Abstract
Cadmium (Cd) is one of the most toxic and widely distributed pollutants in the environment. Cadmium contamination of soils has posed a serious threat to safe food production in many parts of the world. The authors present a comprehensive review of present status of phytoextraction technology for cleaning up Cd-contaminated soils, based primarily on the data resulting from both laboratory and field-scale studies that have been conducted to assess or improve the Cd phytoextraction potential of various plant species in the past decade. The encouraging results of field-scale studies have provided a fundamental basis to usher phytoextraction technology into practical use to remediate slightly to moderately Cd-contaminated soils in Europe and Asia, although this technology is not yet ready for widespread application. Chelators and microorganisms tested so far seem not to contribute to the applicability of Cd phytoextraction. The major challenges for the large-scale application of Cd phytoextraction are (a) how to further improve the efficiency of Cd phytoextraction, (b) how to cut the overall costs of Cd phytoextraction, and (c) how to get greater stakeholders’ acceptance of Cd phytoextraction as a reliable option.
1. INTRODUCTION
Cadmium (Cd) is a rare metallic element and its natural occurrence in the environment is always low (< 1 mg kg−1; World Health Organization [WHO], Citation1992). High levels of Cd in soils mostly result from anthropogenic activities (McLaughlin et al., Citation1999; WHO, Citation1992). Major sources are mining, smelting, metal plating, waste disposal, and the application of phosphate fertilizers (McLaughlin et al., Citation1999; WHO, Citation1992). In the past decades, Cd contamination of soils has been extensively investigated (Chlopecka, Citation1996; Culbard et al., Citation1988; Pietz et al., Citation1978; Simmons et al., Citation2005). It has been reported that Cd concentrations in contaminated soils can be 1–3 orders of magnitude higher than in uncontaminated soils. For example, a study from Southeast Asia found that the maximum concentration of Cd in paddy soil samples collected from an actively Zn-mineralized area was as high as 284 mg kg−1, nearly 1900 times greater than the background soil Cd concentration of 0.15 mg kg−1 (Simmons et al., Citation2005).
Cadmium in soils is more readily bioavailable for plant uptake than other contaminant metals such as Cu, Mn, Ni, Pb, and Zn (McLaughlin et al., Citation1999; Prokop et al., Citation2003). Therefore, low Cd concentrations in soils may also result in a high Cd uptake by plants (Li et al., Citation2006). Although a biological function for Cd in diatoms has already been demonstrated (Lane and Morel, Citation2000), it is generally recognized that Cd is a nonessential trace element for plant growth. More exactly, Cd can be toxic to plants even at low concentrations. For example, it was reported that a soil Cd concentration as low as 2.5 mg kg−1 in (soil pH = 6.7) caused toxicity in wheat (Triticum aestivum) and soybean (Glycine max) (Haghiri, Citation1973). The Cd-induced primary physiological effects on plants include a reduction in photosynthesis, inhibition of N-metabolism, and a decrease in water and mineral uptake (Sanità di Toppi and Gabbrielli, Citation1999; Smeets et al., Citation2008).
Cadmium is also known to be harmful to human health. Chronic Cd exposure can give rise to various diseases (WHO, Citation1992). One of the most known is osteomalacia combined with renal dysfunction, known as Itai-Itai disease (Ikeda et al., Citation2004). Furthermore, Cd has been classified as a human carcinogen (U.S. Department of Health and Human Services, Citation2005). Human intake of Cd can be through food consumption, smoking, and occupational exposure (WHO, Citation1992). For most people, however, soil-crop-human is the most important exposure pathway. It follows that elevated concentrations of Cd in agricultural soils will greatly increase the risk of human exposure to Cd (McLaughlin et al., Citation1999). Numerous surveys have shown that the levels of Cd in some foods grown on soils with Cd contamination have always exceeded regulatory health limits (Demirezen and Aksoy, Citation2006; Lee et al., Citation1999; Watanabe et al., Citation1996; Williams et al., 2009). For example, in an extensive province-wide survey conducted in China, Williams et al. (2009) found that 65 % of all field rice from mine-impacted areas failed to meet the national food standard for Cd. This critical environmental issue has already led to mounting public concerns and therefore highlights an urgent need for a continuing effort to clean up Cd-contaminated soils.
There are a number of ways to reduce Cd contamination in soils. The most commonly used methods are excavation and landfill, soil incineration, soil washing and vitrification, leaching, and electroreclamation. These nonbiological remediation technologies in addition to being expensive are disruptive to the surrounding environment, although they tend to be efficient (Cunningham and Ow, Citation1996). As an emerging technology, Cd phytoextraction, the use of plants to remove Cd from contaminated soils, has attracted tremendous attention since the 1990s, due to its potential low cost, environmental sustainability, and effectiveness (Cunningham and Ow, Citation1996).
Research and application of phytoextraction technology for cleanup of Cd-contaminated soils over the past 10 years has provided much useful information that can be used to design effective remediation schemes and project further improvement. The aim of this article is to review the literature concerning phytoextraction of Cd-contaminated soils thereby giving a strong foundation for understanding the present status and future challenges of Cd phytoextraction. Here we focus on both laboratory and field-scale studies that have been conducted to assess or improve the Cd-phytoextraction potential of various plant species. Although a better understanding of the molecular mechanisms underlying Cd tolerance and accumulation in plants is considered as a promising long-term strategy to improve Cd phytoextraction, the fruitful progress in this aspect will not be discussed here, as it has been reviewed recently by others (Verbruggen et al., Citation2009; Zhao and McGrath, Citation2009).
2. CADMIUM ACCUMULATION IN FIELD-SAMPLED PLANT MATERIALS
Although the term phytoextraction is relatively new, the basic concept of phytoextraction is older (Raskin et al., Citation1997). It came originally from the discovery of unusual accumulation of Ni in field-collected plant samples (Baker et al., Citation1988; Brooks, Citation1998; Chaney, Citation1983). For Cd phytoextraction, many research groups around the world have therefore conducted field surveys in the past decade to screen potential Cd-accumulating plant species. These surveys have found that Cd concentrations in field-sampled plant materials vary widely. The shoot Cd observed in hyperaccumulators can be in the 1000 s mg kg−1 dry weight (DW; Assunção et al., Citation2003b; Baker et al., Citation2000), whereas those of nonaccumulators are frequently <10 mg kg−1 (Cui et al., Citation2007). Caution is needed in the interpretation of these data: (a) it is always somewhat uncertain whether all high shoot Cd observed in plants occurring on mining sites represent genuine Cd-accumulating capacities of the plant species owing to the fact that it is difficult to remove airborne Cd in dusts on plant surfaces (Baker et al., Citation2000); and (b) most importantly, the phytoextraction potentials of most Cd-accumulating plant species recognized through these field surveys have been assessed by means of pot or field experiments, which are discussed in the following sections.
3. CADMIUM REMOVAL BY POT-GROWN PLANT SPECIES
It is easy to understand why a pot experiment in a glasshouse is needed as a preliminary before putting a phytoextraction scheme into practice, although the results obtained under controlled laboratory conditions cannot be directly extrapolated to field conditions (Schmidt, Citation2003). Because many pot experiments have been conducted to assess or improve the Cd phytoextraction potential of various plants species, in this section we not only focus on the Cd removal capacity of different types of plant species, but also focus on the effects of major approaches that have been used to improve Cd phytoextraction. Unless otherwise stated, the Cd removal capacities (expressed as percentages on an annual basis) presented in this section have been calculated as follows based on the data available from literature: % Cd removal = (Cd concentration in shoot × shoot biomass)/(total Cd concentration in soil × soil weight) × 100.
It should be noted that the initial Cd concentration in soils is an important factor determining % Cd removal by plant species. In this article, comparisons were made between various % Cd removals observed in soils with a wide range of Cd concentration. This kind of comparison is therefore possible to be biased in some manner. However, these comparisons are reasonable and useful to attain general but critical information for future studies, especially considering that they were made with relatively large datasets (n > 80).
3.1 The Efficiency of Different Herbaceous Species
To date, most heavy metal hyperaccumulators identified are grasses. So, it is not surprising that many researchers have paid much attention to herbaceous species. In the past 10 years, more than 160 herbaceous species have been investigated for their abilities to extract Cd from soils, by means of pot experiments. Most of these studies have come from Asia. In a large-scale pot experiment, a Japanese research group assessed the Cd-accumulating capacities of 101 herbaceous species (Abe et al., Citation2008). More recently, in another pot experiment from Asia, the Cd-accumulating characteristics of 24 weed species were investigated to screen plants useful in Cd phytoextraction (Wei et al., Citation2009).
The calculated Cd removal percentages of herbaceous test species vary widely from 0.004% to 38.8% (Barrutia et al., Citation2009; Koopmans et al., Citation2008). The maximum value so far observed is in a high Cd-accumulating ecotype (Ganges) of the well-known Cd hyperaccumulator Thlaspi caerulescens (Koopmans et al., Citation2008). However, it should be noted that some newly identified Cd-accumulating species (but not ranked as Cd hyperaccumulators) have also shown excellent capacities for extracting Cd from soils. For example, Glycine max is able to remove as much as 19.7% of the total amount of soil Cd (Murakami et al., Citation2007). Listed in are the herbaceous species that have been reported to be capable of extracting >5% of the total Cd in soils. Here, >5% is selected as a threshold value because it means that the plant can remove 50% of the total Cd in soil within 15 years (), assuming the Cd removal percentage would not change over time (Meers et al., Citation2004; Zhao et al., Citation2003). If the Cd removal potentials of these plant species () can be realized in practice, they can all be considered as promising candidates for large-scale Cd phytoextraction. It has been proposed that a realistic goal for phytoextraction is to reduce concentrations of heavy metals in contaminated soils to acceptable levels within a reasonable time span (<20 years; Huang et al., Citation1997). A 50% reduction in total soil Cd indicates that the greater proportion of bioavailable Cd in soil will be removed as the most bioavailable fraction of Cd always accounts for <50% of the total soil Cd (Basta and Gradwohl, Citation2000; Ma and Rao, Citation1997; Prokop et al., Citation2003).
TABLE 1 List of pot-grown herbaceous species that have been found to be able to remove >5% (on yearly basis) of total soil Cd in a pot
FIGURE 1 Calculated % Cd remained in soil after phytoextraction for years by various plant species that have different Cd removal percentages (RP; on yearly basis).
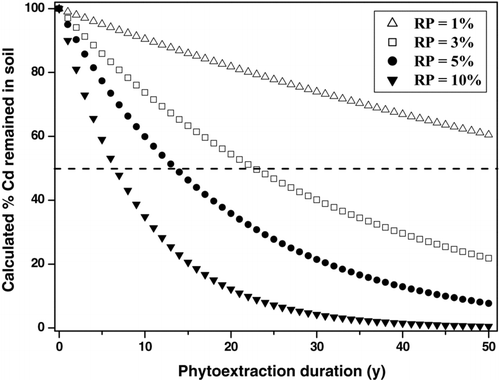
In general, the major factors affecting the efficiency of Cd removal by pot-grown plants are type of plant species, Cd level in soil, the source of soil Cd, and soil pH (Fischerová et al., Citation2006; Wang et al., Citation2006; Yanai et al., Citation2006; Koopmans et al., Citation2008). Based on the data available from literature we have attempted to quantify the effects of these factors. Comparisons between plant groups for these factors were performed using nonparametric Mann-Whitney U tests. It was found that the average % Cd removal by hyperaccumulators was about 3 times higher than that of nonhyperaccumulators (; Mann-Whitney, n 1 = 29, n 2 = 90, p < .001). This result appears to justify the great eagerness of many researchers to screen Cd hyperaccumulators. When soils with <10 mg kg−1 Cd were defined as slightly to moderately contaminated soils (Zhao et al., Citation2003), the average % Cd removal of the plant species grown in these soils was nearly 4 times of that in heavily contaminated soils (>10 mg kg−1; ; Mann-Whitney, n 1 = 61, n 2 = 58, p < .001). According to these results, soil Cd level seems to be almost as important as type of plant species in determining the % Cd removal by pot-grown plant species. Cadmium is always more bioavailable in spiked, artificial soils than in anthropogenically contaminated soils (Prokop et al., Citation2003; Tang et al., Citation2006). However, we have found that the average % Cd removal by plant species grown in spiked artificial soils was 1.7 times lower than that in anthropogenically contaminated soils (; Mann-Whitney, n 1 = 67, n 2 = 52, p < .01). This unexpected result may be largely explained by the result of a further Mann-Whitney U test on the dataset which showed that the average concentration of total Cd in spiked artificial soils used in the pot experiments was 15 times higher (n 1 = 67, n 2 = 52, p < .001) than that in the anthropogenically contaminated soils used (data not shown). Overall, the plant species performed better in acidic soils (pH < 7) than in alkaline soils (pH > 7), resulting in a 1.6 times higher % Cd removal (; Mann-Whitney, n 1 = 81, n 2 = 38, p < .01). We propose that this result is likely to be attributed to the relatively high Cd bioavailability in the acidic soils (Meers et al., Citation2005a; Yanai et al., Citation2006).
