quality of goat meat, and the deposition of adipose tissue is a process of continuous lipid aggregation of preadipocytes and differentiation into mature adipocytes.Citation1 The differentiation of preadipocytes to adipocytes is regulated by various transcription factors, protein factor regulators, and signal transduction pathways.Citation2,Citation3 Many studies have shown that preadipocytes are present throughout adulthood and exhibit adipose depot specificity.Citation2 Goat adipose tissues can be divided into subcutaneous adipose tissue (SAT), intramuscular fat (IMF), and visceral adipose tissue (VAT), depending on the location of deposition. However, the distribution of adipose tissues affects meat flavor and carcass quality,Citation4,Citation5 adipocyte growth and lipidoses in muscle tissue determine general IMF content.Citation6,Citation7 It has been proved that SAT contains high levels of saturated fatty acid (SFA) and excessive intake of SFA by humans may contribute to the development of diseases such as atherosclerosis and coronary heart disease.Citation8,Citation9 Fatty acid accumulation in adipose tissues as a major source of essential nutrients can act as signaling molecule to regulate adipocyte differentiation.Citation10 Therefore, understanding the molecular mechanisms of fat deposition is crucial for livestock and human health and for improving meat quality and achieving breeding goals.
Pre-laboratory, proteomic comparison of intramuscular preadipocytes (P_IMA) and intramuscular adipocytes (IMA) in goats identified a series of proteins that may play important roles in intramuscular adipose differentiation, such as SRSF10, CSRP3, APOH, CRTC2, FOS, DHCR7 and AIF1L. KEGG enrichment analysis of differentially abundant proteins (DAPs) also identified two pathways in intramuscular adipose differentiation: cholesterol metabolism and the glucagon signaling pathway. Both pathways may major involve in lipid metabolism and cell differentiation. 7-dehydrocholesterol reductase (DHCR7) is one of the proteins in the protein-protein interactions (PPI) network and is the key enzyme in the Kandutsch-Russell pathway that catalyzes the conversion of 7-dehydrocholesterol to cholesterol.Citation11,Citation12 It has been reported that inhibition of DHCR7 affects Hedgehog (Hh) signaling,Citation13,Citation14 a decrease in Smoothened (Smo) activity due to cholesterol deficiency.Citation15 The Hedgehog signaling pathway controls cell fate, proliferation and differentiation and aberrant activation of this signaling pathway can cause tumorigenesis and development. In addition, in mammals, overexpression of wild-type or mutant DHCR7 in NIH3T3 cells reduced Hh signaling.Citation16 Knock out of the DHCR7 gene in mice led to a complete loss of endogenous cholesterol biosynthesis in the liver and brain, resulting in early postnatal death.Citation17 Cholesterol is an important component and regulator of the lipid raft region of the cell membrane, and a signaling molecule that directly regulates the metabolism and function of adipocytes.Citation18,Citation19 Cholesterol depletion could interfere with internalization of lipid rafts and increase the Rho activity. This would activate the family of linker proteins—ezrin, radixin and moesin (ERM proteins) and the Rho-substrate Rho-kinase (ROCK), which enhances the cell stiffness, induce spread cellular morphology and inhibits adipogenesis.Citation20 It is interesting to note that recent studies indeed suggested that modulation of the Rho/ROCK signaling alter adipogenesis and adipocyte differentiation.Citation21,Citation22
Improving the degree of intramuscular fat deposition and reducing subcutaneous fat deposition are important and valuable research contents in meat industry. Studies have shown that the mechanisms of deposition of subcutaneous adipose tissue and intramuscular adipose tissue in animals differ,Citation23 and that a large number of differentially expressed genes exist during the differentiation of these two types of adipocytes.Citation24,Citation25 Different adipocytes also differ in the ability to store and release lipids and in their functional properties, predicting differences in different adipocytes at the molecular level.Citation26 Therefore, in the present study, the effect of DHCR7 on goat adipocytes and the underlying mechanisms was investigated, and the results provided meaningful data for exploring the role of DHCR7 in goat preadipocyte differentiation and for improving meat quality and human health.
Materials and methods
Sampling and cell culture
All experimental procedures including animals were approved by the Institutional Animal Care and Use Committee of Southwest Minzu University (Chengdu, Sichuan, China), and all experiments conformed to the requirements of the Chinese Catalogue for the Ethical Treatment of Laboratory Animals. 7-day-old male Jianzhou Daer goats (Capra hircus) (n = 3) were purchased from Sichuan Tiandi Goat Biological Engineering Co. Tissues of heart, liver, spleen, lung, kidney, subcutaneous fat, perirenal fat and longest dorsal muscle were collected rapidly after morning slaughter and stored in liquid nitrogen. Goat subcutaneous and intramuscular preadipocytes were isolated and cultured in accordance with previously described methods,Citation27,Citation28 respectively.
Gene clone and bioinformatics analysis
The total RNA was extracted from the heart, liver, spleen, lung, kidney, fat, and muscle tissues of Jianzhou Daer goats according to the instructions of the RNAiso Plus (Takara, Dalian, China) and the RNA integrity was examined via 1% agarose gel electrophoresis. Total RNA concentration and quality were determined by UV spectrophotometer, and the first strand of cDNA was synthesized by the RevertAid First Strand cDNA Synthesis Kit (Thermo, Waltham, MA, USA). The predicted sequence of goat DHCR7 (accession number: XM_013976064.2) was found in GenBank database in NCBI ((http://www.ncbi.nlm.nih.gov/genbank/ (accessed on 4 January 2022)). Primer Premier 5.0 software was used to design a pair of specific primers with a predicted length of 1,546 bp, and the primers were as follows:
F: 5′-CGCTTGGAAAGAGGTTGGAG-3′
R: 5′-CATTCAACAGGTGAGCAGAGG-3′
Sequence obtained from sequencing were compared with the predicted sequences on NCBI using DNAMAN. The cDNA sequence was predicted by ORF Finder (NCBI) for open reading frames and translated into amino acid sequences. Bioinformatics analysis of the CDS of the goat DHCR7 gene was performed, and the details of the analysis and tools were listed in Table S1.
Vector construction and siRNAs synthesis
The sequence of the CDS region of goat DHCR7 (GenBank accession: ON979535) was amplified using RT-PCR, digested with XhoI and EcoRI (Takara, Dalian, China) and ligated into the pEGFP-N1 (named as NC) vector by T4 DNA ligase (Takara, Dalian, China), and was named as pEGFP-DHCR7 (named as OE-DHCR7). The recombinant plasmid was identified by enzyme digestion and sequencing. Plasmid extraction was performed using the Mini Plasmid Extraction Kit (TIANGEN, Beijing, China) and stored at 4 °C for short-term use. For the RNA interference experiments, siRNA targeting DHCR7 (named as Si-DHCR7) and the negative control Si-RNA (named as Si-NC) were designed and synthesized by GenePharma (Shanghai, China). The sequence of Si-RNAs were as follows:
Si-DHCR7: 5′-GGAAGUGGUUUGACUUUCAATT-3′
Si-NC: 5′-UUCUCCGAACGUGUCACGUTT-3′
Cell transfection and induction of adipogenic differentiation
The subcutaneous and intramuscular preadipocytes of goat were cultured in DMEM/F12 (Hyclone, Logan, UT, USA) containing 10% FBS (Gemini, Calabasas, CA, USA) and 0.1% penicillin/streptomycin (P/S). The goat adipocytes passaged to the third generation were inoculated at 4 × 104 cells/well in 12-well plates. All transfection experiments were performed by TurboFect Transfection Reagent (Thermo, Waltham, MA, USA) at 70–80% preadipocytes confluence. The preadipocytes were induced by induction medium (IM), containing 10% FBS, 0.1% P/S, and 50 µM of oleic acid (Sigma, Tokyo, Japan) 18 h post transfection. After 48 h of cell induction, the cells were collected for subsequent experiments.
