Abstract
The COST Action CM1201 “Biomimetic Radical Chemistry” has been active since December 2012 for 4 years, developing research topics organized into four working groups: WG1 – Radical Enzymes, WG2 – Models of DNA damage and consequences, WG3 – Membrane stress, signalling and defenses, and WG4 – Bio-inspired synthetic strategies. International collaborations have been established among the participating 80 research groups with brilliant interdisciplinary achievements. Free radical research with a biomimetic approach has been realized in the COST Action and are summarized in this overview by the four WG leaders.
Introduction
According to the Memorandum of Understanding (MoU) subscribed by the 24 participating countries, as well as by 2 COST Near Neighbor Countries and 2 International Partner Countries, the aims of the COST Action 1201 were:
affirming the centrality of chemistry for the comprehension at a molecular level of processes in biology and technology and the use of biomimetic models as simplified, yet strictly relevant systems, for studying molecular interactions and effects;
fostering an innovative multidisciplinary scenario ideal for Early Stage Researchers (ESRs), in order to address research with an array of methods and know-how from different fields, and more importantly to design biomimetic models for solving complex tasks;
bringing together scientists with complementary, but distinctive interests in chemical and biological subjects. All groups gain not only from the reciprocal expertise, but also in providing a whole scenario for improving the biomimetic modeling;
promoting contributions from different disciplines, such as analytical, radiation, mechanistic and synthetic chemistry, biochemistry, molecular biology, pharmacology, together with computational tools contributing to the understanding of free radical processes at a molecular level in order to reach an integrated vision of chemistry related to other sciences;
addressing important societal needs related to health and technology with favorable impact, and also for clarifying the perception of chemistry’s contribution to the overall progress;
improving a common language among scientists of different background and experience, creating a common environment for sharing methodologies and solving analytical/mechanistic problems, and enhancing the importance of chemical and molecular aspects within the biological and technological scenarios.
Last but not least, the Action’s participants wished to contribute actively to the scientific impact and success of the Action by an increasing number of articles on the common research. The Special Issue of Free Radical Research dedicated to the Action and the papers published herein demonstrate the strong intention to collaborate that animated all participants.
The relevant achievements will be described below in more detail and divided into contributions from each Working Group, thus providing an updated bibliography on the topic of Biomimetic Radical Chemistry.
Working Group 1: radical enzymes
Radical enzymes catalyze many biological reactions, e.g. biosynthetic pathways to antibiotics and cofactors. In addition to this medical context, radical enzymes are essential for the degradation of alkanes and aromatic hydrocarbons derived not only from natural processes, but also as a result of environmental damage from oil spills and other industrially caused pollution [Citation1]. The primary objectives of WG1 were:
To elucidate the catalytic pathways and mechanisms of selected radical enzymes.
To discover new radical enzymes.
To develop model systems and biomimetic chemistry based on the pathways used by radical enzymes.
There were many issues that WG1 sought to resolve through collaborations between members of WG1 and with other Working Groups. Major advances were made in the understanding of the mechanisms of radical enzymes and were aided by computational studies. Novel potent inhibitors of selected enzymes were synthesized. The power of physical methods for determining binding interactions and elucidating reaction pathways was demonstrated.
Radical enzymes catalyze reactions in which species with an odd number of electrons, classically described as “free radicals”, participate [Citation2]. This area has been intensively developed in recent decades and is now a major sub-discipline of enzymology.
Anaerobic degradation of alkanes is in contrast to their long-known O2-dependent oxidation by cytochrome P450 mono-oxygenase [Citation3]. Thus, hexane is oxidized by the proteobacterium strain HxN1 in a tandem process requiring two radical enzymes: the first of which attacks a C-H bond, whilst the second effects a coenzyme B12-dependent molecular rearrangement. A putative HxN1 enzyme uses a cysteinyl radical to abstract stereospecifically the pro-S hydrogen atom from C-2 of hexane. This step may be a concerted reaction in which hydrogen atom abstraction is coupled with addition of the hex-2-yl moiety to fumarate. Computational chemistry employing density functional theory supported this concept in an inter-Working Group collaboration that will be published in due time [L. A. Eriksson (WG2), Buckel, B. T. Golding, and D. M. Smith (Zagreb)]. Current work is focused on the analogous degradation of decane and defining the role of coenzyme B12 in alkane degradation. The expertise of I. Smonou’s group in stereochemically controlled enzymatic reductions of ketones [Citation4] led, in collaboration with Golding’s group, to the synthesis of (2R,9S)-decane-2,9-diol, a precursor of (2R,9S)-[2,9-2H2]decane, required for studies of anaerobic decane oxidation. In related studies, Buckel and Golding (with J. Heider, Marburg) have shown that benzylsuccinate synthase combines toluene and fumarate with inversion of configuration at the methyl group [Citation5].