3.2 The Efficiency of Different Woody Species
Woody species are typical of high-biomass plants and their potential use in Cd phytoextraction has recently received increasing attention (Dickinson and Pulford, Citation2005; Pulford and Watson, Citation2003; Saraswat and Rai, Citation2011). They are therefore worthy of a specific discussion here. Given the long life cycle of most woody species, they are generally considered to be unable to evolve rapidly enough to adapt to heavy metal–contaminated sites. For this reason, it has been presumed that few trees have developed a capacity to tolerate and accumulate Cd (Pulford and Watson, Citation2003). In the past decade, only about 20 woody species have been pot-grown to test their capacities to extract Cd from soils. Unlike those focusing on herbaceous species, most of these studies came from Europe. The most extensively investigated species are some fast-growing trees belonging to the genera Salix and Populus (Meers et al., Citation2007; Mertens et al., Citation2006; Pulford and Watson, Citation2003; Wu et al., Citation2010). In addition, it should be noted that an Australian research group has explored the Cd accumulating capacities of 6 Salix spp., and 2 Populus spp. for selection of Cd accumulator species (Dos Santos Utmazian and Wenzel, Citation2007).
Based on the data presented in literature, the % Cd removals by eight species were able to be calculated accurately (). The maximum value (11.2%) was recorded in Salix × smithiana (Vysloužilová et al., Citation2003), being nearly 2.5 times lower than that of herbaceous species (). Furthermore, only three species were found to be capable of extracting >5% of the total soil Cd in pots (). This result may partly be explained by two possible reasons. First, as mentioned previously, the number of woody species tested is still small. Secondly, the growth of woody species tends to be more heavily inhibited by the limited soil volume in pots, compared with that of herbaceous species (Arnold and McDonald, Citation1999). It is therefore proposed that the phytoextraction potential of woody species deserves further investigation, especially when considering that there has been some evidence showing that some woody species from uncontaminated sites also can accumulate Cd to relatively high levels (Dos Santos Utmazian and Wenzel, Citation2007; Pulford and Watson, Citation2003).
TABLE 2 List of pot-grown woody species whose % Cd removals (on yearly basis) could be calculated accurately on the basis of data presented in literature
3.3 The Effect of Soil Amendments
It must be noted that the % Cd removals presented previously in the two subsections were obtained without any soil manipulation or amendment. The idea of using soil amendments to increase the concentration of bioavailable heavy metals in soils and subsequently facilitate the metal uptake by phytoextractors was originally inspired by studies on plant nutrition (Marschner, Citation1995). This approach has frequently been proven efficient in Pb phytoextraction (Huang et al., Citation1997; Komárek et al., Citation2007; Shen et al., Citation2002). However, its efficacy in Cd phytoextraction is always less certain which is largely due to the fact that Cd in soils is usually readily bioavailable (Evangelou et al., Citation2007; Santos et al., Citation2006; Sun et al., Citation2001; Tandy et al., Citation2006).
In the past decade, more than 10 different chelating agents have been used to enhance Cd removal by pot-grown plant species (). These chelators can broadly be classified into two major groups: synthetic chelants and natural compounds (Leštan et al., Citation2008; Nowack et al., Citation2006). The former can be further divided into nonbiodegradable (e.g., EDTA and DTPA) and biodegradable (e.g., EDDS and NTA) subgroups, whereas members of the latter group (e.g., citric acid and oxalic acid) all tend to show a high biodegradability (Leštan et al., Citation2008; Meers et al., Citation2005a; Nowack et al., Citation2006). Due to their lower environmental risks compared with nonbiodegradable ones, biodegradable chelators have recently received increasing attention (Leštan et al., Citation2008; Nowack et al., Citation2006; Sun et al., Citation2009).
TABLE 3 Examples of pot experiments using chelate to improve % Cd removal of plant species
The extent of Cd solubilization by chelators follows the order of the stability constants of chelating complexes generated from the chelators and the Cd2+ cation (Leštan et al., Citation2008; Schmidt, Citation2003). It has typically been found that the stability of complexation of Cd with various chelating agents is in decreasing order: EDTA > HEDTA > DTPA > EDDS > NTA > citric acid > oxalic acid > acetic acid (Borowiec et al., Citation2009; Leštan et al., Citation2008; Schmidt, Citation2003). Theoretically, the efficiency of these chelants in enhancing Cd phytoextraction should therefore follow this order. As expected by this theoretical prediction, many studies have found that EDTA is the most efficient chelant (Leštan et al., Citation2008; Luo et al., Citation2005; Peñalosa et al., Citation2007). However, there is some inconsistent evidence showing that EDDS or citric acid are more efficient than EDTA (Meers et al., Citation2005a; Sun et al., Citation2009). Luo et al. (Citation2006; Citation2007) found that the application of hot (90°C) EDTA (3 mmol kg−1) or EDDS (5 mmol kg−1) is able to improve the % Cd removal by Phaseolus vulgaris by a factor of 40.5 or 17.4, respectively (). These authors argued that these enhancements were probably attributed to the root damage resulting from the hot solutions, which allowed Cd to enter the root xylem easily. For the other chelators, however, they generally produced less than a 1.5× increment in % Cd removal (). In a worst-case scenario, the application of 1.8 mmol HEDTA per kg soil caused a 0.59× decrease in % Cd removal by Helianthus annuus, which was largely due to its high toxicity to the plant (; Chen and Cutright, Citation2001).
When the applicability of chelant-enhanced Cd phytoextraction is taken into account, the % Cd removal by chelant-treated plants is more important than the increase/decrease factor [(% Cd removal by chelant-treated plants/that of the control) – 1] per se. However, this concern has always been little addressed in literature. Here, our statistical analysis based upon the available data from literature indicates that in most cases the % Cd removals by chelant-treated plants were <5% (). The only one exception is the value (17.5%) recorded in a Cd hyperaccumulator Sedum alfredii that was treated with 8 mmol kg−1 citric acid (Sun et al., Citation2009). Taken together, the present results seem to suggest that the efficiency of the so far tested chelators is still insufficiently high to consider their large-scale applications in the field.
3.4 The Effect of Microorganisms
Another important approach to improve the % Cd removal by plant species considers plant-microbe interactions. The mutual relationships between plants and the associated microorganisms have long been recognized and are widely applied to promote plant growth for the production of food, fiber, biofuels, and key metabolites (Wu et al., Citation2009). The potential use of plant-microbe symbioses in Cd phytoextraction has received little attention until recently although its possible effects on the phytostabilization process have been well documented (Dell’Amico et al., Citation2005; Grandlic et al., Citation2008, Citation2009; Leyval et al., Citation1997).
Rhizosphere microorganisms can promote Cd phytoextraction through improving plant growth or by enhancing Cd accumulation by plants (Weyens et al., Citation2009). Diverse mechanisms are involved in microorganism-assisted plant growth: (a) production of particular compounds such as siderophores, indole-3-acetic acid, 1-aminocyclopropane-1-carboxylic acid, and giberellic acid, which can directly affect plant metabolism (Idris et al., Citation2004; Khan et al., Citation2009; Rajkumar and Freitas, Citation2008); (b) improvement of plant nutrient acquisition by fixing N from atmosphere or liberating phosphorus from organic compounds (Çakmakçi et al., Citation2006; Khan et al., Citation2009); and (c) protection of plants from certain diseases by secreting diffusible or volatile antibiotics and toxins (Berg, Citation2009; Khan et al., Citation2002). On the other hand, rhizosphere microorganisms may play an important role in enhancing Cd accumulation by plants through two major processes: increasing the transport of soluble Cd into roots and facilitating the solubilization of nonbioavailable forms of Cd in soils by reducing soil pH, and synthesizing chelators (e.g., organic acids) and siderophores (Abou-Shanab et al., Citation2003; Gadd, Citation2004; Whiting et al., Citation2001).
During the past few years, approximately 20 species of microorganisms have been used to improve the % Cd removal by pot-grown plant species. Based on the available data from literature, we have attempted to assess the efficiencies of these microorganisms by calculating the increase/decrease factor according to the following equation: increase/decrease factor = [(% Cd removal by microorganism-treated plants/that of the control) – 1]. Listed in are those microorganisms whose efficiencies could be calculated accurately. For a given species, the maximum value of its efficiency is presented when multiple values were obtained.
TABLE 4 List of microorganisms capable of improving % Cd removal of pot-grown plant species
The most widely investigated species are fungi, especially arbuscular mycorrhizal fungi (AMF; ). This may be partly attributed to the fact that mycorrhizal fungi have been recognized as the only ones providing a direct linkage between soil and plant roots (Leyval et al., Citation1997). Furthermore, it has been found that among the tested microorganisms an AMF species (Glomus mosseae) showed the highest efficiency (i.e., increase factor) in enhancing the % Cd removal by the pot-grown plant species (). Usman and Mohamed (Citation2009) fully investigated the effect of microbial inoculation on the removal of heavy metal by high-biomass crops and demonstrated that an AMF species G. mosseae was able not only to promote the growth of Zea mays but also to increase Cd accumulation in this species, which led to a 2.4-fold higher % Cd removal in the fungus-inculcated individuals than in the control ones (). On the other hand, there is considerable evidence that bacteria were able to improve the % Cd removal by plants (). For example, Sheng and Xia (Citation2006) utilized the bacteria isolated from heavy metal–contaminated soils to enhance the Cd removal of Brassica napus and found that a bacterial strain Bacillus sp. RJ16 was capable of improving the % Cd removal of B. napus by a factor of 1.4. The authors also showed that this positive effect could be attributed to the bacteria-induced decrease in rhizosphere soil pH and production of indole-3-acetic acid. However, it must be noted that the % Cd removals by the microorganism-treated plants were too low (∼1%; ) for efficient phytoextraction, which may be also applied to explain why few field trials have been conducted to explore the Cd removal efficiency of these plants.
4. FIELD APPLICATION OF CD PHYTOEXTRACTION
As research on Cd phytoextraction has gradually progressed from a laboratory stage toward field scale in recent years, a number of field trials have been carried out to obtain a more realistic estimate of the Cd phytoextraction potentials of candidate plants. On this basis, we have attempted to build up a good understanding of the practical applicability of Cd phytoextraction with a focus on the field-derived Cd removal efficiencies of various plants. Unless otherwise defined, the % Cd removals (on yearly basis) presented in this section were calculated according to the method proposed by Zhao et al. (Citation2003).
4.1 The Most Important Factors Influencing Cd Removal Efficiency
As discussed in the previous section (3.1), many results from laboratory studies have indicated that types of plant species, Cd concentration in soil, soil pH, and experimental duration are among the most important factors that may greatly influence the Cd removal efficiency of plants. However, to date, few field trials have been conducted to determine the effects of these important factors (Hammer et al., Citation2003; Li et al., Citation2009a; McGrath et al., Citation2006; Mertens et al., Citation2006) and no study has taken into account all of these factors. In fact, for field trials, many contextual factors are site-specific and it is difficult to effectively manipulate factors such as soil Cd concentration and soil pH. Alternatively, using the data from literature, we made some statistical calculations to give an overview of the effects of these important factors on the Cd removal efficiencies of field-grown plants.
There has been debate about whether low-biomass hyperaccumulators are more effective than high-biomass nonhyperaccumulators in heavy metal phytoextraction (Chaney et al., Citation2007; Ebbs and Kochian, Citation1998; McGrath and Zhao, Citation2003). Here, our statistical analysis showed that the average % Cd removal of hyperaccumulators was approximately 1.9× as high as that of nonhyperaccumulators (; Mann-Whitney, n 1 = 9, n 2 = 78, p < .05). On the one hand this result seems to lend some support to the notion that hyperaccumulators tend to play a more important role in Cd phytoextraction than nonhyperaccumulators (Chaney et al., Citation2007; Hammer et al., Citation2003; McGrath and Zhao, Citation2003). On the other hand, it only reflects a general trend and does not exclude the possibility that in some cases nonhyperaccumulators can perform as well as or even better than hyperaccumulators (see and the relevant text to come).
TABLE 5 List of field-grown plant species proven to be able to remove >5% (on yearly basis) of total Cd in the top 20 cm soil layer
FIGURE 3 Response of % Cd removal (M ± SE) of field-grown plant species to effects of type of plant species (A), total Cd concentration in soil (B), soil pH (C), and experimental duration (D). ns = not significant. *p < .05. ***p < .001 (Mann-Whitney U tests).
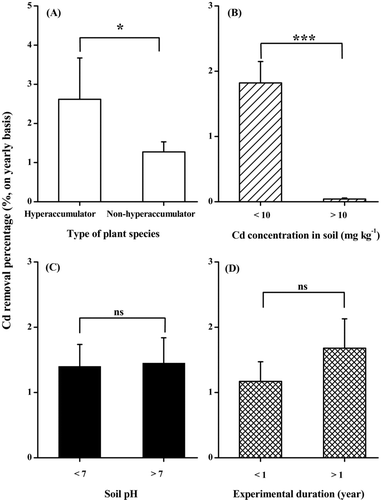
It has been proposed that phytoextraction is a promising technology to decontaminate soils with low to moderate metal concentrations rather than those heavily contaminated (McGrath and Zhao, Citation2003). In agreement with this statement, we found that the average % Cd removal of plants grown on slightly to moderately Cd-contaminated soils (<10 mg kg−1) was 1.82%, being 42-fold higher than that of heavily contaminated soils (; Mann-Whitney, n 1 = 67, n 2 = 20, p < .001).
In contrast to the result of statistical analysis based on data from laboratory experiments (), there was no significant difference in average % Cd removal between plants grown in acidic field soils and in base-rich, high pH field soils (; Mann-Whitney, n 1 = 63, n 2 = 24, p > .05). This inconsistent result suggests that soil pH may play a less important role in determining Cd removal efficiencies of plants at the field scale than at the laboratory scale, which is possibly attributed to the fact that in the field there are many other critical environmental factors affecting the soil Cd bioavailability (Ernst, Citation1996; Vangronsveld et al., Citation2009).