Oil red O staining and Bodipy staining
Cultured cells were washed with PBS and fixed in 4% formaldehyde at room temperature for 15 min. Then the cells were incubated using the oil red O working solution containing 6 ml oil red O stock solution (5 g/L in isopropanol) and 4 ml ddH2O for 30 min and washed twice with PBS. The Bodipy staining was performed according to the method described by Cai et al.Citation29 Briefly, the cells were stained using Bodipy (Thermo, Waltham, MA, USA) solution (away from light) for 20 min and then washed twice with PBS. The lipid droplets were observed and photographed under an Olympus IX-73 epifluorescence microscope (Tokyo, Japan) after staining and washing. Oil red O dye was extracted from stained adipocytes with 100% isopropanol, and the oil red O signal was quantified by measuring the optical density at 490 nm (OD 490).
RNA extraction and quantification
Total RNA was extracted from cells using RNAiso Plus (Takara, Dalian, China) and cDNA was reverse transcribed using the reverse transcriptase kit (Thermo, Waltham, MA, USA). The cDNA was diluted 5-fold for quantitative real-time PCR (qPCR) detection and the latter was carried out with a Bio-Rad CFX96 PCR System (Hercules, CA, USA) using SYBR Green Master Mix (Takara, Dalian, China) together with gene-specific primers. The 2−ΔΔCt method was used to analyze the mRNA relative expression level of each gene, and the UXT gene was used as the internal control.Citation30,Citation31 The information of qPCR primers was shown in .
Table 1. Primer information for quantitative real-time PCR (qPCR).
Western blot
Cells were harvested and lysed in RIPA buffer on ice (Biosharp, Beijing, China) containing 1% PMSF (Biosharp, Beijing, China). Protein concentration was measured with a BCA Protein Assay Kit (Biosharp, Beijing, China) according to the manufacturer’s instructions. Total protein (30 μg) of each sample was separated by 12% SDS–PAGE and transferred to a PVDF membrane (Millipore, Billerica, MA, USA) for immunoblot analysis. The following primary antibodies were used: anti-C/EBPα (1:1000 dilution; Cell Signaling Technology, America; 8178S), anti-PPARγ (1:1000 dilution; Cell Signaling Technology, America; 2435S), anti-C/EBPβ (1:1000 dilution; Abcam, England; ab32358) and anti-β-actin (1:5000 dilution; Abways, Shanghai, China; AB0035) and were incubated for 12 h at 4 °C. After washing by TBST buffer for 3 times, 15 min each time, the horse radish peroxidase-conjugated goat anti-rabbit IgG secondary antibody (1:5000 dilution; Abways, Shanghai, China; AB0102) was used and incubated for 2 h at room temperature. The PVDF membranes were washed by TBST buffer for 3 times, 15 min each time, and the immunoreactive signals were visualized by an ECL kit (BioRad, Hercules, CA, USA). Protein expression relative to β-actin was assessed by Image J2 software densitometric analysis.
Statistical analysis
Statistical analysis was performed by SPSS 25.0 (SPSS Inc., Chicago, IL, USA). All experiments were independently performed in triplicate. Comparisons were made by unpaired two-tailed Student’s t-test. All experimental data was presented as means ± SEM. *p < 0.05 was considered as statistically significant, and **p < 0.01 was considered as extremely significant. The images were generated by GraphPad Prism 8.0 (San Diego, CA, USA) software.
Results
Cloning and bioinformatic analysis of the DHCR7 gene in goats
The full length of the DHCR7 gene (1546 bp) was consisted of a 62 bp-long 5′ UTR, a 107 bp-long 3′ UTR, and a 1428 bp-long coding sequence (CDS), which encodes 475 amino acids (Fig. S1). The GenBank accession number of the goat DHCR7 gene was obtained from the NCBI database as ON979535. Similarity analysis of the CDS region of the DHCR7 gene in several species using NCBI Blast showed that the DHCR7 CDS region of the goat was similar to that of sheep, cattle, horse deer, killer whale, wild boar, camel, horse, mus musculus and homo sapiens with the following similarities: 98.81, 95.94, 95.03, 91.38, 90.53, 90.25, 82.98 and 70.66%, respectively (). The genetic similarity was high among different species and highly conserved during evolution. A phylogenetic tree of the DHCR7 gene was constructed using MEGA5.0 software, with goats being the most closely related to sheep, followed by other mammals such as cattle and deer (). These results suggested that the function of the DHCR7 gene was relatively conservative among species. Protein interacting analysis using the STRING database showed proteins such as DHCR24, SC5D, LSS, NSDHL, CH25H, LIPA and STS may have interactions with the DHCR7 protein (), among which DHCR24 could synergistically control the activity of DHCR7.Citation32 Lysosomal acid lipase (LAL), encoded by the LIPA gene, is a key regulator of cellular lipid homeostasis, catalyzing the hydrolysis of cholesteryl esters and triglycerides.Citation33 Therefore, we speculated that these proteins may indirectly influence the role of DHCR7 in adipocytes. The prediction results of basic physicochemical properties of goat DHCR7 protein were shown in Table S2. The amino acid composition showed that leucine (Leu) was the most abundant amino acid, accounting for 12.6% (Fig. S2A). The phosphorylation site prediction showed that there were 29 potential phosphorylation sites for the DHCR7 protein, including 12 serine (Ser) phosphorylation sites (red), 11 threonine (Thr) phosphorylation sites (green), and 6 tyrosine (Tyr) phosphorylation sites (blue) ( and SCitation2B). Phosphorylation is a chemical modification of proteins after biosynthesis, which is involved in various cellular activities such as cell growth, differentiation and apoptosis.Citation34 It is speculated that these sites may contribute to the occurrence of phosphorylation of DHCR7 protein and may be important sites for regulating the function of DHCR7 protein in goats. The glycosylation site prediction results showed that DHCR7 contains five O-glycosylation sites and two N-glycosylation sites (Fig. S1). In addition, DHCR7 protein was predicted to be a transmembrane hydrophobic protein (Figs. S2C and S2D), and the predicted secondary and tertiary structure of DHCR7 protein in goats were shown in Fig. S3.
Figure 1. The alignment analysis, phylogenetic tree construction and protein interaction analysis of DHCR7. (A) The nucleotide sequence identity analysis for DHCR7 gene among goats and other mammalian species. (B) The construction of DHCR7 nucleotide sequence phylogenetic tree. (C) Protein interaction analysis of DHCR7 protein in goats.