Sulfur-containing small metabolites are key players in cellular oxidative stress. F. P. Seebeck’s group has studied the mechanism of enzyme-catalyzed oxidative sulfur–carbon bond formation. In the biosynthesis of aromatic thiols, e.g. thiohistidines, there is an unprecedented transfer of a sulfur atom from cysteine or γ-glutamylcysteine (γGC) to the imidazole ring of Nα-trimethylhistidine. The crystal structure of the iron(II)-dependent radical enzyme EgtB that is responsible for the biosynthesis of ergothioneine has been solved [Citation6] and a catalytic mechanism proposed, in which Tyr377 acts as a general acid catalyst [Citation7].
A. K. Croft’s group initiated computations on radical SAM enzymes [Citation8], notably the enzyme QueE, which is involved in biosynthetic pathways for antibiotics, antivirals, and anticancer agents. This work led in 2015 to the award of a Marie–Curie CASCADE fellowship and the Nottingham Research Fellowship to C. Jaeger for the project “Radical Enzymes for Biotechnology – Pioneering with Computational Chemistry”, for which Dr. Croft is host. As a result of this COST Action, inter-WG collaborations have been established with M. Davies (WG3) and H. Zipse (WG4), as well as the groups of M. Boll and M. Seemann.
Most discussions of life’s origin focus on heterolytic chemistry even though many radical processes in biology have been recognized. A computational study taking today’s extant biology [ribonucleotide reductase (RNR) mechanism featuring a cysteine thiyl radical] and extrapolating back, in an attempt to identify prebiotic radical chemistry, led to the proposal that the parent thiyl radical HS• derived from H2S was the prebiotic equivalent of the cysteinyl radical of RNR [Citation9]. Modeling of this process was initiated through collaboration between C. Ferreri (WG3) and B. T. Golding with the aid of an ESR aided by a short-term scientific mission (STSM) grant.
F. Dekker’s group has developed novel small molecule inhibitors for the radical enzyme 15-LOX-1 [Citation10], which plays an important role in the regulation of inflammation. The aim was to inhibit proinflammatory gene transcription, with N. Rohr-Udilova (WG3) performing biological studies on the inhibitors. In addition, the inhibitors will be tested in a cellular model for hepatocellular carcinoma, as 15-LOX-1 is involved in this type of cancer. Interaction with A. Georgakilas (WG2) led to a review [Citation11] on the role of lipoxygenases in both inflammation and cancers. Development of probes for activity-based protein profiling of the radical enzyme lysine demethylase 1 has been pursued by A. Lenoci (University of Rome “La Sapienza”), through funding of a STSM.
Mechanistic investigations of IspH/LytB, a [4Fe-4S]2+ radical enzyme target for the development of new antimicrobials, have been pursued by M. Seemann’s group. In many bacteria (e.g. Mycobacterium tuberculosis), in plant chloroplasts and in the malaria parasite Plasmodium falciparum, the biosynthesis of isoprenoids proceeds via the methylerythritol phosphate (MEP) pathway, an alternative to the well-known mevalonate pathway. The MEP pathway is absent from humans and is therefore a target for the development of new selective antibacterial and antiparasitic drugs. IspH/LytB, the last enzyme of the methylerythritol phosphate pathway, converts (E)-4-hydroxy-3-methylbut-2-enyl diphosphate (HMBPP) into a mixture of isopentenyl diphosphate (IPP) and dimethylallyl diphosphate (DMAPP). In collaboration with V. Schünemann (TU Kaiserslautern), the structure of substrate-free LytB was clarified in solution [Citation12]. Potent LytB inhibitors were designed and prepared in collaboration with D. E. Poulter (University of Utah, Salt Lake City, UT, USA), whereby the OH group of HMBPP was replaced by a thiol (TMBPP, Ki 20 nM) or an amino group (AMBPP, Ki 54 nM) [Citation13].
Mechanisms of action of some catalase inhibitors have been studied by the Gebicki-Gebicka group. Catalases are ferriheme enzymes that catalyze decomposition of hydrogen peroxide to water and molecular oxygen. The catalytic cycle proceeds in two steps, with the formation of radical intermediate, compound I (Cpd I):
{where, Cat = catalase, Por = porphyrin}
Under some conditions, e.g. under low fluxes of H2O2 and in the presence of appropriate substrate, Cpd I is reduced to an inactive Compound II (Cpd II (Por-FeIV = O)). The Gebicki team has found that flavonoids, plant polyphenols, inhibit catalase in vitro [Citation14,Citation15], which is due, at least partially, to conformational changes of the enzyme upon flavonoid binding, and the reduction of Cpd I to inactive Cpd II by flavonoids.