In addition, it was unexpectedly found that the experimental duration (<1 year vs. >1 year) did not seem to significantly impact the Cd removal efficiencies of field-grown plants (; Mann-Whitney, n 1 = 45, n 2 = 42, p > .05). There are two possible reasons for this unexpected result. First, most field trials available were of much shorter duration than three years. In such a relatively short time span, the decline of the bioavailable Cd pool in soils may be not great enough to cause a significant decrease in Cd removal efficiency of the plants (Koopmans et al., Citation2008; van Nevel et al., Citation2007; Wieshammer et al., Citation2007). Second, some plant species tend to show a higher % Cd removal in the second/third growing season than in the first, which may be attributed to their improved subsequent growth after they have acclimated to local environmental conditions (Li et al., Citation2009a; Mertens et al., Citation2006).
4.2 The Most Promising Plant Species for Cd Phytoextraction
In the past decade, more than 60 plant species have been tested under field conditions to explore their potential for Cd phytoextraction. Among them, T. caerulescens, Brassica juncea, H. annuus, Z. mays, B. napus, and Salix viminalis are the most widely investigated species. Most of this kind of study has been conducted with a focus on comparing the % Cd removals of different cultivars/clones of the same species or different species within the same genus to screen superior plant varieties to extract Cd from soils (Lombi et al., Citation2000; Mertens et al., Citation2006; Nehnevajova et al., Citation2005).
There are certainly fruitful results from the field trials. However, perhaps the most important outcome is that some promising plant species for commercial Cd phytoextraction have been identified. The plant varieties that have been demonstrated to be able to extract >5% of total Cd in top 20-cm soil layer (on an annual basis) are summarized in . There is strong evidence so far that the first known Cd hyperaccumulator T. caerulescens is one of the most promising candidates (Hammer and Keller, Citation2003; McGrath et al., Citation2006). An excellent case study was conducted by McGrath et al. (Citation2006), who found that a superior Cd-accumulating race of T. caerulescens Ganges was able to remove 21.7% of the total soil Cd when it was grown for 14 months (i.e., the annual % Cd removal of Ganges plants could be as high as 10.9%; ). Assuming a linear removal (Meers et al., Citation2004; Zhao et al., Citation2003), only 6 years would then be needed for a crop of Ganges plants to reduce the total Cd in the soil to 50%. Such a short time-span is desirable for any commercial phytoextraction (Huang et al., Citation1997). In addition, the Cd-rich T. caerulescens produced from phytoextraction can be disposed by pyrolysis. It may allow the recovery of Cd and recycling of Cd-free bottom ashes as fertilizers (Keller et al., Citation2005). However, this species is susceptible to pests, weed competition, drought, and hot weather, which make it difficult to maintain T. caerulescens in the field (Assunção et al., Citation2003a; Kayser et al., Citation2000; Vangronsveld et al., Citation2009). This is also a major practical problem facing most other potential phytoextractors. In the short term, there are two possible solutions to this problem. One is to get an extended knowledge of effective agronomic practices for phytoextraction (Vangronsveld et al., Citation2009). The other is to improve relevant agronomic characteristics of potential phytoextractors by screening outstanding phenotypes/populations/individuals (Schwartz et al., Citation2006). In the long term, improvements in potential phytoextractors’ abilities to deal with various environmental stressors may be achieved through genetic engineering processes (Cherian and Oliveira, Citation2005; Doty, Citation2008). Recently, a Japanese research group demonstrated that several Indica-type rice (Oryza sativa) cultivars were quite efficient in phytoextraction of Cd-contaminated soils (Ibaraki et al., Citation2009; Murakami et al., Citation2009). Among these cultivars, Chokoukoku is the most extreme example, as its annual % Cd removal also reached 10.9%. However, as noted by the authors, the use of rice for Cd phytoextraction highlights the environmental and health risks associated with this technology (Murakami et al., Citation2009). The high Cd concentrations in phytoextractors (especially crop plants such as rice) may pose risks not only for soil microbes and animals in the surrounding areas, but also for residents (van Nevel et al., Citation2007). There are some recommended measures that can be taken to lower the risks, for examples, harvesting phytoextractors before leaf-fall or fruiting period, collecting as much plant litter as possible at intervals, preventing animals from reaching phytoextractors (Dickinson and Pulford, Citation2005; Li et al., Citation2009a; Mertens et al., Citation2006; van Nevel et al., Citation2007). In China, there are presently two plant species (i.e., Averrhoa carambola and Sedum plumbizincicola; ) that have been proven to be able to remove >5% (on yearly basis) of total Cd in the top 20 cm soil layer when grown in the field (Li et al., Citation2009a; Li et al., Citation2010; Liu et al., Citation2009). At this point, it should also be kept in mind that the % Cd removals listed in were obtained under general field managements and without any additional soil manipulation. Therefore, there is a possibility that the Cd removal potentials of these plant varieties can be further improved in the future by optimizing agronomic practice and soil conditions (Wei et al., Citation2008; Zhao et al., Citation2003).
It is apparent, however, that such a species list in does not tell the whole story. Phytoextraction of Cd with some Salix spp. has been in practice for many years in Europe, although their % Cd removals are lower than 5% (Dickinson and Pulford, Citation2005; Hammer et al., Citation2003; Mertens et al., Citation2006). There are three major reasons for this unusual phenomenon. First, Europe has a long history of commercial exploitation of willows, which yields a well-established agronomic system for this and similar tree crops (Paulson et al., Citation2003). Secondly, willows are high-biomass and fast-growing, which can produce profitable biomass within a relatively short time span and thereby provide the farmer/grower with an additional income (Dickinson and Pulford, Citation2005; Paulson et al., Citation2003). Third, the stricter environmental laws in Europe limit food production on contaminated lands (European Union, Citation2000), so that more lands can be available for remediation although a long cleanup time would be required to reach the remediation target. Nevertheless, it should be noted that the encouraging results mentioned above were all observed in slightly to moderately Cd-contaminated soils (<10 mg kg−1) and the relevant field trials were conducted in Europe or Asia. These results, therefore, highlight (a) a unique opportunity for successful phytoextraction of slightly to moderately Cd-contaminated soils in Europe and Asia and (b) an urgent need for more research on Cd phytoextraction in other parts of the world, especially where soil Cd contamination is also among the major environmental problems.
4.3 The Dilemma for Chelant-Enhanced Cd Phytoextraction
Continued efforts have been made to determine the efficacy of chelant-enhanced Cd phytoextraction under field conditions, although a number of pot experiments have shown that this approach is not ready for full implementation. Likewise in pot experiments, the most widely investigated chelant in field experiments is EDTA. However, few data on the efficiencies of biodegradable chelants (especially EDDS) in the field are available, which may be at least partly due to the fact that most of this kind of chelant is still too expensive to be used at field scale now.
Listed in are those field experiments in which the % Cd removal of plant species and the efficiency of the chelant (increase/decrease factor) can be calculated using the data presented in literature. Unfortunately, it was found that the chelants tested were much less efficient, compared with the results from controlled-environment experiments (). That is particularly the case for EDTA. Specifically, the increase/decrease factors of this chelant ranged from 0.48 to −0.62 (Neugschwandtner et al., Citation2008; Zhuang et al., Citation2007), depending on the dose used and the identity of plants considered (). This means that EDTA can barely double the % Cd removals of those plants tested, even in a best-case scenario (Zhuang et al., Citation2007). By contrast, a relatively high increase factor has been observed for sulfur. Kayser et al. (Citation2000) found that the addition of sulfur at a high dose was able to increase the % Cd removal of a high-biomass crop of H. annuus by a factor of 3 ().
TABLE 6 Examples of field experiments using chelant to improve % Cd removal of plant species
A central dilemma facing chelant-enhanced Cd phytoextraction is how to improve efficiency. There are some examples in literature claiming that chelant-enhanced phytoextraction is a promising approach to clean up Cd-contaminated soils. However, we believe that this kind of statement is somewhat optimistic as presently available data showed that the % Cd removals of chelant-treated plants grown under field conditions are generally <1% (). This follows that it would still take >50 years for these plants to remove 50% of the total Cd from the soils (), assuming that the % Cd removal will not change over time (Meers et al., Citation2004; Zhao et al., Citation2003).
Another important problem for chelant-enhanced Cd phytoextraction is how to reduce the potential ecotoxicological risks associated (Nowack et al., Citation2006). As to nonbiodegradable chelants, they are per se of concern because they may endanger soil biota and leach down to groundwater and aquifers. In the case of EDTA, it has a negligible toxicity profile only when its concentration in an aqueous environment is <2.2 mg L−1 (about 10 μM; Grundler et al., Citation2005). However, when applied to the field, the dose of EDTA is generally much higher than 1 mM (see for some examples). Considering that at best only about 10% of the EDTA added can be taken up by plants (Nowack et al., Citation2006), an overwhelming majority of the EDTA applied remains in soils, presenting potentially high ecotoxicological risks. For all chelants, leaching of mobilized soil Cd down to groundwater seems to be unavoidable (Nowack et al., Citation2006). There is evidence that, even in the best-case scenario, about 10% of the solubilized soil metal will be absorbed into plants (Lombi et al., Citation2001; Thayalakumaran et al., Citation2003). The remainder in soil readily leaches into receiving water (Schmidt, Citation2003). In summary, successful chelant-enhanced Cd phytoextraction is still a blue-sky vision.
4.4 A Comparison Between the Field and Laboratory Experiments
It has long been recognized that the % Cd removals of plant species observed in pot experiments are always overestimates compared to those from field trials. However, little is known about the extent to which this kind of overestimation and how it will vary depending on plant species. We attempted to provide some general quantitative assessments for such kind of overestimation with the data from literature.
It is somewhat surprising that the most overestimated species was a Cd hyperaccumulator Arabidopsis halleri. In detail, when grown in pots the average % Cd removal of this species was 5.13%, which was 513× of that from the field trial (). This may be largely attributed to the extremely low biomass production of this species in the field (McGrath et al., Citation2006). Another important reason for the large discrepancy probably relates to the heterogeneous nature of soil Cd contamination in the field (Dickinson and Pulford, Citation2005; Robinson et al., Citation2006). Both spatial and temporal variability in the distribution of contaminants would make it more difficult for some roots come into close contact with the contaminants, leading to a reduced uptake of the contaminants (Robinson et al., Citation2006). In the only exceptional case, the average % Cd removal of pot-grown Phaseolus vulgaris was only about 50% of the value observed in the field trial (). For most other species, however, it was found that pot experiments always overestimated the average % Cd removals by approximately 10× compared with the values recorded in the field (). Thlaspi caerulescens and Z. mays are the strong examples for this pattern. A considerable number of pot and field experiments have been conducted with these two species (for references see ) and so provide relatively large datasets for our statistical analysis. It should also be noted that most of our results mentioned previously are preliminary but can be useful indicators for future studies on Cd phytoextraction. They at least allow an estimation of the Cd phytoextraction potential of a candidate plant species under field conditions based on the data from pot experiments, thereby determining whether a species is worthy of field trialing. This is important, especially considering that field experiments are relatively expensive and time consuming compared to pot experiments.
TABLE 7 Comparison of the % Cd removal of pot-grown plant species (RPCd-p) and that of field-grown plant species (RPCd–f)
5. CHALLENGES AND EMERGING INTERESTS
The information presented previously suggests that there has been significant progress in the field of Cd phytoextraction over the past decade. However, there are challenges ahead and more fundamental studies are still needed to pave the way for commercial Cd phytoextraction. From a practical perspective, the most urgent challenges to be overcome include (a) how to further improve the efficiency of Cd phytoextraction, (b) how to cut the overall costs of Cd phytoextraction, and (c) how to get greater stakeholders’ acceptance of Cd phytoextraction. There are emerging interests in addressing these challenges, which can be highlighted as following.
5.1 How to Further Improve the Efficiency of Cd Phytoextraction?
Phytoextraction of Cd-contaminated soils has increasingly become a multidisciplinary topic. It would therefore benefit from the efforts of not only environmental scientists, but also biologists and agronomists. Discussed subsequently are major approaches that may be applied to further improve the efficiency of Cd phytoextraction.