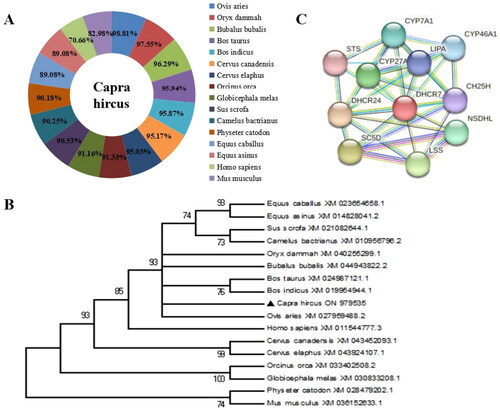
Figure 2. The DHCR7 gene expression patterns in various tissues, and in the process of subcutaneous preadipocyte and intramuscular preadipocyte differentiation. (A) The relative expression of DHCR7 in heart, liver, spleen, lung, kidney, longissimus dorsi muscle, subcutaneous fat and perirenal fat (n = 6). (B) The DHCR7 mRNA level on days 0–5 in induced differentiation of subcutaneous adipocyte (n = 6). (C) The DHCR7 mRNA level on days 0–5 in induced differentiation of intramuscular adipocyte (n = 6). data were shown as the means ± standard error of the mean (SEM). Different majuscule represented extremely significant difference (p < 0.01), **indicated extremely significant difference (p < 0.01).
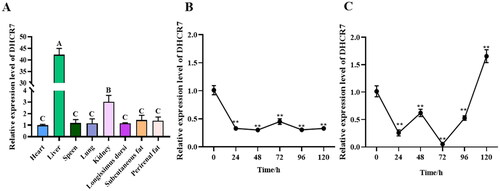
The expression patterns of DHCR7 gene in various tissue of goat and in the process of adipogenesis
Subsequently, the mRNA levels of DHCR7 were examined in goat tissues, including heart, liver, spleen, lung, kidney, longest dorsal muscle, subcutaneous fat, and perirenal fat. The results showed that DHCR7 was widely expressed in goat and highly expressed in liver tissue, which was extremely significant (p < 0.01) compared with other tissue samples in the experiment (). During subcutaneous fat formation, the mRNA levels of the DHCR7 gene generally tended to decrease before remaining stable (). In addition, the mRNA levels of DHCR7 gene showed a general trend of first falling and then rising during lipogenic differentiation of intramuscular adipocytes, during which, a small rise was observed at 48 h of lipogenic induction (). In summary, these results suggested that DHCR7 may play different roles in the process of subcutaneous fat formation and intramuscular adipocytes differentiation in goats.
DHCR7 inhibits the differentiation of subcutaneous preadipocytes in goats
To elucidate the exact role of DHCR7 in goat adipocytes adipogenesis, OE-DHCR7 and NC plasmids were transfected into primary cultured subcutaneous preadipocytes, respectively, and the function of DHCR7 was obtained by lipogenesis induction medium. The expression level of DHCR7 in the overexpressed group was significantly upregulated compared to the control group (). Furthermore, differentiated adipocytes transfected with OE-DHCR7 plasmid were treated with oil red O and Bodipy staining 2 days after induction, and a decrease in the number of lipid droplets within adipocytes was observed (). The oil red O signal in the overexpression group was significantly weaker than that in the control group (p < 0.01), indicated by the OD value at 490 nm (). The mRNA level of the adipocyte differentiation marker genes was detected by qPCR, and the results showed that except for PPARγ, the overexpression of DHCR7 significantly downregulated the expression of the adipose differentiation marker genes (p < 0.01) (). At the same time, the protein expression levels of C/EBPα, C/EBBβ, and PPARγ were verified by western blot, and the result showed that the content of C/EBPα and C/EBBβ protein in the overexpressed group was lower than that in the control group (). The expression levels of lipid metabolism-related genes including FASN, AGPAT6, ACC, ADRP, DGAT1, SCD1 and TIP47 were extremely significantly downregulated (p < 0.01), and the expression level of LPL was significantly downregulated (p < 0.05) (), indicating that overexpression of DHCR7 inhibited the differentiation of subcutaneous preadipocytes to form mature adipocytes.
Figure 3. The effect of DHCR7 overexpression on goat subcutaneous adipocyte differentiation. (A) The overexpression efficiency of DHCR7 in goat subcutaneous preadipocytes in NC and OE-DHCR7 groups 48 h post transfection (n = 6), UXT was the internal reference gene to normalize the expression levels. (B) Representative images (200×; scale bar = 50 μm; 400×; scale bar = 20 μm) of oil red O staining of goat subcutaneous preadipocytes. (C) representative images (200×; scale bar = 50 μm; 400×; scale bar = 20 μm) of mature adipocytes stained with Bodipy. (D) Semi-quantitative assessment of oil red O content absorbance detection at 490 nm in NC and OE-DHCR7 groups 48 h post transfection (n = 6). (E) The mRNA level of the adipocyte differentiation marker genes in NC and OE-DHCR7 groups 48 h post transfection (n = 6), UXT was the internal reference gene to normalize the expression levels. (F) Protein expression of C/EBPα, C/EBPβ, and PPARγ in NC and OE-DHCR7 groups 48 h post transfection. β-actin: internal reference gene. (G) Protein expression relative to β-actin was assessed by densitometric analysis. (H) Triglyceride metabolism enzymes in the NC and OE-DHCR7 groups 48 h post transfection (n = 6), UXT was the internal reference gene to normalize the expression levels. Data were shown as means ± SEM. *Indicates p values <0.05, **indicates p values <0.01.
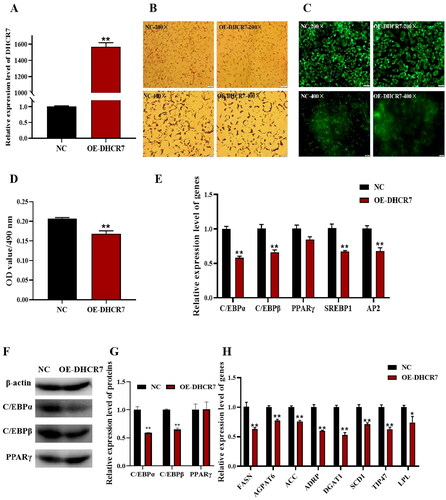
The effect of DHCR7 on adipocyte differentiation of subcutaneous preadipocytes in goats was further verified by interfering with DHCR7 using siRNA. Si-NC and Si-DHCR7 were transfected into goat subcutaneous preadipocytes to silence DHCR7 expression. DHCR7 interference efficiency was detected by qPCR, and Si-DHCR7 showed greater than 70% interference efficiency compared to the Si-NC group 48h post induction (). An increase in the degree of intracellular lipid accumulation was observed using oil red O staining and Bodipy fluorescence staining, and also an increase in the number of lipid droplets (). The oil red O signal was significantly stronger in the Si-DHCR7 group than that in the control group (p < 0.01), detected by the OD value at 490 nm (). The mRNA levels of adipocyte differentiation marker genes were detected by qPCR. The results showed that the expression of C/EBPβ, PPARγ, SREBP1, and AP2 was significantly higher than that in the control group (p < 0.01), and interfered DHCR7 expression significantly up regulated the expression of fat differentiation marker genes (). The expression levels of lipid metabolism-related genes including LPL, AGPAT6, GLUT4 and FASN were significantly downregulated (p < 0.05), and the expression levels of HSL, ATGL and SCD1 were extremely significantly downregulated (p < 0.01) (). These results suggested that the interference with DHCR7 promoted subcutaneous preadipocytes differentiation into mature adipocytes.