Serum albumins are a significant class of proteins in the circulatory system, which can act as carriers for a broad spectrum of compounds or assemblies such as nanoparticles. Research in G. Ionita’s group was focused on studies of protein/surfactant complexes, monitoring conformational changes induced by temperature, surfactants, and cyclodextrins, using spin labeling methods and EPR spectroscopy to investigate these systems [Citation16]. The collective data may support potential applications in protein purification [Citation17] or in optimizing drug formulations.
Interactions of serotonin with myeloperoxidase were investigated by the group of M. Číž. Serotonin inhibited the chemiluminescence response of neutrophils in human whole blood partially due to the direct scavenging activity of serotonin toward individual reactive oxygen species and partially due to the inhibition of myeloperoxidase activity [Citation18]. Serotonin acts as an alternative substrate for myeloperoxidase and therefore can undergo oxidation via the peroxidase cycle of myeloperoxidase. Recent data suggested that myeloperoxidase activity differs according to the pH value.
Working Group 2: models of DNA damage and consequences
Due to its important role in the maintenance of the genetic information, any modification of the DNA macromolecules may have harmful effects. Hence, the study of DNA damage formation, its efficacy of repair, and subsequent biological consequences are a matter of great interest. Among the genotoxic agents that could damage DNA, ionizing radiations and photosensitization reactions could induce the formation of DNA radicals that can be subsequently converted into chemical modifications of the DNA structure. Several radicals, having very short lifetimes, could be produced giving rise to a plethora of different DNA lesions. Thus, several experimental and theoretical approaches have to be used and combined to better delineate the mechanisms of formation of these DNA lesions. Moreover, to understand the effects of the modifications produced, collaboration with biologists is also essential. This was one of the main objectives of Working Group 2 and is illustrated here by the presentation of several examples of the results obtained by the different partners of this Working Group.
Reaction of singlet oxygen with DNA has been studied in detail during the last two decades [Citation19]. Experimentally it has been shown that 1O2 reacts exclusively with the guanine moiety of DNA [Citation20]. Several guanine decomposition products can be formed (Scheme 1) and interestingly the reactivity is different for the free nucleoside (2′-deoxyguanosine in aqueous solution) compared to what is observed in double-stranded DNA. The postulated mechanism for the formation of the decomposition products involves first the formation of a guanine endoperoxide (3, Scheme 1) through a [4 + 2] Diels-Alder cycloaddition of 1O2 onto guanine (1) [Citation20]. Such an intermediate has been observed experimentally at low temperature [Citation21]. Its decomposition generates spironucleosides (4), 4-hydroxy-8-oxo-2′-deoxyguanosine (5) and 8-oxodGuo (6) as final stable products (Scheme 1). At the nucleoside level, spironucleosides are the major products, whereas in double-stranded (ds)DNA, 8-oxodGuo is predominantly generated.
Scheme 1. Proposed mechanisms for the reaction of singlet molecular oxygen (7O8) with the guanine moiety of DNA.
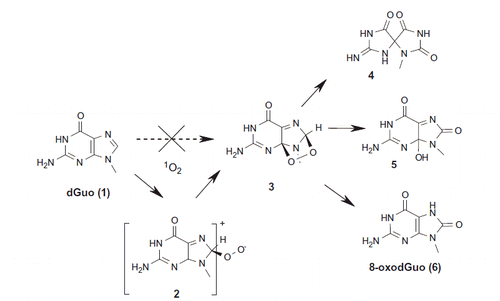
A couple of experimental results were difficult to explain. First, if 1O2 reacts with dGuo through a Diels-Alder reaction with the imidazole moiety of that purine base, why does such a reaction not take place with adenine, the other purine base having a similar imidazole ring? Second, why is the reactivity of 1O2 with guanine different in DNA compared to what is observed for the free nucleoside in aqueous solution? Theoretical approaches have been used to decipher the reaction mechanisms of singlet oxygen with DNA and to compare its reactivity with guanine and adenine [Citation22,Citation23]. The results show that if indeed the endoperoxide is generated by reaction of singlet oxygen with guanine; its formation does not proceed through a Diels-Alder reaction, since 1O2 first adds to the C8 position of guanine to produce a zwitterionic intermediate (2). A higher energy barrier has been calculated for reaction with adenine, giving for the first time an explanation of the lack of reactivity of 1O2 toward adenine. Moreover, the attack of singlet oxygen onto 8-oxodGuo has been found to have only a very low barrier, rationalizing the experimentally observed higher reactivity than guanine itself [Citation25]. The results obtained highlight the importance of theoretical studies [Citation24,Citation25] to delineate the mechanisms of formation of DNA lesions that have been identified experimentally.