Natural or Transgenic High-Biomass Cd Hyperaccumulators
Although several natural hyperaccumulators of Cd have so far been reported (Baker et al., Citation1994; Bert et al., Citation2002; Liu et al., Citation2004; Vogel-Mikuš et al., Citation2005; Wei et al., Citation2005; Xu et al., Citation2009; Yang et al., Citation2004), there is no doubt that the lack of high-biomass Cd hyperaccumulators remains a major bottleneck in the development of commercial Cd phytoextraction (Chaney et al., Citation2007). One classical way to gain such a desirable species is to screen naturally occurring high-biomass Cd hyperaccumulating plants in large-scale field surveys followed by experiments under controlled-environment conditions (Baker and Whiting, Citation2002). The majority of the known hyperaccumulators of metals or metalloids are low-biomass plants. However, the phenomenon does not exclude the possibility that a combination of Cd hyperaccumulation and high-biomass can be found in the plant kingdom (McGrath and Zhao, Citation2003). It is worthy of emphasis that in future studies regarding identification of natural Cd hyperaccumulators more attention should be paid to high-biomass species. Another possible way to attain such a desirable species is to transfer the hyperaccumulation genes to high-biomass plants (Rugh et al., Citation1998). Genetic engineering had earlier been considered as the best long-term strategy for improving heavy metal phytoextraction (Raskin et al., Citation1997). Recent advances in understanding the molecular mechanisms underlying Cd tolerance and accumulation in plants have given researchers the ability to develop transgenic plants for Cd phytoextraction (Cherian and Oliveira, Citation2005; Doty, Citation2008; Farinati et al., Citation2010). There have been efforts to introduce the genes involved in sulfur metabolism, glutathione and phytochelatin synthesis, and metallothionein and adenosine triphosphate (ATP)–binding cassette transporter expression into high-biomass plants such as B. juncea, Nicotiana tabacum, and Populus spp. (Bennett et al., Citation2003; Janoušková et al., Citation2005; Kawashima et al., Citation2004; Song et al., Citation2003). When grown in pots or hydroponics, these transgenic plants often showed significantly higher Cd tolerance and shoot Cd than wild-type plants (Bennett et al., Citation2003; Janoušková et al., Citation2005; Kawashima et al., Citation2004). However, the available pot experiments suggest that the % Cd removals of these transgenic plants are always < 1.5% (Janoušková et al., Citation2005) and little information exists about their abilities to extract Cd from contaminated soils under field conditions. On the other hand, there is increasing evidence that genes (e.g., HMA2 and HMA4) encoding metal pumps in the P-type ATPase superfamily are responsible for Cd hyperaccumulation in plants (Hanikenne et al., Citation2008; Papoyan and Kochian, Citation2004; Verret et al., Citation2004; Wong and Cobbett, Citation2009; Wong et al., Citation2009). In the future, therefore, more effort should be encouraged to address the possibility for further enhancing Cd phytoextraction via overexpression of these kinds of genes in high-biomass plants.
Intercropping
In the practice of agriculture, intercropping offers farmers the opportunity to achieve a greater yield per unit land area by growing two or more crops in proximity, whereby crops can make use of resources that would otherwise not be utilized by a single crop (Karpenstein-Machan and Stuelpnagel, Citation2000). From the perspective of phytoextraction, however, a major mechanism for enhancing phytoextraction by intercropping is that an intercropped hyperaccumulator can mobilize selected metals for a coplanted high-biomass nonhyperaccumulator (Gove et al., Citation2002). On the other hand, intercropping also can promote the growth of both cocropped plant species compared to monoculture cropping (Jiang et al., Citation2010; Liu et al., Citation2005). In recent years, there has been increased interest in the role that intercropping may play in further improving Cd phytoextraction. Besides the typical Cd hyperaccumulator + high-biomass Cd accumulator intercropping systems such as A. halleri + Salix caprea (Wieshammer et al., Citation2007), S. afredii + Z. mays (Liu et al., Citation2005; Wu et al., Citation2007), T. caerulescens + Hordeum vulgare (Gove et al., Citation2002), and T. caerulescens + Salix dasyclados (Fuksová et al., Citation2009), some high-biomass Cd accumulator + high-biomass Cd accumulator intercropping systems including B. napus + Z. mays (Selvam and Wong, Citation2009) and Medicago sativa + Z. mays (Li et al., Citation2009b) have also been tested. The results obtained showed that the Cd hyperaccumulator + high-biomass Cd accumulator systems are always more efficient than high-biomass Cd accumulator + high-biomass Cd accumulator systems in extracting Cd from the soils. The most efficient intercropping system tested was T. caerulescens + Salix dasyclados, whose % Cd removal was 8.65%, being about 1.2× as high as that of a T. caerulescens monoculture (Fuksová et al., Citation2009). However, it should be noted that these studies, with one exception (Wu et al., Citation2007), were conducted in pots under controlled-environment conditions. For this reason, more field trials are needed to determine the real efficacies of various intercropping systems. Moreover, there is an important lesson from the previous studies that the Cd removed by hyperaccumulators often accounted for the majority (60–90%) of the total extracted by the intercropping systems (Fuksová et al., Citation2009; Wu et al., Citation2007). On this basis, it should be proposed that when considering the establishment of a successful intercropping system for enhancing Cd phytoextraction, one should attempt to enable a hyperaccumulator to achieve its maximum possible contribution in the system.
Fertilization and Irrigation
Proper management of fertilization and irrigation has long been recognized as one of the most efficient but easily achievable means to increase the yields of crops (Ercolia et al., Citation1999). There has been some evidence that fertilization with a single nutrient (e.g., nitrogen [N], phosphorus [P], potassium [K], and calcium [Ca]) or a combination of several nutrients can greatly improve the efficiency of Cd phytoextraction through promoting biomass yield or Cd content in plant shoot (Catherine et al., Citation2006; Sun et al., Citation2007). It should be noted, however, that this kind of positive effect seems to be fertilizer form-, species- and site-specific. In the case of N fertilizer, supplying NO3 − is more efficient than supplying NH+ 4 in promoting the Cd phytoextraction by T. caerulescens (Schwartz et al., Citation2003; Xie et al., Citation2009), whereas the opposite is true for H. annuus (Zaccheo et al., Citation2006). As to a given N fertilizer, its efficacy for a species is also dependent on the initial fertility of target soils (Catherine et al., Citation2006; Schwartz et al., Citation2003). On the other hand, the effect of fertilization is closely related to irrigation. In a most recent study, Arao et al. (Citation2009) demonstrated that different irrigation strategies significantly affected the Cd accumulation in O. sativa. However, little attention has been paid to the impact of irrigation on the efficiency of Cd phytoextraction. Therefore, it can be deduced that in most cases the present management of fertilization and irrigation is far from ideal for the various candidate species for Cd phytoextraction (Wei et al., Citation2008; Zhao et al., Citation2003). Elaborate and systematic studies especially under field conditions are needed to address the complex interactions between plant, fertilization, and irrigation practice, thereby identifying the optimal levels of fertilization and irrigation for each species concerned.
Optimization of Root-Zone Temperature
There has been documentary evidence that root-zone temperature seems to play a more critical role in determining plant growth than leaf temperature (Ziska, Citation1998). By maintaining an optimal root-zone temperature, it is possible to gain greater yield of crops (Díaz-Pérez, Citation2009). On the other hand, the promoted root growth has been expected to in turn facilitate heavy metal uptake by plants because it tends to increase the root surface for heavy metal absorption (Singh and Subramaniam, Citation1997). In the past decade, studies at laboratory and field-scale have shown that heavy metal accumulation in plants can indeed be increased significantly via manipulating root-zone temperature (Almås and Singh, Citation2001; Baghour et al., Citation2001; Fritioff et al., Citation2005). However, little attention has been given to the possible usability of these positive effects of optimizing root-zone temperature in promoting heavy metal phytoextraction. This aspect is worthy of further study in the future, particularly considering that there are many easily achievable ways (e.g., covering the root-zone soil surface with plastic sheet or rice straw) to alter root-zone temperature.
Optimization of Harvest Timing
The timing of harvest is a critical aspect of agricultural production, as it impacts not only the yield but also the quality of crops (McGauley and Way, Citation2002). Inspired by this kind of idea, researchers have recently attempted to promote the Cd phytoextraction potentials of some plants via optimizing the harvest timing for these species (Ji et al., Citation2011; Li et al., Citation2009a; Mertens et al., Citation2006; Wei et al., Citation2006). Based on the obtained results, several general recommendations that may be useful to further improve Cd phytoextraction in the future can be proposed as follows. First, for deciduous plants, harvest before leaf-fall occurring would be required to attain the peak % Cd removals, as the amount of Cd extracted by leaves may account for >50% of the total removed by the shoots (Mertens et al., Citation2006). Second, for annual plants, harvest at flowering stage is a recommended option because plants often reach their maximum biomass and accumulate relatively high amounts of Cd in their shoots at this time point (Malhi et al., Citation2007; Wei et al., Citation2006). More importantly, this approach would make another harvest possible (Wei et al., Citation2008). For example, Wei et al. (Citation2006) demonstrated that by harvesting the shoots of Solanum nigrum at its flowering stage twice a year it was possible to increase the Cd extraction efficiency of this plant by 75% compared with that of a single harvest at its maturity stage. Third, for perennial plants (especially trees), harvesting can be done at the end of the second growing season, as these plants tend to display a relatively higher annual % Cd removal in the first two growing seasons than in other years thereafter (Li et al., Citation2009a; Mertens et al., Citation2006).
A Realistic Choice: Development of Phytoextractor–Crop Rotation Systems
For continuous phytoextraction, targeted agricultural land has to be removed from food production for years or even decades (Dickinson et al., Citation2009). This is a critical reality that still requires considerable further attention, especially considering that the increase in food demand due to population growth is now a great challenge in many parts of the world (Food and Agriculture Organization of the United Nations, Citation2009). In such a context, development of phytoextraction–crop rotation systems should be a more realistic choice for most developing countries. However, the rotation interval will certainly depend upon several specific scenarios, such as the identity of plant species available and some major physical-chemical characteristics of the soil targeted. More fundamental studies are needed to help to establish efficient phytoextraction–crop rotation systems in the future.
5.2 How to Cut the Cost of Cd Phytoextraction?
Phytoextraction has been highlighted as a low-cost technology since it emerged in 1980s (Cunningham and Ow, Citation1996), but there is no doubt that considerable efforts are still needed to cut the cost of Cd phytoextraction. In general, the cost of Cd phytoextraction includes the expenses of plant material, fertilizer, water, labor, and disposal of harvested Cd-rich plant material. As shown subsequently, there are some potential means that may be useful to reduce these costs.
Development of Micropropagation Systems for Phytoextractors
A vital prerequisite for phytoextraction practice is to obtain enough seedlings of phytoextractors. However, there is evidence that some potential phytoextractors are often difficult to propagate. This is especially the case for some Cd hyeraccumulators, which have narrow geographic distribution and produce limited seeds having a very low germination capacity (dal Corso et al., Citation2005; Xu et al., Citation2008). Given that micropropagation is an economical and effective method to produce a large number of plant seedlings (Smith, Citation2000), development of micropropagation systems for candidate phytoextractors should be a good option to reduce the expense of preparing plant material. This idea has recently received increasing attention. For example, the micropropagation systems for A. halleri, T. caerulescens, and S. alfredii have been successfully established and it has also been demonstrated that the micropropagated seedlings retain their ability to hyperaccumulate Cd (dal Corso et al., Citation2005; Xu et al., Citation2008; Zhao et al., Citation2009).
Enhancing Fertilizer and Water use Efficiency of Phytoextractors
This approach has so far been seriously ignored. However, it may be possible to significantly reduce the relevant cost elements, particularly in arid and semiarid areas. Although many promising strategies for improving fertilizer and water use efficiency have been proposed, the two following appear to deserve more attention: (a) fertigation, a technology combining fertilization and irrigation to maximize the synergy between the two agricultural inputs (Ramah et al., Citation2009), and (b) improving root-zone microenvironment with mulch, which can reduce fertilizer leaching and water evaporation, and is also helpful to control some weeds and pests (Díaz-Pérez, Citation2009).
Establishment of Mechanical Planting and Harvesting Systems for Phytoextractors
Planting and harvesting phytoextractors by hand are especially labor intensive. Nowadays, however, except for some Salix species (Paulson et al., Citation2003), potential candidate plants for Cd phytoextraction do not have commercial mechanical planting or harvesting systems. This issue should be addressed in future studies to reduce the labor costs. More studies are also needed to test whether the candidate phytoextractors can regrow from the cut stumps and then if possible determine how many successive harvests a single planting may permit.
Making the Best use of Harvested Cd-Rich Plant Materials
It has been proposed that the harvested Cd-rich plant materials associated with Cd phytoextraction may be disposed by incineration or pyrolysis, both of which allow the recycling of bottom ashes as fertilizers (Keller et al., Citation2005). There is still a possibility that they can be used for production of bioenergy, paper, chip board, extruded trim, and so on (Licht and Isebrands, Citation2005). Future researchers should therefore address these potential usages to gain some income that may offset a fraction of the expense for disposal of the Cd-rich plant materials.
5.3 How to Get Greater Stakeholders’ Acceptance of Cd Phytoextraction?
It is less acknowledged that the low stakeholders’ acceptance is another important factor limiting the development of Cd phytoextraction (Robinson et al., Citation2006; Vangronsveld et al., Citation2009). The apparent cause of stakeholders’ unwillingness to accept Cd phytoextraction as a reliable option is the expected long remediation period due to the relatively low annual % Cd removal of available plant candidates (Dickinson et al., Citation2009; van Nevel et al., Citation2007), but the real cause is an uncertain economic viability of this technology (Robinson et al., Citation2003; Robinson et al., Citation2006; Vangronsveld et al., Citation2009). Up to now, it is still difficult to make accurate cost estimates for Cd phytoextraction schemes due to the lack of successful implementation of Cd phytoextraction (Chaney et al., Citation2007; Lasat, Citation2000), although Salt et al. (Citation1995) claimed that an estimated cost of phytoextraction (US$100,000 per hectare) accounted for only about one tenth of those of competing technologies such as soil removal and capping. According to Robinson et al. (Citation2006), in its pure form, Cd phytoextraction is presently a nonprofitable technology. However, there is evidence showing that Cd phytoextraction in combination with a profit-making operation could be an economically attractive option (Robinson et al., Citation2003; Thewys et al., Citation2010a, Citationb). Such an argument does not necessarily prevail with stakeholders. To get greater stakeholders’ acceptance, it is important to make stakeholders (i.e., government, land's owner, legislator, the public, funding agency, and land remediation company) realize that (a) the productivity or exploitability of land remediated by phytoextraction will be greater than that using nonbiological technologies, (b) in the long-term, phytoextraction may present greater social or environmental benefits than economic ones, and (c) similar to other technologies, Cd phytoextraction will take time to mature.