Figure 4. The effect of DHCR7 interference on goat subcutaneous adipocyte differentiation. (A) The interference efficiency of DHCR7 in goat subcutaneous preadipocytes in NC and Si-DHCR7 groups 48 h post transfection (n = 6), UXT was the internal reference gene to normalize the expression levels. (B) Representative images (200×; scale bar = 50 μm; 400×; scale bar = 20 μm) of oil red O staining of goat subcutaneous preadipocytes. (C) Representative images (200×; scale bar = 50 μm; 400×; scale bar = 20 μm) of mature adipocytes stained with Bodipy. (D) Semi-quantitative assessment of oil red O content absorbance detected at 490 nm in NC and Si-DHCR7 groups 48 h post transfection (n = 6). (E) The mRNA level of the adipocyte differentiation marker genes in NC and OE-DHCR7 groups 48 h post transfection (n = 6), UXT was the internal reference gene to normalize the expression levels. (F) Triglyceride metabolism enzymes in the NC and Si-DHCR7 groups 48 h post transfection (n = 6), UXT was the internal reference gene to normalize the expression levels. Data were shown as means ± SEM. *Indicates p values <0.05, **indicates p values <0.01.
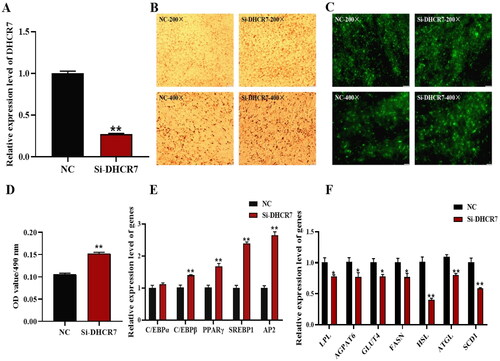
DHCR7 promotes the differentiation of goat intramuscular preadipocytes
To investigate the role of DHCR7 in regulating intramuscular preadipocyte differentiation in goats, similarly, the OE-DHCR7 and NC, Si-DHCR7 and Si-NC were transfected into intramuscular preadipocytes, respectively. The overexpression efficiency of DHCR7 in goat intramuscular preadipocytes was detected by qPCR, and the efficiency achieved a 38-fold increase compared to the control (p < 0.01) (). Furthermore, differentiated adipocytes transfected with OE-DHCR7 plasmid were treated with oil red O and Bodipy staining 2 days after induction, and an increase not only in the degree of intracellular lipid accumulation, but also in the number of lipid droplets was observed (). The oil red O signal in the overexpressed group was significantly stronger than that in the control group (p < 0.01), indicated by the OD value at 490 nm (). The adipogenesis process involves the differentiation of preadipocytes into mature adipocytes and lipid accumulation in mature adipocytes, a process regulated by lipogenic marker genes and genes associated with triglyceride synthesis (lipid metabolism). Therefore, the mRNA levels of these genes were detected by qPCR. The results showed that there was a significant change in the expression level of marker genes, including C/EBPα (p < 0.05), C/EBPβ (p < 0.01), PPARγ (p < 0.01) and SREBP1 (p < 0.05) compared with the control group (). At the same time, the expression levels of C/EBPα, C/EBPβ and PPARγ proteins were verified by western blot, and the contents of C/EBPβ and PPARγ proteins in the overexpressed group were higher than those in the control group (). The mRNA expression levels of GPAM, DGAT1, SCD and LPL were significantly upregulated (p < 0.05), and the expression levels of AGPAT6 were extremely significantly upregulated (p < 0.01). The expression levels of FASN were significantly downregulated (p < 0.05), and the expression levels of ACC, ATGL and ADRP were extremely significantly downregulated (p < 0.01) (). These results suggested that overexpression of DHCR7 promoted intramuscular preadipocytes differentiation to form mature adipocytes.
Figure 5. The effect of DHCR7 overexpression on goat intramuscular adipocyte differentiation. (A) The overexpression efficiency of DHCR7 in goat intramuscular preadipocytes in NC and OE-DHCR7 groups 48 h post transfection (n = 6), UXT was the internal reference gene to normalize the expression levels. (B) Representative images (200×; scale bar = 50 μm; 400×; scale bar = 20 μm) of oil red O staining of goat subcutaneous preadipocytes. (C) Representative images (100×; scale bar = 100 μm; 200×; scale bar = 50 μm) of mature adipocytes stained with Bodipy. (D) Semi-quantitative assessment of oil red O content absorbance detection at 490 nm in NC and OE-DHCR7 groups 48 h post transfection (n = 6). (E) The mRNA level of the adipocyte differentiation marker genes in NC and OE-DHCR7 groups 48 h post transfection (n = 6), UXT was the internal reference gene to normalize the expression levels. (F) Protein expression of C/EBPα, C/EBPβ and PPARγ in NC and OE-DHCR7 groups 48 h post transfection. β-actin: internal reference gene. (G) Protein expression relative to β-actin was assessed by densitometric analysis. (H) Triglyceride metabolism enzymes in the NC and OE-DHCR7 groups 48 h post transfection (n = 6), UXT was the internal reference gene to normalize the expression levels. Data were shown as means ± SEM. *Indicates p values <0.05, **indicates p values <0.01.
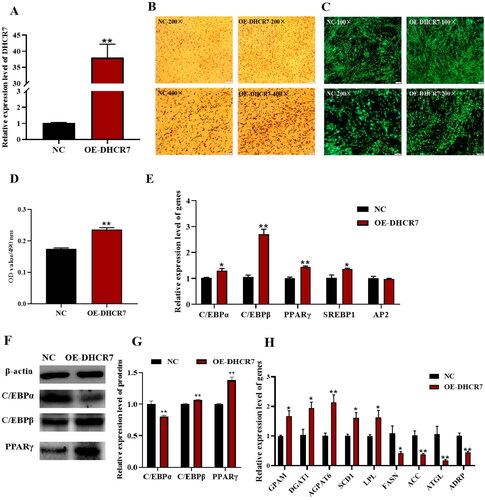
The effect of DHCR7 on adipocyte differentiation of intramuscular preadipocytes in goats was further verified by siRNA-mediated interfering experiment. Si-NC and Si-DHCR7 were transfected into goat intramuscular preadipocytes to silence DHCR7 expression, and the interference efficiency was detected by qPCR, and Si-DHCR7 showed greater than 75% interference efficiency compared to the Si-NC group 48 h after induction (). Oil red O staining and Bodipy staining showed that Si-DHCR7 promoted lipid content and the number of lipid droplets in goat intramuscular preadipocytes compared to the control (), which was consistent with morphological observations of adipocytes after overexpression of DHCR7. The qPCR results showed that Si-DHCR7 significantly promoted the expression of fat differentiation marker genes PPARγ and AP2 48 h after differentiation (). Western blot results showed an increase in protein expression of PPARγ after overexpression of DHCR7 (). The mRNA expression levels of FASN, AGPAT6, DGAT1, ATGL, TIP47 and GPAM were significantly increased (p < 0.01) (). Taken together, these data suggested that DHCR7 promoted the differentiation and lipid accumulation of goat intramuscular preadipocytes.