Theoretical approaches have been also used to model the interaction between DNA and external molecules potentially acting as photosensitizers [Citation26–38]. This has allowed the recognition of different competitive modes, by means of classical all-atom molecular dynamics, and the subsequent elucidation of the different photochemical pathways [Citation26,Citation27]. Modeling of electronic circular dichroism also allows for a straightforward relation with experience, also in the case of noncanonical DNA structures such as G-quadruplexes [Citation28,Citation32]. In particular, we have established the presence of a double-inserted interaction mode for benzophenone, and relying on QM/MM methodologies, we have also evidenced the mechanisms of triplet transfer toward DNA thymine [Citation35]. Finally the use of nonadiabatic dynamics has allowed unravelling of the time scale and the electronic mechanisms leading to the triplet population in benzophenone despite the relative weak spin-orbit coupling, i.e. the first step of photosensitization [Citation30]. We have also analyzed the possibility of other photochemical reactions such as hydrogen abstraction [Citation31], evidencing strong environmental effects and a global self-protection by DNA. In the same spirit, we have analyzed electron transfer, in the case of Nile red and Nile blue sensitizers, showing once again a marked effect of the DNA environment [Citation33]. Also the photophysical mechanisms leading to the environmental controlled production of singlet oxygen by the alkaloid palmatine only upon DNA interaction have been rationalized as mainly due to the differential stabilization of charge-transfer excited states [Citation26]. Finally sensitization by artificial nucleobases mimicking DNA lesions has also been assessed.
The research of WG2 has also been focussed on complex DNA lesions that may represent a challenge for the DNA repair machinery. Among them, 5′,8-cyclo-2-deoxyadenosine (cdA) and 5′,8-cyclo-2-deoxyguanosine (cdG) are tandem lesions produced by hydroxyl radicals (HO•) of endogenous or exogenous origin that insult DNA by abstracting a hydrogen from the C5 of a purine nucleoside moiety [Citation39]. Both cdA and cdG lesions having a C5′–C8 bond exist in two diastereomeric forms, 5′S and 5′R (Scheme 2).
There were many issues related to cdA and cdG lesions that WG2 addressed through collaborations between its members and associated scientists from USA. Major advances were made in the synthesis of two diastereomers of cdG and their incorporation into oligonucleotides [Citation40]. Lipophilic derivatives of cdG have been used to study the association constant with cytidine [Citation41] and formation of G-quadruplex in chloroform [Citation42]. Spectroscopic data indicated that the Watson-Crick complex of cytidine with cdG is weaker than with guanosine, the difference being one order of magnitude between the two association constants [Citation41]. A “fully-anti” lipophilic G-quadruplex, a dodecameric complex composed of three stacked G-quartets of 5′S-cdG in the presence of strontium picrate, was observed for the first time [Citation42].
A cost-effective and efficient protocol for the quantification of the four purine 5′,8-cyclo-2′-deoxynucleosides together with the two 8-oxo derivatives (8-oxo-dA and 8-oxo-dG) in oxidized DNA was obtained [Citation43] and applied to radiation-induced formation of purine lesions [Citation44–46]. In single- and double-stranded DNA, the overall lesion formation follows the order: 8-oxo-dG ≫8-oxo-dA >5′R-cdG >5′R-cdA >5′S-cdA >5′S-cdG [Citation44]. The rate constants for a unimolecular pathway were estimated to be ca. 5 × 104 s−1 for both 2′-deoxyadenosin-5′-yl and 2′-deoxyguanosin-5′-yl radicals in ss-DNA, and about halved (2–3 ×104 s−1) in ds-DNA [Citation44,Citation45]. The same purine lesions were also quantified in order to individuate the influence given by different DNA helical topology and folding on the purine exposure to the damage [Citation46]. This was investigated in tertiary dsDNA helical forms of supercoiled (SC), open circular (OC), and linear (L) conformations, along with single-stranded folded and non-folded sequences of guanine-rich DNA in selected G-quadruplex structures. Purine oxidation was indeed different following the order: L > OC ≫ SC, indicating greater damage toward the extended B-DNA topology. In G-quadruplex sequences, the unfolded states were more resistant compared to G-tetrad folded topologies.