5.4 Phytoextraction Versus Phytostabilization
In conclusion, the progress achieved in the past decade has made Cd phytoextraction more efficient than ever before, although this technology is not yet ready for widespread application. It is time to consider to usher this technology into practical use to remediate slightly to moderately Cd-contaminated soils, especially those contaminated only by Cd, considering that most of the encouraging results from field trials () have been obtained in soils contaminated only by Cd at slight to moderate level (in most cases, soils with < 5 mg Cd kg−1). In reality, there are a large number of cases where other contaminants (inorganic or organic) may be present at high concentrations in Cd-contaminated soils (Dickinson et al., Citation2009). The presence of cocontaminants tends to lower annual % Cd removal by phytoextractors through prohibiting plant growth or reducing Cd uptake, thereby extending the period of Cd phytoextraction (Ernst, Citation2005). Furthermore, supplementary specific remediation schemes are probably needed to remove the cocontaminants, as there are few plant species that can (hyper)accumulate more than one contaminant (McGrath and Zhao, Citation2003). For such cases and those soils heavily contaminated by Cd, phytostabilization, in situ immobilization of contaminants using plants, is an applicable alternative to phytoextraction (Adriano et al., Citation2004; Ernst, Citation2005; Vangronsveld et al., Citation2009).
ACKNOWLEDGMENTS
The authors thank all of the researchers and scientists whose studies involved in Cd phytoextraction made this review possible. This work was supported by the National Natural Science Foundation of China (No. 30970548 and 31100372).
REFERENCES
- Abe , T. , Fukami , M. and Ogasawara , M. 2008 . Cadmium accumulation in the shoots and roots of 93 weed species . Soil Sci. Plant Nutr. , 54 : 566 – 573 .
- Abou-Shanab , R. A. , Angle , J. S. , Delorme , T. A. , Chaney , R. L. , van Berkum , P. , Moawad , H. , Ghanem , K. and Ghozlan , H. A. 2003 . Rhizobacterial effects on nickel extraction from soil and uptake by Alyssum murale . New Phytol. , 158 : 219 – 224 .
- Adriano , D. C. , Wenzel , W. W. , Vangronsveld , J. and Bolan , N. S. 2004 . Role of assisted natural remediation in environmental cleanup . Geoderma , 122 : 121 – 142 .
- Almås , Å.R. and Singh , B. R. 2001 . Plant uptake of cadmium-109 and zinc-65 at different temperature and organic matter levels . J. Environ. Qual. , 30 : 869 – 877 .
- Arao , T. , Kawasaki , A. , Baba , K. , Mori , S. and Matsumoto , S. 2009 . Effects of water management on cadmium and arsenic accumulation and dimethylarsinic acid concentrations in Japanese rice . Environ. Sci. Technol. , 43 : 9361 – 9367 .
- Arnold , M. A. and McDonald , G. V. 1999 . Accelerator containers alter plant growth and the root zone environment . J. Environ. Hort. , 17 : 168 – 173 .
- Assunção , A. G.L. , Schat , H. and Aarts , M. 2003a . Thlaspi caerulescens, an attractive model species to study heavy metal hyperaccumulation in plants . New Phytol. , 159 : 351 – 360 .
- Assunção , A. G.L. , ten Bookum , W. M. , Nelissen , H. J.M. , Vooijs , R. , Schat , H. and Ernst , W. H.O. 2003b . Differential metal-specific tolerance and accumulation patterns among Thlaspi caerulescens populations originating from different soil types . New Phytol. , 159 : 411 – 419 .
- Baghour , M. , Moreno , D. A. , Víllora , G. , Hernández , J. , Castilla , N. and Romero , L. 2001 . Phytoextraction of Cd and Pb and physiological effects in potato plants (Solanum tuberosum var. Spunta): Importance of root temperature . J. Agric. Food Chem. , 49 : 5356 – 5363 .
- Baker , A. J.M. , Brooks , R. and Reeves , R. 1988 . Growing for gold…and copper…and zinc . New Sci. , 117 : 44 – 48 .
- Baker , A. J.M. , McGrath , S. P. , Reeves , R. D. and Smith , J. A.C. 2000 . “ Metal hyperaccumulator plants: A review of the ecology and physiology of a biochemical resource for phytoremediation of metal-polluted soils ” . In Phytoremediation of Contaminated Soil and Water , Edited by: Terry , N. and Bañuelos , G. 85 – 107 . Florida : Lewis Publishers .
- Baker , A. J.M. , Reeves , R. D. and Hajar , A. S.M. 1994 . Heavy metal accumulation and tolerance in British populations of the metallophyte Thlaspi caerulescens J. and C. Presl (Brassicaceae) . New Phytol. , 127 : 61 – 68 .
- Baker , A. J.M. and Whiting , S. N. 2002 . In search of the Holy Grail: A further step in understanding metal hyperaccumulation? . New Phytol. , 155 : 1 – 4 .
- Barrutia , O. , Epelde , L. , García-Plazaola , J. I. , Garbisu , C. and Becerril , J. M. 2009 . Phytoextraction potential of two Rumex acetosa L. accessions collected from metalliferous and non-metalliferous sites: Effect of fertilization . Chemosphere , 74 : 259 – 264 .
- Basta , N. and Gradwohl , R. 2000 . Estimation of Cd, Pb and Zn bioavailability in smelter-contaminated soils by a sequential extraction procedure . Soil Sed. Contam. , 9 : 149 – 164 .
- Bennett , L. E. , Burkhead , J. L. , Hale , K. L. , Terry , N. , Pilon , M. and Pilon-Smits , E. A.H. 2003 . Analysis of transgenic Indian mustard plants for phytoremediation of metal-contaminated mine tailings . J. Environ. Qual. , 32 : 432 – 440 .
- Berg , G. 2009 . Plant-microbe interactions promoting plant growth and health: perspectives for controlled use of microorganisms in agriculture . Appl. Microbiol. Biotechnol. , 84 : 11 – 18 .
- Bert , V. , Bonnin , I. , Saumitou-Laprade , P. , de Laguérie , P. and Petit , D. 2002 . Do Arabidopsis halleri from nonmetallicolous populations accumulate zinc and cadmium more effectively than those from metallicolous populations? . New Phytol. , 155 : 47 – 57 .
- Borowiec , M. , Hoffmann , K. and Hoffmann , J. 2009 . The determination of the degree of zinc complexation by chelating agents with differential pulse voltammetry . Int. J. Environ. Anal. Chem. , 89 : 717 – 725 .
- Brooks , R. R. 1998 . Plants that hyperaccumulate heavy metals: Their role in phytoremediation, microbiology, archaeology, mineral exploration and phytomining , New York , NY : CAB International .
- Çakmakçi , R. , Dönmez , F. , Aydm , A. and Şahin , F. 2006 . Growth promotion of plants by plant growth promoting rhizobacteria under greenhouse and two different field soil conditions . Soil Biol. Biochem. , 38 : 1482 – 1487 .
- Cao , L. X. , Jiang , M. , Zeng , Z. R. , Du , A. X. , Tan , H. M. and Liu , Y. H. 2008 . Trichoderma atroviride F6 improves phytoextraction efficiency of mustard (Brassica juncea (L.) Coss. var. foliosa Bailey) in Cd, Ni contaminated soils . Chemosphere , 71 : 1769 – 1773 .
- Catherine , S. , Christophe , S. and Louis , M. J. 2006 . Response of Thlaspi caerulescens to nitrogen, phosphorus and sulfur fertilization . Int. J. Phytoremed. , 8 : 149 – 161 .
- Chaney , R. L. 1983 . “ Plant uptake of inorganic waste constituents ” . In Land treatment of hazardous wastes , Edited by: Parr , J. F. , Marsh , P. B. and Kla , J. M. 50 – 76 . Park Ridge , NJ : Noyes Data Corporation .
- Chaney , R. L. , Angle , J. S. , Broadhurst , C. L. , Peters , C. A. , Tappero , R. V. and Sparks , D. L. 2007 . Improved understanding of hyperaccumulation yields commercial phytoextraction and phytomining technologies . J. Environ. Qual. , 36 : 1429 – 1443 .
- Chen , H. and Cutright , T. 2001 . EDTA and HEDTA effects on Cd, Cr, and Ni uptake by Helianthus annuus . Chemosphere , 45 : 21 – 28 .
- Cherian , S. and Oliveira , M. M. 2005 . Transgenic plants in phytoremediation: Recent advances and new possibilities . Environ. Sci. Technol. , 39 : 9377 – 9390 .
- Chlopecka , A. 1996 . Assessment of form of Cd, Zn and Pb in contaminated calcareous and gleyed soils in Southwest Poland . Sci. Total Environ. , 188 : 253 – 262 .
- Ciura , J. , Poniedziałek , M. , Sękara , A. and Jędrszczyk , E. 2005 . The possibility of using crops as metal phytoremediants . Pol. J. Environ. Stud. , 14 : 17 – 22 .
- Cui , S. , Zhou , Q. X. and Chao , L. 2007 . Potential hyperaccumulation of Pb, Zn, Cu and Cd in endurant plants distributed in an old smeltery, northeast China . Environ. Geol. , 51 : 1043 – 1048 .
- Cui , Y. S. , Dong , Y. T. , Li , H. F. and Wang , Q. R. 2004 . Effect of elemental sulphur on solubility of soil heavy metals and their uptake by maize . Environ. Int. , 30 : 323 – 328 .
- Culbard , E. B. , Thornton , I. , Watt , J. , Weatley , M. , Moorcraft , S. and Thompson , M. 1988 . Metal contamination in British suburban dusts and soils . J. Environ. Qual. , 17 : 226 – 23 .
- Cunningham , S. D. and Ow , D. W. 1996 . Promises and prospects of phytoremediation . Plant Physiol. , 110 : 715 – 719 .
- dal Corso , G. , Borgato , L. and Furini , A. 2005 . In vitro plant regeneration of the heavy metal tolerant and hyperaccumulator Arabidopsis halleri (Brassicaceae) . Plant Cell Tissue Organ Cult. , 82 : 267 – 270 .
- Dell’Amico , E. , Cavalca , L. and Andreoni , V. 2005 . Analysis of rhizobacterial communities in perennial Graminaceae from polluted water meadow soil, and screening of metal-resistant, potentially plant growth-promoting bacteria . FEMS Microbial. Ecol. , 52 : 153 – 162 .
- Demirezen , D. and Aksoy , A. 2006 . Heavy metal levels in vegetables in Turkey are within safe limits for Cu, Zn, Ni and exceeded for Cd and Pb . J. Food Qual. , 29 : 252 – 265 .
- Díaz-Pérez , J. C. 2009 . Root zone temperature, plant growth and yield of broccoli [Brassica oleracea (Plenck) var. italica] as affected by plastic film mulches . Scientia Hort. , 123 : 156 – 163 .
- Dickinson , N. M. , Baker , A. J.M. , Doronila , A. , Laidlaw , S. and Reeves , R. D. 2009 . Phytoremediation of inorganics: Realism and synergies . Int. J. Phytoremed. , 11 : 97 – 114 .
- Dickinson , N. M. and Pulford , I. D. 2005 . Cadmium phytoextraction using short-rotation coppice Salix: The evidence trail . Environ. Int. , 31 : 609 – 613 .
- do Nascimento , C. W. , Amarasiriwardena , D. and Xing , B. 2006 . Comparison of natural organic acids and synthetic chelates at enhancing phytoextraction of metals from a multi-metal contaminated soil . Environ. Pollut. , 140 : 114 – 123 .
- Dos Santos Utmazian , M. N. and Wenzel , W. W. 2007 . Cadmium and zinc accumulation in willow and poplar species grown on polluted soils . J. Plant Nutr. Soil Sci. , 170 : 265 – 272 .
- Doty , S. L. 2008 . Enhancing phytoremediation through the use of transgenics and endophytes . New Phytol. , 179 : 318 – 333 .
- Doumett , S. , Lamperi , L. , Checchini , L. , Azzarello , E. , Mugnai , S. , Mancuso , S. , Petruzzelli , G. and Del Bubba , M. 2008 . Heavy metal distribution between contaminated soil and Paulownia tomentosa, in a pilot-scale assisted phytoremediation study: Influence of different complexing agents . Chemosphere , 72 : 1481 – 1490 .
- Ebbs , S. D. and Kochian , L. V. 1998 . Phytoextraction of zinc by oat (Avena sativa), barley (Hordeum vulgare), and Indian mustard (Brassica juncea) . Environ. Sci. Technol. , 32 : 802 – 806 .
- Ercolia , L. , Mariottib , M. , Masonib , A. and Bonaria , E. 1999 . Effect of irrigation and nitrogen fertilization on biomass yield and efficiency of energy use in crop production of Miscanthus . Field Crop. Res. , 63 : 3 – 11 .
- Ernst , W. H.O. 1996 . Bioavailability of heavy metals and decontamination of soils by plants . Appl. Geochem. , 11 : 163 – 167 .
- Ernst , W. H.O. 2005 . Phytoextraction of mine wastes: Options and impossibilities . Chem. Erde-Geochem. , 65 : 29 – 42 .
- European Union . 2000 . Working document on sludge, 3rd draft, ENV.E.3/LM , Brussels , , Belgium : EU .