Figure 6. The effect of DHCR7 interference on goat intramuscular adipocyte differentiation. (A) The interference efficiency of DHCR7 in goat intramuscular preadipocytes in NC and Si-DHCR7 groups 48 h post transfection (n = 6), UXT was the internal reference gene to normalize the expression levels. (B) Representative images (200×; scale bar = 50 μm; 400×; scale bar = 20 μm) of oil red O staining of goat subcutaneous preadipocytes. (C) Representative images (200×; scale bar = 50 μm; 400×; scale bar = 20 μm) of mature adipocytes stained with Bodipy. (D) Semi-quantitative assessment of oil red O content absorbance detection at 490 nm in NC and Si-DHCR7 groups 48 h post transfection (n = 6). (E) The mRNA level of the adipocyte differentiation marker genes in NC and OE-DHCR7 groups 48 h post transfection (n = 6), UXT was the internal reference gene to normalize the expression levels. (F) Protein expression of C/EBPα, C/EBPβ and PPARγ in NC and Si-DHCR7 groups 48 h post transfection. β-actin: internal reference gene. (G) Protein expression relative to β-actin was assessed by densitometric analysis. (H) Triglyceride metabolism enzymes in the NC and Si-DHCR7 groups (n = 6), UXT was the internal reference gene to normalize the expression levels. Data were shown as means ± SEM. *Indicates p values <0.05, **indicates p values <0.01.
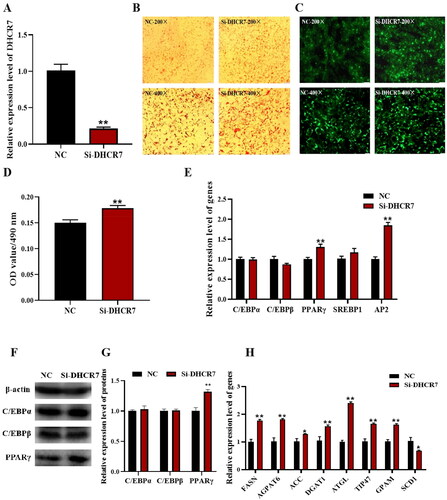
Comparison of the effects of DHCR7 on the subcutaneous and intramuscular preadipocytes differentiation in goat
To comprehensively compare and analyze the differences in the effects of DHCR7 on the differentiation of goat subcutaneous and intramuscular preadipocytes, Venny 2.1 program was used to cross the genes with significant changes in expression. DHCR7 overexpression inhibited the differentiation of goat subcutaneous adipocytes and accumulation of lipids, promoted the differentiation of goat intramuscular adipocytes and accumulation of lipids. There was intersection of the regulated marker genes and showed different expression trends, such as C/EBPα, C/EBPβ, AGPAT6, DGAT1 and SCD1 (). Differentiation and lipid accumulation were regulated in goat subcutaneous adipocytes by down-regulating AP2, FASN, ACC, et al. It was speculated that overexpressing DHCR7 inhibited the differentiation of goat intramuscular fat cells by promoting lipid decomposition and inhibiting lipid production. However, in the intramuscular adipocytes of goats, the expression of PPARγ and GPAM genes was up regulated. Therefore, it was speculated that overexpressing DHCR7 gene promoted the differentiation of goat intramuscular adipocytes by promoting lipid production. At the same time, PPARγ, AP2, AGPAT6, FASN and SCD1 genes were differently regulated in both subcutaneous and intramuscular adipocytes after silencing DHCR7 gene. However, the expression of C/EBPβ and SREBP1 were up regulated in subcutaneous fat cells, while ATGL, HSL and LPL were inhibited. ACC, DGAT1 and GPAM were downregulated in intramuscular fat cells ().
Figure 7. Overexpressing and silencing DHCR7 gene have discrepancy between subcutaneous adipocytes and intramuscular adipocytes. (A) Differentiation marker genes were differently regulated in intramuscular and subcutaneous adipocyte after overexpressing DHCR7. (B) Differentiation marker genes were differently regulated in intramuscular and subcutaneous adipocyte after silencing DHCR7.
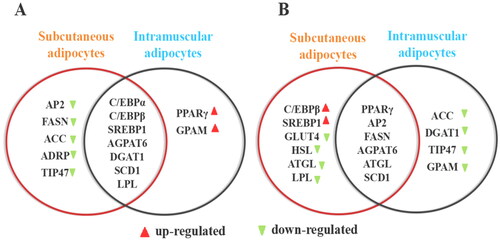
Discussion
Several research groups successfully cloned DHCR7 in humans and rodents and determined its chromosomal location.Citation35,Citation36 DHCR7 has been implicated in the human disease Smith-Lemli-Opitz syndrome (SLOS) and in the synthesis of endogenous cholesterol in mice,Citation17,Citation37 but the role of DHCR7 in animal fat metabolism and fat deposition has not been reported yet. In this study, the goat DHCR7 gene was cloned, and the expression patterns in various tissues and in the process of subcutaneous and intramuscular adipogenesis were revealed. Functionally, the exact role of DHCR7 in regulating goat SAT and IMF deposition in vitro was investigated systematically by loss and gain of function. Overall, this work elucidates the specific molecular mechanism of DHCR7 in regulating the differentiation of subcutaneous and intramuscular adipocytes and provides theoretical support for improving human health and meat quality.
Analysis of gene expression in different tissues is a way to study molecular regulation and provides important insights into the function of the gene. In this study, it was found that DHCR7 expresses widely in goat tissues, but the mRNA expression levels vary from tissue to tissue. It expressed highly in liver tissue, which is consistent with other species.Citation38,Citation39 The liver is an important metabolic organ of the animal body, Musso G et al.Citation40 linked altered hepatic cholesterol homeostasis to hypercholesterolemia and the pathogenesis of cardiovascular disease. DHCR7 is a key enzyme that catalyzes the conversion of 7-dehydrocholesterol to cholesterol.Citation11 Cholesterol can form bile acids in liver cells, these metabolites also play important biological roles either as signal transducers or as solubilisers of other lipids.Citation18,Citation41 Thus, the highest mRNA level in the liver was observed among sample tissues. Meanwhile, the kidney is regarded as one of the important and high-energy demanding organs of the body which ensures normal metabolism of the organism by removing the poisons and wastes in the body,Citation42 the DHCR7 gene also has a high expression level in the kidney. The widespread expression of the DHCR7 gene showed that it plays an indispensable role in maintaining the vital activities and organisms’ metabolism. To further investigate the biological role of DHCR7 gene in goat adipocytes, the expression trend of DHCR7 during the formation of subcutaneous and intramuscular precursor fat in goats was measured, and the results showed different expression patterns in between. The low expression of DHCR7 during the process of subcutaneous adipocytes formation in goats was speculated that it may negatively regulate subcutaneous fat differentiation, and the results of functional studies in the following verification experiments also confirmed this assumption. The expression trend of DHCR7 during intramuscular adipogenesis was speculated that DHCR7 may dynamically regulate intramuscular adipogenesis, and the effect of DHCR7 on intramuscular adipogenesis was studied through overexpression and interference. Other studies have shown that adipocytes isolated from subcutaneous fat or intramuscular fat tissues display distinct patterns in differentiation and lipid metabolism.Citation43 It is suggested that the DHCR7 gene may involve in different regulatory patterns in two different types of preadipocytes.
Adipocytes differentiation is a key process in adipogenesis and is necessary for preadipocytes to differentiate into mature adipocytes. Preadipocytes differentiation is a complicated biological process accompanied by the change of cell morphological structure and function.Citation44 In order to reveal the regulatory effect of DHCR7 on the differentiation of different adipocytes, DHCR7 was overexpressed by cell transfection, and the data showed that overexpression of DHCR7 inhibited goat subcutaneous preadipocytes differentiation, manifested by reduction of bodipy and oil red O signal, and a decreased expression levels of adipocyte differentiation marker genes and TG synthesis-related genes. In mammalian cells, PPARγ, C/EBPα, and C/EBPβ are major regulators of adipogenesis.Citation1,Citation29,Citation45 In addition, AP2 and SREBP1 positively regulate transport, and has an increasing protein expression during adipogenesis and is recognized as a marker of mature adipocytes.Citation46 SREBP1 is also a key transcription factor in adipocyte differentiation and plays a crucial role in lipid metabolism, involved in the synthesis and metabolism of cholesterol, triglycerides and fatty acids.Citation47 Therefore, the above factors are used as criteria for judging adipocyte differentiation. Eventual, it was found that DHCR7 affects cell differentiation by regulating the expression of C/EBPα, C/EBPβ and PPARγ, as well as SREBP1 and AP2 genes in goat subcutaneous adipocytes. In addition, the protein expression levels of C/EBPα and C/EBPβ are down regulated after DHCR7 overexpression, which was consistent with the downregulation of their mRNA levels, indicating that DHCR7 hinders goat subcutaneous adipocytes differentiation by inhibiting the expression of C/EBPα and C/EBPβ. It is known that fat deposition was mainly determined by the hyperplasia and hypertrophy of adipocytes, the latter was associated with triglycerides (TG) biogenesis and its lipolysis,Citation48 which was associated with processes of de novo biogenesis and transfer of fatty acids, as the dominant function and the regulation of it was controlled by a number of enzymes. After overexpression of DHCR7, the mRNA levels of TG biogenic-related genes (DGAT1, AGPAT6, and SCD1) were significantly decreased and TG lipolytic-related genes (ADRP, FASN, and ACC) were significantly down-regulated in subcutaneous adipocytes. AGPAT6 and DGAT1 are the rate-limiting enzymes during triglyceride synthesis.Citation49,Citation50 DGAT1 forms triglycerides by catalyzing the ligation of diacylglycerol and esteryl-CoA,Citation51 overexpression of DGAT1 significantly increased the content of mouse adipose tissue and skeletal muscle TAG, and promoted the deposition of IMF in skeletal muscle.Citation52 Stearoyl coenzyme A desaturase1 (SCD1) is an enzyme involved in monounsaturated fatty acid biosynthesis. The contents of triacylglycerol and cholesterol were significantly decreased as a consequence of SCD1 knockout in goat mammary epithelial cells.Citation53 In addition, other protein-related lipid droplets, such as PAT family proteins (ADRP), are embedded on the surface of intracellular fat droplets and regulate lipid droplet and lipid production, transport, aggregation, and lipolysis.Citation54 Both FASN and ACC are key enzymes in fatty acid synthesis,Citation55 it is hypothesized that the decrease in lipid accumulation after overexpression of DHCR7 is due to the weakening of fatty acid synthesis. Evidence suggests that TMEM147 interacts with DHCR7 to affect cellular cholesterol homeostasis and increases the extracellular level of 27-hydroxycholesterol in hepatocellular carcinoma cells, and increased lipid metabolism in macrophages.Citation56 Moreover, knockdown of DHCR7 promoted the lipid accumulation and enhanced adipogenic biogenic-related genes, and the opposite result was achieved at the morphological and molecular levels in the DHCR7 knockdown group (), which further confirmed the inhibitory effect of DHCR7 on subcutaneous adipocyte differentiation and lipid deposition.
Different adipocytes differ in the ability to store and release lipids and functional characteristics, indicating there are differences among different adipocytes at the molecular level.Citation26 In this work, we proved that DHCR7 as a negative regulator for goat subcutaneous preadipocytes adipogenesis, but as a positive regulator for goat intramuscular preadipocytes adipogenesis. By overexpressing and interfering with DHCR7, the role of DHCR7 in goat intramuscular adipocytes was investigated. Interestingly, after overexpression of DHCR7, the number of lipid droplets in intramuscular adipocytes was increased, lipid deposition was promoted. Specifically, fat differentiation marker genes C/EBPα, C/EBPβ, PPARγ and SREBP1 were significantly up regulated, the protein expression levels of C/EBPβ and PPARγ were up regulated, which was consistent with the upregulation of their mRNA levels. However, the protein expression levels of C/EBPα was down regulated, studies have found that C/EBPβ can activate the expression of C/EBPα and PPARγ genes during adipocyte differentiation.Citation57 The protein expression level of C/EBPα was inconsistent with mRNA level when overexpressed goat DHCR7, possibly for C/EBPα, C/EBPβ and PPARγ, and a negative feedback mechanism among the three. That is, the high protein levels of C/EBPβ and PPARγ inhibited the expression of C/EBPα protein. Lipid metabolism genes GPAM, DGAT1, AGPAT6, LPL and SCD1 were up regulated, which was consistent with increasement of oil red O signal. In other studies, DHCR7 overexpression was found to increase the cholesterol level in gastric cancer cells.Citation58 At the same time, the possible role of DHCR7 in intramuscular preadipocytes was studied through the loss of function (). However, we found that some genes were expressed in the same way in different experimental conditions (DHCR7 overexpression and inhibition). Ideally, overexpression and interference of genes would show different expression tendencies in downstream genes, but we found that some genes exhibit the same expression tendencies in upstream molecular overexpression and interfering cells, possibly because the gene expression within the cell is regulated by multiple factors and genes, and the signal regulatory network within the cell is very complex. Overall, DHCR7 promoted intramuscular adipocyte differentiation and lipid droplet accumulation by altering the expression of adipocyte differentiation, TG synthesis and metabolism marker genes, which differed from the role played by DHCR7 in subcutaneous adipocyte. There are studies also indicated that the expression of some genes involved in lipolysis and lipogenesis were different among various adipose depots. For example, silencing KLF9 gene promoted lipid accumulation in intramuscular preadipocytes of goats, however, the opposite results were obtained from 3T3-L1 adipocytes.Citation31,Citation59 Therefore, this study determined the role of DHCR7 in adipocyte differentiation and fat deposition and provided meaningful data for further elucidating the specific mechanism of DHCR7 in adipocyte differentiation and fat deposition in different parts of goat.