- Evangelou , M. W.H. , Bauer , U. , Ebel , M. and Schaeffer , A. 2007 . The influence of EDDS and EDTA on the uptake of heavy metals of Cd and Cu from soil with tobacco Nicotiana tabacum . Chemosphere , 68 : 345 – 353 .
- Farinati , S. , DalCorso , G. , Varotto , S. and Furini , A. 2010 . The Brassica juncea BjCdR15, an ortholog of Arabidopsis TGA3, is a regulator of cadmium uptake, transport and accumulation in shoots and confers cadmium tolerance in transgenic plants . New Phytol. , 185 : 964 – 978 .
- Food and Agriculture Organization of the United Nations . 2009 . State of food insecurity in the world 2009 , Rome , , Italy : FAO .
- Fischerová , Z. , Tlustoš , P. , Száková , J. and Šichorová , K. 2006 . A comparison of phytoremediation capability of selected plant species for given trace elements . Environ. Pollut. , 144 : 93 – 100 .
- Fritioff , Å. , Kautsky , L. and Greger , M. 2005 . Influence of temperature and salinity on heavy metal uptake by submersed plants . Environ. Pollut. , 133 : 265 – 274 .
- Fuksová , Z. , Száková , J. and Tlustoš , P. 2009 . Effects of co-cropping on bioaccumulation of trace elements in Thlaspi caerulescens and Salix dasyclados . Plant Soil Environ. , 55 : 461 – 467 .
- Gadd , G. M. 2004 . Microbial influence on metal mobility and application for bioremediation . Geoderma , 122 : 109 – 119 .
- Ghosh , M. and Singh , S. P. 2005 . A comparative study of cadmium phytoextraction by accumulator and weed species . Environ. Pollut. , 133 : 365 – 371 .
- Gove , B. , Hutchinson , J. J. , Young , S. D. , Craigon , J. and McGrath , S. P. 2002 . Uptake of metals by plants sharing rhizosphere with the hyperaccumulator Thlaspi caerulescens . Int. J. Phytoremed. , 4 : 267 – 281 .
- Grandlic , C. J. , Mendez , M. O. , Chorover , J. , Machado , B. and Maier , R. M. 2008 . Plant grow-promoting bacteria for phytostabilization of mine tailings . Environ. Sci. Technol. , 42 : 2079 – 2084 .
- Grandlic , C. J. , Palmer , M. W. and Maier , R. M. 2009 . Optimization of plant-promoting bacteria-assisted phytostablization of mine tailings . Soil Biol. Biochem. , 41 : 1734 – 1740 .
- Grispen , V. M.J. , Nelissen , H. J.M. and Verkleij , J. A.C. 2006 . Phytoextration with Brassica napus L.: A tool for sustainable management of heavy metal contaminated soils . Environ. Pollut. , 144 : 77 – 83 .
- Grundler , O. J. , van der Steen , A. T. M. and Wilmot , J. 2005 . “ Overview of the European risk assessment on EDTA ” . In Biogeochemistry of chelating agents; ACS Symposium Series Vol. 910 , Edited by: Nowack , B. and VanBriesen , J. M. 336 – 347 . Washington , DC : American Chemical Society .
- Haghiri , F. 1973 . Cadmium uptake by plants . J. Environ. Qual. , 2 : 93 – 95 .
- Hammer , D. and Keller , C. 2003 . Phytoextraction of Cd and Zn with Thlaspi caerulescens in field trials . Soil Use Manag. , 19 : 144 – 149 .
- Hammer , D. , Kayser , A. and Keller , C. 2003 . Phytoextraction of Cd and Zn with Salix viminalis in field trials . Soil Use Manag. , 19 : 187 – 192 .
- Hanikenne , M. , Talke , I. N. , Haydon , M. J. , Lanz , C. , Nolte , A. , Motte , P. , Kroymann , J. , Weigel , D. and Kramer , U. 2008 . Evolution of metal hyperaccumulation required cis-regulatory changes and triplication of HMA4 . Nature , 453 : 391 – 395 .
- He , L. Y. , Chen , Z. J. , Ren , G. D. , Zhang , Y. F. , Qian , M. and Sheng , X. F. 2009 . Increased cadmium and lead uptake of a cadmium hyperaccumulator tomato by cadmium-resistant bacteria . Ecotoxicol. Environ. Saf. , 72 : 1343 – 1348 .
- Hernández-Allica , J. , Becerril , J. M. and Garbisu , C. 2008 . Assessment of the phytoextraction potential of high biomass crop plants . Environ. Pollut. , 152 : 32 – 40 .
- Huang , J. W. , Chen , J. J. , Berti , W. R. and Cunningham , S. D. 1997 . Phytoremediation of lead-contaminated soils: Role of synthetic chelates in lead phytoextraction . Environ. Sci. Technol. , 31 : 800 – 805 .
- Ibaraki , T. , Kuroyanagi , N. and Murakami , M. 2009 . Practical phytoextraction in cadmium-polluted paddy fields using a high cadmium accumulating rice plant cultured by early drainage of irrigation water . Soil Sci. Plant Nutr. , 55 : 421 – 427 .
- Idris , R. , Trifonova , R. , Puschenreiter , M. , Wenzel , W. W. and Sessitsch , A. 2004 . Bacterial communities associated with flowering plants of the Ni hyperaccumulator Thlaspi goesingense . Appl. Environ. Microbiol. , 70 : 2667 – 2677 .
- Ikeda , M. , Ezaki , T. , Tsukahara , T. and Moriguchi , J. 2004 . Dietary cadmium intake in polluted and non-polluted area in Japan in past and in the present . Int. Occup. Environ. Health , 77 : 227 – 234 .
- Janoušková , M. , Pavlíková , D. , Macek , T. and Vosátka , M. 2005 . Arbuscular mycorrhiza decreases cadmium phytoextraction by transgenic tobacco with inserted metallothionein . Plant Soil , 272 : 29 – 40 .
- Jensen , J. K. , Holm , P. E. , Nejrup , J. , Larsen , M. B. and Borggaard , O. K. 2009 . The potential of willow for remediation of heavy metal polluted calcareous urban soils . Environ. Pollut. , 157 : 931 – 937 .
- Ji , P. H. , Sun , T. H. , Song , Y. F. , Ackland , M. L. and Liu , Y. 2011 . Strategies for enhancing the phytoremediation of cadmium-contaminated agricultural soils by Solanum nigrum L . Environ. Pollut. , 159 : 762 – 768 .
- Jiang , C. A. , Wu , Q. T. , Sterckeman , T. , Schwartz , C. , Sirguey , C. , Ouvrard , S. , Perriguey , J. and Morel , J. L. 2010 . Co-planting can phytoextract similar amounts of cadmium and zinc to mono-cropping from contaminated soils . Ecol. Engineer. , 36 : 391 – 395 .
- Karpenstein-Machan , M. and Stuelpnagel , R. 2000 . Biomass yield and nitrogen fixation of legumes monocropped and intercropped with rye and rotation effects on a subsequent maize crop . Plant Soil , 218 : 215 – 232 .
- Kawashima , C. G. , Noji , M. , Nakamura , M. , Ogra , Y. , Suzuki , K. T. and Saito , K. 2004 . Heavy metal tolerance of transgenic tobacco plants over-expressing cysteine synthase . Biotech. Lett. , 26 : 153 – 157 .
- Kayser , A. , Wenger , K. , Keller , A. , Attinger , W. , Felix , H. R. , Gupta , S. K. and Schulin , R. 2000 . Enhancement of phytoextraction of Zn, Cd, and Cu from calcareous soil: The use of NTA and sulfur amendments . Environ. Sci. Technol. , 34 : 1778 – 1783 .
- Keller , C. , Hammer , D. , Kayser , A. , Richner , W. , Brodbeck , M. and Sennhauser , M. 2003 . Root development and heavy metal phytoextraction efficiency: Comparison of different plant species in the field . Plant Soil , 249 : 67 – 81 .
- Keller , C. , Ludwig , C. , Davoli , F. and Wochele , J. 2005 . Thermal treatment of metal-enriched biomass produced from heavy metal phytoextraction . Environ. Sci. Technol. , 39 : 3359 – 3367 .
- Khan , M. S. , Zaidi , A. and Aamil , M. 2002 . Biocontrol of fungal pathogens by the use of plant growth promoting rhizobacteria and nitrogen fixing microorganisms . Ind. J. Bot. Soc. , 81 : 255 – 263 .
- Khan , M. S. , Zaidi , A. , Wani , P. A. and Oves , M. 2009 . Role of plant growth promoting rhizobacteria in the remediation of metal contaminated soils . Environ. Chem. Lett. , 7 : 1 – 19 .
- Komárek , M. , Tlustoš , P. , Száková , J. and Chrastný , V. 2008 . The use of poplar during a two-year induced phytoextraction of metals from contaminated agricultural soils . Environ. Pollut. , 151 : 27 – 38 .
- Komárek , M. , Tlustoš , P. , Száková , J. , Chrastný , V. and Ettler , V. 2007 . The use of maize and poplar in chelant-enhanced phytoextraction of lead from contaminated agricultural soils . Chemosphere , 67 : 640 – 651 .
- Koopmans , G. F. , Römkens , P. F. , Fokkema , M. J. , Song , J. , Luo , Y. M. , Japenga , J. and Zhao , F. J. 2008 . Feasibility of phytoextraction to remediate cadmium and zinc contaminated soils . Environ. Pollut. , 156 : 905 – 914 .
- Lane , T. W. and Morel , F. M.M. 2000 . A biological function for Cd in marine diatoms . Proc. Natl. Acad. Sci. USA. , 97 : 4627 – 4631 .
- Lasat , M. 2000 . Phytoextraction of metals from contaminated soil: A review of plant/soil/metal interaction and assessment of pertinent agronomic issues . J. Hazard. Sub. Res. , 2 : 5 – 25 .
- Lee , Y. Z. , Suzuki , S. , Kawada , T. , Wang , J. , Koyama , H. , Rivai , I. F. and Herawati , N. 1999 . Content of cadmium in carrots compared with rice in Japan . Bull. Environ. Contam. Toxicol. , 63 : 711 – 719 .
- Leštan , D. , Luo , C. L. and Li , X. D. 2008 . The use of chelating agents in the remediation of metal-contaminated soils: A review . Environ. Pollut. , 153 : 3 – 13 .
- Leyval , C. , Turnau , K. and Haselwandter , K. 1997 . Effect of heavy metal pollution on mycorrhizal colonization and function: physiological, ecological and applied aspects . Mycorrhiza , 7 : 139 – 153 .
- Li , J. T. , Liao , B. , Dai , Z. Y. , Zhu , R. and Shu , W. S. 2009a . Phytoextraction of Cd-contaminated soil by carambola (Averrhoa carambola) in field trials . Chemosphere , 76 : 1233 – 1239 .
- Li , J. T. , Liao , B. , Lan , C. Y. , Ye , Z. H. , Baker , A. J.M. and Shu , W. S. 2010 . Cadmium accumulation in a high-biomass tropical tree (Averrhoa carambola) and its potential for phytoextraction . J. Environ. Qual. , 39 : 1261 – 1268 .
- Li , J. T. , Qiu , J. W. , Wang , X. W. , Zhong , Y. , Lan , C. Y. and Shu , W. S. 2006 . Cadmium contamination in orchard soils and fruit trees and its potential health risk in Guangzhou, China . Environ. Pollut. , 143 : 159 – 165 .
- Li , N. Y. , Li , Z. A. , Zhuang , P. , Zou , B. and McBride , M. 2009b . Cadmium uptake from soil by maize with intercrops . Water Air Soil Pollut. , 199 : 45 – 56 .
- Licht , L. A. and Isebrands , J. G. 2005 . Linking phytoremediated pollutant removal to biomass economic opportunities . Biomass Bioenerg. , 28 : 203 – 218 .
- Liu , D. , Islam , E. , Li , T. Q. , Yang , X. E. , Jin , X. F. and Mahmood , Q. 2008 . Comparison of synthetic chelators and low molecular weight organic acids in enhancing phytoextraction of heavy metals by two ecotypes of Sedum alfredii Hance . J. Hazard. Mater. , 153 : 114 – 122 .
- Liu , L. , Wu , L. H. , Li , N. , Cui , L. Q. , Li , Z. , Jiang , J. P. , Jiang , Y. G. , Qiu , X. Y. and Luo , Y. M. 2009 . Effect of planting densities on yields and zinc and cadmium uptake by Sedum plumbizincicola . Chin. J. Environ. Sci. , 30 : 3422 – 3426 .
- Liu , W. , Shu , W. S. and Lan , C. Y. 2004 . Viola baoshanensis, a plant that hyperaccumulates cadmium . Chin. Sci. Bull. , 49 : 29 – 32 .
- Liu , X. M. , Wu , Q. T. and Banks , M. K. 2005 . Effect of simultaneous establishment of Sedum alfredii and Zea mays on heavy metal accumulation in plants . Int. J. Phytoremed. , 7 : 43 – 53 .
- Lombi , E. , Zhao , F. J. , Dunham , S. J. and McGrath , S. P. 2000 . Cadmium accumulation in populations of Thlaspi caerulescens and Thlaspi goesingense . New Phytol. , 145 : 11 – 20 .
- Lombi , E. , Zhao , F. J. , Dunham , S. J. and McGrath , S. P. 2001 . Phytoremediation of heavy metal-contaminated soils: Natural hyperaccumulation versus chemically enhanced phytoextraction . J. Environ. Qual. , 30 : 1919 – 1926 .