Conclusions
In summary, the DHCR7 gene in goats was highly conserved among species, and DHCR7 was widely expressed in most tissues of goat and was highly expressed in the liver. In addition, this study proved that DHCR7 can inhibit subcutaneous adipocyte differentiation and promote intramuscular adipocyte differentiation, which is related to the expression changes of adipocyte differentiation marker genes and lipid metabolism related genes such as C/EBPβ, PPARγ, AGPAT6, DGAT1, SCD1, et al. These results suggested that DHCR7 is a new candidate gene for goat adipogenic differentiation, which provided important data for further study of the underlying molecular mechanism of goat DHCR7 gene in the regulation of goat adipocyte differentiation and fat deposition.
Author contributions
Zhibin Li, Tingting Hu, Ruiwen Li and Jinlan Li looked for information. Zhibin Li, Jiani Xing and Youli Wang conceived and designed the experiment. Zhibin Li, Tingting Hu and Jinlan Li completed the experimental content. Zhibin Li, Yaqiu Lin and Youli Wang organizeand analyzed the experiment data. Zhibin Li and Youli Wang wrote the article. Yong Wang, Yanyan Li and Jiani Xing revised the article. All authors read and approved the final version of the manuscript.
Supplemental Material
Download MS Word (759.2 KB)Disclosure statement
No potential conflict of interest was reported by the author(s).
Additional information
Funding
References
- Mota de Sá P, Richard AJ, Hang H, et al. Transcriptional regulation of adipogenesis. Compr Physiol. 2017;7(2):635–674.
- Sarantopoulos CN, Banyard DA, Ziegler ME, et al. Elucidating the preadipocyte and its role in adipocyte formation: a comprehensive review. Stem Cell Rev Rep. 2018;14(1):27–42.
- Wei S, Zhang M, Zheng Y, et al. ZBTB16 Overexpression enhances white adipogenesis and induces brown-like adipocyte formation of bovine white intramuscular preadipocytes. Cell Physiol Biochem. 2018;48(6):2528–2538.
- Liu R, Liu X, Bai X, et al. A study of the regulatory mechanism of the CB1/PPARγ2/PLIN1/HSL pathway for fat metabolism in cattle. Front Genet. 2021;12:631187.
- Wang Y, Zhang Y, Su X, et al. Cooperative and independent functions of the miR-23a∼27a∼24-2 cluster in bovine adipocyte adipogenesis. Int J Mol Sci. 2018;19(12):3957.
- Stewart SM, Gardner GE, McGilchrist P, et al. Prediction of consumer palatability in beef using visual marbling scores and chemical intramuscular fat percentage. Meat Sci. 2021;181:108322.
- Wang L, Shan T. Factors inducing transdifferentiation of myoblasts into adipocytes. J Cell Physiol. 2021;236(4):2276–2289.
- Perna M, Hewlings S. Saturated fatty acid chain length and risk of cardiovascular disease: a systematic review. Nutrients. 2022;15(1):30.
- Sakata Y, Shimokawa H. Saturated fatty acid intake and cardiovascular risk. Eur Heart J. 2013;34(16):1178–1180.
- Queiroz JC, Alonso-Vale MI, Curi R, et al. Control of adipogenesis by fatty acids. Arq Bras Endocrinol Metabol. 2009;53(5):582–594.
- Prabhu AV, Luu W, Li D, et al. DHCR7: a vital enzyme switch between cholesterol and vitamin D production. Prog Lipid Res. 2016;64:138–151.
- Du Y, Wang Y, Xu Q, et al. TMT-based quantitative proteomics analysis reveals the key proteins related with the differentiation process of goat intramuscular adipocytes. BMC Genomics. 2021;22(1):417.
- Cooper MK, Wassif CA, Krakowiak PA, et al. A defective response to Hedgehog signaling in disorders of cholesterol biosynthesis. Nat Genet. 2003;33(4):508–513.
- Bijlsma MF, Spek CA, Zivkovic D, et al. Repression of smoothened by patched-dependent (pro-)vitamin D3 secretion. PLOS Biol. 2006;4(8):e232.
- Blassberg R, Macrae JI, Briscoe J, et al. Reduced cholesterol levels impair smoothened activation in Smith-Lemli-Opitz syndrome. Hum Mol Genet. 2016;25(4):693–705.
- Lauth M, Rohnalter V, Bergström A, et al. Antipsychotic drugs regulate hedgehog signaling by modulation of 7-dehydrocholesterol reductase levels. Mol Pharmacol. 2010;78(3):486–496.
- Fitzky BU, Moebius FF, Asaoka H, et al. 7-Dehydrocholesterol-dependent proteolysis of HMG-CoA reductase suppresses sterol biosynthesis in a mouse model of Smith-Lemli-Opitz/RSH syndrome. J Clin Invest. 2001;108(6):905–915.
- Le Lay S, Ferré P, Dugail I. Adipocyte cholesterol balance in obesity. Biochem Soc Trans. 2004;32(Pt 1):103–106.
- Briand N, Prado C, Mabilleau G, et al. Caveolin-1 expression and cavin stability regulate caveolae dynamics in adipocyte lipid store fluctuation. Diabetes. 2014;63(12):4032–4044.
- Sun S, Adyshev D, Dudek S, et al. Cholesterol-dependent modulation of stem cell biomechanics: application to adipogenesis. J Biomech Eng. 2019;141(8):0810051–08100510.
- Diep DTV, Hong K, Khun T, et al. Anti-adipogenic effects of KD025 (SLx-2119), a ROCK2-specific inhibitor, in 3T3-L1 cells. Sci Rep. 2018;8(1):2477.
- Sim CK, Kim SY, Brunmeir R, et al. Regulation of white and brown adipocyte differentiation by RhoGAP DLC1. PLOS One. 2017;12(3):e0174761.
- Zhang GH, Lu JX, Chen Y, et al. Comparison of the adipogenesis in intramuscular and subcutaneous adipocytes from Bamei and Landrace pigs. Biochem Cell Biol. 2014;92(4):259–267.
- Sun WX, Wang HH, Jiang BC, et al. Global comparison of gene expression between subcutaneous and intramuscular adipose tissue of mature Erhualian pig. Genet Mol Res. 2013;12(4):5085–5101.
- Sheng X, Ni H, Liu Y, et al. RNA-seq analysis of bovine intramuscular, subcutaneous and perirenal adipose tissues. Mol Biol Rep. 2014;41(3):1631–1637.
- Jo J, Shreif Z, Periwal V. Quantitative dynamics of adipose cells. Adipocyte. 2012;1(2):80–88.
- Li A, Li Y, Wang Y, et al. ACADL promotes the differentiation of goat intramuscular adipocytes. Animals. 2023;13(2):281.
- Chen D, Lin Y, Zhao N, et al. Hoxa5 inhibits the proliferation and induces adipogenic differentiation of subcutaneous preadipocytes in goats. Animals. 2022;12(14):1859.
- Cai R, Sun Y, Qimuge N, et al. Adiponectin AS lncRNA inhibits adipogenesis by transferring from nucleus to cytoplasm and attenuating adiponectin mRNA translation. Biochim Biophys Acta Mol Cell Biol Lipids. 2018;1863(4):420–432.
- Livak KJ, Schmittgen TD. Analysis of relative gene expression data using real-time quantitative PCR and the 2(-delta delta C(T)) method. Methods. 2001;25(4):402–408.
- Xu Q, Lin Y, Wang Y, et al. Knockdown of KLF9 promotes the differentiation of both intramuscular and subcutaneous preadipocytes in goat. Biosci Biotechnol Biochem. 2020;84(8):1594–1602.
- Luu W, Hart-Smith G, Sharpe LJ, et al. The terminal enzymes of cholesterol synthesis, DHCR24 and DHCR7, interact physically and functionally. J Lipid Res. 2015;56(4):888–897.
- Arnaboldi L, Ossoli A, Giorgio E, et al. LIPA gene mutations affect the composition of lipoproteins: Enrichment in ACAT-derived cholesteryl esters. Atherosclerosis. 2020;297:8–15.
- Humphrey SJ, James DE, Mann M. Protein phosphorylation: a major switch mechanism for metabolic regulation. Trends Endocrinol Metab. 2015;26(12):676–687.
- Fitzky BU, Witsch-Baumgartner M, Erdel M, et al. Mutations in the Delta7-sterol reductase gene in patients with the Smith-Lemli-Opitz syndrome. Proc Natl Acad Sci USA. 1998;95(14):8181–8186.
- Waterham HR, Wijburg FA, Hennekam RC, et al. Smith-Lemli-Opitz syndrome is caused by mutations in the 7-dehydrocholesterol reductase gene. Am J Hum Genet. 1998;63(2):329–338.
- Tint GS, Irons M, Elias ER, et al. Defective cholesterol biosynthesis associated with the Smith-Lemli-Opitz syndrome. N Engl J Med. 1994;330(2):107–113.
- Fagerberg L, Hallström BM, Oksvold P, et al. Analysis of the human tissue-specific expression by genome-wide integration of transcriptomics and antibody-based proteomics. Mol Cell Proteomics. 2014;13(2):397–406.
- Li M, Chen L, Tian S, et al. Comprehensive variation discovery and recovery of missing sequence in the pig genome using multiple de novo assemblies. Genome Res. 2017;27(5):865–874.
- Musso G, Gambino R, Cassader M. Cholesterol metabolism and the pathogenesis of non-alcoholic steatohepatitis. Prog Lipid Res. 2013;52(1):175–191.
- Hylemon PB, Zhou H, Pandak WM, et al. Bile acids as regulatory molecules. J Lipid Res. 2009;50(8):1509–1520.
- Han SH, Malaga-Dieguez L, Chinga F, et al. Deletion of Lkb1 in renal tubular epithelial cells leads to CKD by altering metabolism. J Am Soc Nephrol. 2016;27(2):439–453.
- Wang S, Zhou G, Shu G, et al. Glucose utilization, lipid metabolism and BMP-Smad signaling pathway of porcine intramuscular preadipocytes compared with subcutaneous preadipocytes. Cell Physiol Biochem. 2013;31(6):981–996.
- Rosen ED, Spiegelman BM. Molecular regulation of adipogenesis. Annu Rev Cell Dev Biol. 2000;16(1):145–171.
- Kudo M, Sugawara A, Uruno A, et al. Transcription suppression of peroxisome proliferator-activated receptor gamma2 gene expression by tumor necrosis factor alpha via an inhibition of CCAAT/enhancer-binding protein delta during the early stage of adipocyte differentiation. Endocrinology. 2004;145(11):4948–4956.
- Atlas E, Pope L, Wade MG, et al. Bisphenol A increases aP2 expression in 3T3L1 by enhancing the transcriptional activity of nuclear receptors at the promoter. Adipocyte. 2014;3(3):170–179.
- Jeon TI, Osborne TF. SREBPs: metabolic integrators in physiology and metabolism. Trends Endocrinol Metab. 2012;23(2):65–72.
- Haczeyni F, Bell-Anderson KS, Farrell GC. Causes and mechanisms of adipocyte enlargement and adipose expansion. Obes Rev. 2018;19(3):406–420.
- Harris CA, Haas JT, Streeper RS, et al. DGAT enzymes are required for triacylglycerol synthesis and lipid droplets in adipocytes. J Lipid Res. 2011;52(4):657–667.
- Shingfield KJ, Bonnet M, Scollan ND. Recent developments in altering the fatty acid composition of ruminant-derived foods. Animal. 2013;7(1):132–162.
- Yen CL, Stone SJ, Koliwad S, et al. Thematic review series: glycerolipids. DGAT enzymes and triacylglycerol biosynthesis. J Lipid Res. 2008;49(11):2283–2301.
- Roorda BD, Hesselink MK, Schaart G, et al. DGAT1 overexpression in muscle by in vivo DNA electroporation increases intramyocellular lipid content. J Lipid Res. 2005;46(2):230–236.
- Tian H, Luo J, Zhang Z, et al. CRISPR/Cas9-mediated stearoyl-CoA Desaturase 1 (SCD1) deficiency affects fatty acid metabolism in goat mammary epithelial cells. J Agric Food Chem. 2018;66(38):10041–10052.
- Fujimoto T, Ohsaki Y, Cheng J, et al. Lipid droplets: a classic organelle with new outfits. Histochem Cell Biol. 2008;130(2):263–279.
- Zappaterra M, Luise D, Zambonelli P, et al. Association study between backfat fatty acid composition and SNPs in candidate genes highlights the effect of FASN polymorphism in large white pigs. Meat Sci. 2019;156:75–84.
- Huang J, Pan H, Sun J, et al. TMEM147 aggravates the progression of HCC by modulating cholesterol homeostasis, suppressing ferroptosis, and promoting the M2 polarization of tumor-associated macrophages. J Exp Clin Cancer Res. 2023;42(1):286.
- Yan H, Li Q, Li M, et al. Ajuba functions as a co-activator of C/EBPβ to induce expression of PPARγ and C/EBPα during adipogenesis. Mol Cell Endocrinol. 2022;539:111485.
- Chen Y, Yan W, Yang K, et al. Integrated multi-dimensional analysis highlights DHCR7 mutations involving in cholesterol biosynthesis and contributing therapy of gastric cancer. J Exp Clin Cancer Res. 2023;42(1):36.
- Kimura H, Fujimori K. Activation of early phase of adipogenesis through Krüppel-like factor KLF9-mediated, enhanced expression of CCAAT/enhancer-binding protein β in 3T3-L1 cells. Gene. 2014;534(2):169–176.