- Luo , C. L. , Shen , Z. G. , Baker , A. J.M. and Li , X. D. 2006 . A novel strategy using biodegradable EDDS for the chemically enhanced phytoextraction of soils contaminated with heavy metals . Plant Soil , 285 : 67 – 80 .
- Luo , C. L. , Shen , Z. G. and Li , X. D. 2005 . Enhanced phytoextraction of Cu, Pb, Zn and Cd with EDTA and EDDS . Chemosphere , 59 : 1 – 11 .
- Luo , C. L. , Shen , Z. G. and Li , X. D. 2007 . Plant uptake and the leaching of metals during the hot EDDS-enhanced phytoextraction process . Int. J. Phytoremed. , 9 : 181 – 196 .
- Ma , L. Q. and Rao , G. N. 1997 . Chemical fractionation of cadmium, copper, nickel, and zinc in contaminated soils . J. Environ. Qual. , 26 : 259 – 264 .
- Malhi , S. S. , Johnston , A. M. , Schoenau , J. J. , Wang , Z. H. and Vera , C. L. 2007 . Seasonal biomass accumulation and nutrient uptake of canola, mustard, and flax on a black chernozem soil in Saskatchewan . J. Plant Nutr. , 30 : 641 – 658 .
- Marschner , H. 1995 . Mineral nutrition of higher plants , London , , England : Academic Press .
- Maxted , A. P. , Black , C. R. , West , H. M. , Crout , N. M.J. , McGrath , S. P. and Young , S. D. 2007 . Phytoextraction of cadmium and zinc by Salix from soil historically amended with sewage sludge . Plant Soil , 290 : 157 – 172 .
- McGauley , G. N. and Way , M. O. 2002 . Drain and harvest timing affects on rice grain drying and whole-milled grain . Field Crop. Res. , 74 : 163 – 172 .
- McGrath , S. P. , Lombi , E. , Gray , C. W. , Caille , N. , Dunham , S. J. and Zhao , F. J. 2006 . Field evaluation of Cd and Zn phytoextraction potential by the hyperaccumulators Thlaspi caerulescens and Arabidopsis halleri . Environ. Pollut. , 141 : 115 – 125 .
- McGrath , S. P. and Zhao , F. J. 2003 . Phytoextraction of metals and metalloids from contaminated soils . Curr. Opin. Biotechnol. , 14 : 277 – 282 .
- McLaughlin , M. J. , Parker , D. R. and Clarke , J. M. 1999 . Metal and micronutrients – food safety issues . Field Crop. Res. , 60 : 143 – 163 .
- Meers , E. , Hopgood , M. , Lesage , E. , Vervaeke , P. , Tack , F. M.G. and Verloo , M. G. 2004 . Enhanced phytoextraction: In search of EDTA alternatives . Int. J. Phytoremed. , 6 : 95 – 109 .
- Meers , E. , Ruttens , A. , Hopgood , M. J. , Samson , D. and Tack , F. M.G. 2005a . Comparison of EDTA and EDDS as potential soil amendments for enhanced phytoextraction of heavy metals . Chemosphere , 58 : 1011 – 1022 .
- Meers , E. , Unamuno , V. , Vandegehuchte , M. , Vanbroekhoven , K. , Geebelen , W. , Samson , R. , Vangronsveld , J. , Diels , L. , Ruttens , A. , Du Laing , G. and Tack , F. 2005b . Soil-solution speciation of Cd as affected by soil characteristics in unpolluted and polluted soils . Environ. Toxicol. Chem. , 24 : 499 – 509 .
- Meers , E. , Vandecasteele , B. , Ruttens , A. , Vangronsveld , J. and Tack , F. M.G. 2007 . Potential of five willow species (Salix spp.) for phytoextraction of heavy metals . Environ. Exp. Bot. , 60 : 57 – 68 .
- Mertens , J. , Vervaeke , P. , Meers , E. and Tack , F. M.F. 2006 . Seasonal changes of metals in willow (Salix sp.) stands for phytoremediation on dredged sediment . Environ. Sci. Technol. , 40 : 1962 – 1968 .
- Munn , J. , January , M. and Cutright , T. J. 2008 . Greenhouse evaluation of EDTA effectiveness at enhancing Cd, Cr, and Ni uptake in Helianthus annuus and Thlaspi caerulescens . J. Soils Sed. , 8 : 116 – 122 .
- Murakami , M. , Ae , N. and Ishikawaa , S. 2007 . Phytoextraction of cadmium by rice (Oryza sativa L.), soybean (Glycine max (L.) Merr.), and maize (Zea mays L.) . Environ. Pollut. , 145 : 96 – 103 .
- Murakami , M. , Ae , N. , Ishikawa , S. , Ibaraki , T. and Ito , M. 2008 . Phytoextraction by a high-Cd-accumulating rice: Reduction of Cd content of soybean seeds . Environ. Sci. Technol. , 42 : 6167 – 6172 .
- Murakami , M. , Nakagawa , F. , Ae , N. , Ito , M. and Arao , T. 2009 . Phytoextraction by rice capable of accumulating Cd at high levels: Reduction of Cd content of rice grain . Environ. Sci. Technol. , 43 : 5878 – 5883 .
- Nehnevajova , E. , Herzig , R. , Federer , G. and Erismann , K.-H. 2005 . Screening of sunflower cultivars for metal phytoextraction in a contaminated field prior to mutagenesis . Int. J. Phytoremed. , 7 : 337 – 349 .
- Neugschwandtner , R. W. , Tlustoš , P. , Komárek , M. and Száková , J. 2008 . Phytoextraction of Pb and Cd from a contaminated agricultural soil using different EDTA application regimes: Laboratory versus field scale measures of efficiency . Geoderma , 144 : 446 – 454 .
- Nishiyama , Y. , Yanai , J. and Kosaki , T. 2005 . Potential of Thlaspi caerulescens for dadmium phytoremediation: Comparison of two representative soil types in Japan under different planting frequencies . Soil Sci. Plant Nutr. , 51 : 827 – 834 .
- Nowack , B. , Schulin , R. and Robinson , B. H. 2006 . Critical assessment of chelant-enhanced metal phytoextraction . Environ. Sci. Technol. , 40 : 5225 – 5232 .
- Papoyan , A. and Kochian , L. V. 2004 . Identification of Thlaspi caerulescens genes that may be involved in heavy metal hyperaccumulation and tolerance. Characterization of a novel heavy metal transporting ATPase . Plant Physiol. , 136 : 3814 – 3823 .
- Paulson , M. , Bardos , P. , Harmsen , J. , Wilczek , J. , Barton , M. and Edwards , D. 2003 . The practical use of short rotation coppice in land restoration . Land Contam. Reclam. , 11 : 323 – 338 .
- Peñalosa , J. M. , Carpena , R. O. , Vázquez , S. , Agha , R. , Granado , A. , Sarro , M. J. and Esteban , E. 2007 . Chelate-assisted phytoextraction of heavy metals in a soil contaminated with a pyritic sludge . Sci. Total Environ. , 378 : 199 – 204 .
- Perronnet , K. , Schwartz , C. , Gérard , E. and Morel , J. L. 2000 . Availability of cadmium and zinc accumulated in the leaves of Thlaspi caerulescens incorporated into soil . Plant Soil , 227 : 257 – 263 .
- Pietz , R. I. , Vetter , R. J. , Masarik , D. and McFee , W. W. 1978 . Zinc and cadmium contents of agricultural soils and corn in Northwestern Indiana . J. Environ. Qual. , 7 : 381 – 385 .
- Pongrac , P. , Zhao , F. J. , Razinger , J. , Zrimec , A. and Regvar , M. 2009 . Physiological responses to Cd and Zn in two Cd/Zn hyperaccumulating Thlaspi species . Environ. Exp. Bot. , 66 : 479 – 486 .
- Prokop , Z. , Cupr , P. , Zlevorova-Zlamalikova , V. , Komarek , J. , Dusek , L. and Holoubek , I. 2003 . Mobility, bioavailability, and toxic effects of cadmium in soil samples . Environ. Res. , 91 : 119 – 126 .
- Pulford , I. D. and Watson , C. 2003 . Phytoredediation of heavy metal-contaminated land by trees: A review . Environ. Int. , 29 : 529 – 540 .
- Quartacci , M. F. , Argilla , A. , Baker , A. J.M. and Navari-Izzo , F. 2006 . Phytoextraction of metals from a multiply contaminated soil by Indian mustard . Chemosphere , 63 : 918 – 925 .
- Rajkumar , M. and Freitas , H. 2008 . Influence of metal resistant-plant growth-promoting bacteria on the growth of Ricinus communis in soil contaminated with heavy metals . Chemosphere , 71 : 834 – 842 .
- Ramah , K. , Santhi , P. and Ponnuswamy , K. 2009 . Irrigation scheduling and water use efficiency in maize (Zea mays L.) based cropping system under drip fertigation . Crop Res. , 38 : 15 – 20 .
- Raskin , I. , Smith , R. D. and Salt , D. E. 1997 . Phytoremediation of metals: Using plants to remove pollutants from the environment . Curr. Opin. Biotechnol. , 8 : 221 – 226 .
- Robinson , B. H. , Fernández , J.-E. , Madejón , P. , Marañón , T. , Murillo , J. M. , Green , S. and Clothier , B. 2003 . Phytoextraction: An assessment of biogeochemical and economic viability . Plant Soil , 249 : 117 – 125 .
- Robinson , B. H. , Schulin , R. , Nowack , B. , Roulier , S. , Menon , M. , Clothier , B. E. , Green , S. R. and Mills , T. M. 2006 . Phytoremediation for the management of metal flux in contaminated sites . For. Snow Landsc. Res. , 80 : 221 – 234 .
- Rugh , C. L. , Senecoff , J. F. , Meagher , R. B. and Merkle , S. A. 1998 . Development of transgenic yellow poplar for mercury phytoremediation . Nature Biotechnol. , 16 : 925 – 928 .
- Salt , D. E. , Blaylock , M. , Kumar , N. P.B.A. , Dushenkov , V. , Ensley , B. , Chet , I. and Raskin , I. 1995 . Phytoremediation: A novel strategy for the removal of toxic metals from the environment using plants . Nature Biotechnol. , 13 : 468 – 474 .
- Sanità di Toppi , L. and Gabbrielli , R. 1999 . Response to cadmium in higher plant . Environ. Exp. Bot. , 41 : 105 – 130 .
- Santos , F. S. , Hernández-Allica , J. , Becerril , J. M. , Amaral-Sobrinho , N. , Mazur , N. and Carlos , G. 2006 . Chelate-induced phytoextraction of metal polluted soils with Brachiaria decumbens . Chemosphere , 65 : 43 – 50 .
- Saraswat , S. and Rai , J. P.N. 2011 . Prospective application of Leucaena Leucocephala for phytoextraction of Cd and Zn and nitrogen fixation in metal polluted soils . Intern. J. Phytoremed. , 13 : 271 – 288 .
- Schmidt , U. 2003 . Enhancing phytoextraction: The effect of chemical soil manipulation on mobility, plant accumulation, and leaching of heavy metals . J. Environ. Qual. , 32 : 1939 – 1954 .
- Schwartz , C. , Echevarria , G. and Morel , J. L. 2003 . Phytoextraction of cadmium with Thlaspi caerulescens . Plant Soil , 249 : 27 – 35 .
- Schwartz , C. , Sirguey , C. , Peronny , S. , Reeves , R. D. , Bourgaud , F. and Morel , J. L. 2006 . Testing of outstanding individuals of Thlaspi caerulescens for cadmium phytoextraction . Int. J. Phytoremed. , 8 : 339 – 357 .
- Sell , J. , Kayser , A. , Schulin , R. and Brunner , I. 2005 . Contribution of ectomycorrhizal fungi to cadmium uptake of poplars and willows from a heavily polluted soil . Plant Soil , 277 : 245 – 253 .
- Selvam , A. and Wong , J. W.C. 2009 . Cadmium uptake potential of Brassica napus cocropped with Brassica parachinensis and Zea mays . J. Hazard. Mater. , 167 : 170 – 178 .
- Shen , Z. G. , Li , X. D. , Wang , C. C. , Chen , H. M. and Chua , H. 2002 . Lead phytoextraction from contaminated soil with high biomass plant species . J. Environ. Qual. , 31 : 1893 – 1900 .
- Sheng , X. F. and Xia , J. J. 2006 . Improvement of rape (Brassica napus) plant growth and cadmium uptake by cadmium-resistant bacteria . Chemosphere , 64 : 1036 – 1042 .
- Simmons , R. W. , Pongsakul , P. , Saiyasitpanich , D. and Klinphoklap , S. 2005 . Elevated levels of cadmium and zinc in paddy soils and elevated levels of cadmium in rice grain downstream of a zinc mineralized area in Thailand: Implications for public health . Environ. Geochem. Health , 27 : 501 – 511 .
- Singh , B. R. and Subramaniam , V. 1997 . Phosphorus supplying capacity of heavily fertilized soils II. Dry matter yield of successive crops and phosphorus uptake at different temperatures . Nutr. Cycl. Agroecosyst. , 47 : 123 – 134 .
- Smeets , K. , Ruytinx , J. , Semane , B. , van Belleghem , F. , Remans , T. , van Sanden , S. , Vangronsveld , J. and Cuypers , A. 2008 . Cadmium-induced transcriptional and enzymatic alterations related to oxidative stress . Environ. Exp. Bot. , 63 : 1 – 8 .
- Smith , R. H. 2000 . Plant tissue culture: Techniques and experiments () , 2nd ed. , New York , NY : Academic Press .
- Song , W. Y. , Sohn , E. J. , Martinoia , E. , Lee , Y. J. , Yang , Y. Y. , Jasinski , M. , Forestier , C. , Hwang , I. and Lee , Y. 2003 . Engineering tolerance and accumulation of lead and cadmium in transgenic plants . Nature Biotechnol. , 21 : 914 – 919 .
- Soriano , M. A. and Fereres , E. 2003 . Use of crops for in situ phytoremediation of polluted soils following a toxic flood from a mine spill . Plant Soil , 256 : 253 – 264 .
- Su , D. C. and Wong , J. W.C. 2004 . Phytoremediation potential of oilseed rape (Brassica juncea) for cadmium contaminated soil . Bull. Environ. Contam. Toxicol. , 72 : 991 – 998 .
- Sun , B. , Zhao , F. J. , Lombi , E. and McGrath , S. P. 2001 . Leaching of heavy metals from contaminated soils using EDTA . Environ. Pollut. , 113 : 111 – 120 .
- Sun , L. N. , Niu , Z. X. and Sun , T. H. 2007 . Effects of amendments of N, P, Fe on phytoextraction of Cd, Pb, Cu, and Zn in soil of Zhangshi by mustard, cabbage, and sugar beet . Environ. Toxicol. , 22 : 565 – 571 .
- Sun , Y. B. , Zhou , Q. X. , An , J. , Liu , W. T. and Liu , R. 2009 . Chelator-enhanced phytoextraction of heavy metals from contaminated soil irrigated by industrial wastewater with the hyperaccumulator plant (Sedum alfredii Hance) . Geoderma , 150 : 106 – 112 .
- Tandy , S. , Schulin , R. and Nowack , B. 2006 . Uptake of metals during chelant-assisted phytoextraction with EDDS related to the solubilized metal concentration . Environ. Sci. Technol. , 40 : 2753 – 2758 .
- Tang , X. Y. , Zhu , Y. G. , Cui , Y. S. , Duan , J. and Tang , L. 2006 . The effect of ageing on the bioaccessibiliy and fractionation of cadmium in some typical soils of China . Environ. Int. , 32 : 682 – 689 .
- Thayalakumaran , T. , Robinson , B. H. , Vogeler , I. , Scotter , D. R. , Clothier , B. E. and Percival , H. J. 2003 . Plant uptake and leaching of copper during EDTA-enhanced phytoremediation of repacked and undisturbed soil . Plant Soil , 254 : 415 – 423 .
- Thewys , T. , Witters , N. , Meers , E. and Vangronsveld , J. 2010b . Economic viability of phytoremediation of a cadmium contaminated agricultural area using energy maize. Part II: Economics of anaerobic digestion of metal contaminated maize in Belgium . Int. J. Phytoremed. , 12 : 663 – 679 .
- Thewys , T. , Witters , N. , van Slycken , S. , Ruttens , A. , Meers , E. , Tack , F. M. and Vangronsveld , J. 2010a . Economic viability of phytoremediation of a cadmium contaminated agricultural area using energy maize. Part I: Effect on the farmer's income . Int. J. Phytoremed. , 12 : 650 – 662 .
- U.S. Department of Health and Human Services . 2005 . Eleventh report on carcinogens , Washington , DC : U.S Department of Health and Human Services .
- Usman , A. R.A. and Mohamed , H. M. 2009 . Effect of microbial inoculation and EDTA on the uptake and translocation of heavy metal by corn and sunflower . Chemosphere , 76 : 893 – 899 .
- van Nevel , L. , Mertens , J. , Oorts , K. and Verheyen , K. 2007 . Phytoextraction of metals from soils: How far from practice? . Environ. Pollut. , 150 : 34 – 40 .
- Vangronsveld , J. , Herzig , R. , Weyens , N. , Boulet , J. , Adriaensen , K. , Ruttens , A. , Thewys , T. , Vassilev , A. , Meers , E. , Nehnevajova , E. , van der Lelie , D. and Mench , M. 2009 . Phytoremediation of contaminated soils and groundwater: Lessons from the field . Environ. Sci. Pollut. Res. , 16 : 765 – 794 .
- Verbruggen , N. , Hermans , C. and Schat , H. 2009 . Mechanisms to cope with arsenic or cadmium excess in plants . Curr. Opin. Plant Biol. , 12 : 364 – 72 .
- Verret , F. , Gravot , A. , Auroy , P. , Leonhardt , N. , David , P. , Nussaume , L. , Vavasseur , A. and Richaud , P. 2004 . Overexpression of AtHMA4 enhances root-to-shoot translocation of zinc and cadmium and plant metal tolerance . FEBS Lett. , 576 : 306 – 312 .
- Vogel-Mikuš , K. , Drobne , D. and Regvar , M. 2005 . Zn, Cd and Pb accumulation and arbuscular mycorrhizal colonisation of pennycress Thlaspi praecox Wulf. (Brassicaceae) from the vicinity of a lead mine and smelter in Slovenia . Environ. Pollut. , 133 : 233 – 242 .
- Vysloužilová , M. , Tlustoš , P. and Száková , J. 2003 . Cadmium and zinc phytoextraction potential of seven clones of Salix spp. planted on heavy metal contaminated soils . Plant Soil Environ. , 49 : 542 – 547 .
- Wang , A. S. , Angle , J. S. , Chaney , R. L. , Delorme , T. A. and Reeves , R. D. 2006 . Soil pH effects on uptake of Cd and Zn by Thlaspi caerulescens . Plant Soil , 281 : 325 – 337 .
- Wang , F. Y. , Lin , X. G. and Yin , R. 2005 . Heavy metal uptake by arbuscular mycorrhizas of Elsholtzia splendens and the potential for phytoremediation of contaminated soil . Plant Soil , 269 : 225 – 232 .
- Watanabe , T. , Shimbo , S. , Moon , C. S. , Zhang , Z. W. and Ikeda , M. 1996 . Cadmium contents in rice samples from various areas in the world . Sci. Total Environ. , 184 : 191 – 196 .
- Wei , S. H. , Zhou , Q. X. and Koval , P. V. 2006 . Flowering stage characteristics of cadmium hyperaccumulator Solanum nigrum L., and their significance to phytoremediation . Sci. Total Environ. , 369 : 441 – 446 .
- Wei , S. H. , Teixeira da Silva , J. A. and Zhou , Q. X. 2008 . Agro-improving method of phytoextracting heavy metal contaminated soil . J. Hazard. Mater. , 150 : 662 – 668 .
- Wei , S. H. , Zhou , Q. X. , Wang , X. , Zhang , K. S. , Guo , G. L. and Ma , L. Q. 2005 . A newly-discovered Cd-hyperaccumulator Solanum nigrum L . Chin. Sci. Bull. , 50 : 33 – 38 .
- Wei , S. H. , Zhou , Q. X. , Xiao , H. , Yang , C. J. , Hu , Y. H. and Ren , L. P. 2009 . Hyperaccumulative property comparision of 24 weed species to heavy metals using a pot culture experiment . Environ. Monit. Assess. , 152 : 299 – 307 .
- Weyens , N. , van der Lelie , D. , Taghavi , S. , Newman , L. and Vangronsveld , J. 2009 . Exploiting plant-microbe partnerships to improve biomass production and remediation . Trends Biotechnol. , 27 : 591 – 598 .
- Whiting , S. N. , de Souza , M. P. and Terry , N. 2001 . Rhizosphere bacteria mobilize Zn for hyperaccumulation by Thlaspi caerulescens . Environ. Sci. Technol. , 35 : 3144 – 3150 .
- Wieshammer , G. , Unterbrunner , R. , Garcia , T. B. , Zivkovic , M. F. , Puschenreiter , M. and Wenzel , W. W. 2007 . Phytoextraction of Cd and Zn from agricultural soils by Salix ssp., and intercropping of Salix caprea and Arabidopsis halleri . Plant Soil , 298 : 255 – 264 .
- World Health Organization . 1992 . Environmental health criteria Vol. 134: Cadmium , Geneva , , Switzerland : WHO .
- Wong , C. K.E. and Cobbett , C. S. 2009 . HMA P-type ATPases are the major mechanism for root-to-shoot Cd translocation in Arabidopsis thaliana . New Phytol. , 181 : 71 – 78 .
- Wong , C. K.E. , Jarvis , R. S. , Sherson , S. M. and Cobbett , C. S. 2009 . Functional analysis of the heavy metal binding domains of the Zn/Cd-transporting ATPase, HMA2, in Arabidopsis thaliana . New Phytol. , 181 : 79 – 88 .
- Wu , C. H. , Bernard , S. M. , Andersen , G. L. and Chen , W. 2009 . Developing microbe-plant interactions for applications in plant-growth promotion and disease control, production of useful compounds, remediation and carbon sequestration . Microb. Biotechnol. , 2 : 428 – 440 .
- Wu , F. Z. , Yang , W. Q. , Zhang , J. and Zhou , L. Q. 2010 . Cadmium accumulation and growth responses of a poplar (Populus deltoids × Populus nigra) in cadmium contaminated purple soil and alluvial soil . J. Hazard. Mater. , 177 : 268 – 273 .
- Wu , Q. T. , Deng , J. C. , Long , X. X. , Morel , J. L. and Schwartz , C. 2006 . Selection of appropriate organic additives for enhancing Zn and Cd phytoextraction by hyperaccumulators . J. Environ. Sci. , 18 : 1113 – 1118 .
- Wu , Q. T. , Wei , Z. B. and Ouyang , Y. 2007 . Phytoextraction of metal-contaminated soil by Sedum alfredii H: Effects of chelator and co-planting . Water Air Soil Pollut. , 180 : 131 – 139 .
- Xie , H. L. , Jiang , R. F. , Zhang , F. S. , McGrath , S. P. and Zhao , F. J. 2009 . Effect of nitrogen form on the rhizosphere dynamics and uptake of cadmium and zinc by the hyperaccumulator Thlaspi caerulescens . Plant Soil , 318 : 205 – 215 .
- Xu , J. , Zhang , Y. X. , Chai , T. Y. , Guan , Z. Q. , Wei , W. , Han , L. and Cong , L. 2008 . In vitro multiplication of heavy metals hyperaccumulator Thlaspi caerulescens . Biol. Plantarum. , 52 : 97 – 100 .
- Xu , L. S. , Zhou , S. B. , Wu , L. H. , Li , N. , Cui , L. Q. , Luo , Y. M. and Christie , P. 2009 . Cd and Zn tolerance and accumulation by Sedum Jinianum in east China . Int. J. Phytoremed. , 11 : 283 – 295 .
- Yamato , M. , Yoshida , S. and Iwase , K. 2008 . Cadmium accumulation in Crassocephalum crepidioides (Benth.) S. Moore (Compositae) in heavy-metal polluted soils and Cd-added conditions in hydroponic and pot cultures . Soil Sci. Plant Nutr. , 54 : 738 – 743 .
- Yanai , J. , Zhao , F. J. , McGrath , S. P. and Kosaki , T. 2006 . Effect of soil characteristics on Cd uptake by the hyperaccumulator Thlaspi caerulescens . Environ. Pollut. , 139 : 167 – 175 .
- Yang , X. E. , Long , X. X. , Ye , H. B. , He , Z. L. , Calvert , D. V. and Stoffella , P. J. 2004 . Cadmium tolerance and hyperaccumulation in a new Zn-hyperaccumulating plant species (Sedum alfredii Hance) . Plant Soil , 259 : 181 – 189 .
- Yu , X. Z. , Cheng , J. M. and Wong , M. H. 2005 . Earthworm–mycorrhiza interaction on Cd uptake and growth of ryegrass . Soil Biol. Biochem. , 37 : 195 – 201 .
- Zaccheo , P. , Crippa , L. and Pasta , V. D. 2006 . Ammonium nutrition as a strategy for cadmium mobilisation in the rhizosphere of sunflower . Plant Soil , 283 : 43 – 56 .
- Zhang , H. , Dang , Z. , Zheng , L. C. and Yi , X. Y. 2009 . Remediation of soil co-contaminated with pyrene and cadmium by growing maize (Zea mays L.) . Int. J. Environ. Sci. Technol. , 6 : 249 – 258 .
- Zhao , F. J. , Lombi , E. and McGrath , S. P. 2003 . Assessing the potential for zinc and cadmium phytoremediation with the hyperaccumulator Thlaspi caerulescens . Plant Soil , 249 : 37 – 43 .
- Zhao , F. J. and McGrath , S. P. 2009 . Biofortification and phytoremediation . Curr. Opin. Plant Biol. , 12 : 373 – 380 .
- Zhao , S. J. , Zhang , Z. C. , Gao , X. , Tohsun , G. and Qiu , B. S. 2009 . Plant regeneration of the mining ecotype Sedum alfredii and cadmium hyperaccumulation in regenerated plants . Plant Cell Tissue Organ Cult. , 99 : 9 – 16 .
- Zhuang , P. , Yang , Q. W. , Wang , H. B. and Shu , W. S. 2007 . Phytoextraction of heavy metals by eight plant species in the field . Water Air Soil Pollut. , 184 : 235 – 242 .
- Ziska , L. H. 1998 . The influence of root zone temperature on photosynthetic acclimation to elevated carbon dioxide concentrations . Ann. Bot. , 81 : 717 – 721 .