Major advances were also made in understanding the biology of these lesions by incorporating them in DNA sequences. Both cdG and cdA lesions, in their 5′S and 5′R diastereomeric forms, are repaired at low efficiency by the human nucleotide excision repair (NER) pathway, there being ca. 2 times greater efficacy for 5′R over 5′S diastereomer repair, owing to greater DNA backbone distortions encountered in 5′R diastereomeric lesion-containing sequences [Citation47,Citation48]. Given the known correlation among oxidative DNA damage and its repair in trinucleotide repeats (TNR), it was investigated whether TNR instability can be induced by the cdA lesions during replication and repeat [Citation49]. Evidence that a cdA can effect TNR deletion by inducing the formation of various sizes of loop structures with the lesion in the template strand of a TNR repeat tract were obtained. DNA pol β efficiently bypasses 5’R-cdA, but inefficiently bypasses 5′S-cdA during DNA replication and base excision repair (BER) [Citation50]. This result highlights that the diastereomeric nature of purine cyclonucleoside lesions can play a crucial role in DNA repair mechanisms.
Even though much work has been done during the last three decades to determine the nature of the modifications produced in DNA exposed to ionizing radiations, we could not totally exclude that still unknown lesions are produced in cells. In particular, much attention has been focused on complex DNA lesions produced by a single radical [Citation51], as described above for cyclonucleosides. These lesions could be produced by reaction of the initially generated radicals with surrounding molecules and in particular with other DNA bases producing intra-strand crosslinks and also tandem lesions (for a review see [Citation52]). In cellular DNA, the situation is even more complicated since DNA is covered by several proteins, in particular histones, and also other small molecules such as polyamines that play an important role in the regulation of several biological functions. Previous works have shown that the guanine radical cation (G•+), that is the main one-electron oxidation product of DNA due to an efficient charge transfer reaction in dsDNA, reacts with water to produce 8-oxodGuo. Moreover, addition of other nucleophilic molecules [Citation53], including for example lysine to produce guanine-lysine crosslinks [Citation54], has also been reported. In addition, it has been also shown that the presence of tyrosine could chemically repair G•+ to restore guanine [Citation55,Citation56], by an electron transfer reaction from tyrosine to G°+. This suggests that in cells, the fate of the initially produced guanine radical cation will depend on its environment. Since polyamines, including spermine, spermidine, and putrescine are found at very high concentration in the nucleus of eukaryotic cells (mM range) the possibility that these nucleophilic molecules add to G•+ has been studied. It has been shown in vitro that such addition is very efficient [Citation57], and that the presence of these polyamines (at concentrations below 1 mM) completely inhibits the formation of 8-oxodGuo that is compensated by the formation of polyamine-dGuo adducts. The reactivity of the guanine radical cation has been used to determine that UV-induced formation of oxidative DNA lesions involves a one-electron oxidation reaction and not singlet oxygen as initially reported [Citation58]. Additional work has now to be done to determine the importance of these lesions at the cellular level. This requires the development of a highly sensitive and specific method that could quantify levels of modifications lower than one lesion per million nucleosides, using a few microgram of DNA. Nowadays, HPLC coupled through electrospray ionization to tandem mass spectrometry represents the most powerful method that has already been applied to monitor several lesions in cellular DNA exposed to oxidative stress [Citation59].
However such methods do not allow localization of the lesion within the DNA sequence, and following one-electron oxidation reactions, the DNA sequences play a predominant role. Indeed, the redox properties of DNA are of outstanding importance for understanding the site distribution of DNA oxidative damage and for clarifying mechanistic aspects of long-range hole transfer. In that context, the possible establishment of charge delocalized domains is of particular significance, in as much as delocalized domains are expected to exhibit lower oxidation potentials, changing hole site energies and speeding up hole migration along the strand. In a collaborative research differential pulse voltammetry (DPV) has been carried out on several guanine-rich oligonucleotides, containing up to six consecutive guanines. The progressive lowering of the first oxidation potential as the number of adjacent guanines increases unambiguous points toward the establishment of delocalized hole domains [Citation60]. Simulations of hole transfer dynamics in double-strand G(T)nG oligonucleotides, performed by using a set of parameters inferred from electrochemical measurements, have clearly shown the effects of delocalized domains on hole transfer rates: for small n, hole transfer rates exponentially depend on the donor–acceptor distance, whereas for n > 3, the rates become almost independent of distance. That behavior has been assigned to the formation of hole delocalized domains in the complementary strand, which by increasing n become nearly degenerate with the donor state [Citation61].
DPV measurements have also been used to investigate the redox properties of 5′,8-cyclo-2′-deoxyguanosine (cdG), the DNA tandem lesion referred to above. Electrochemical measurements have shown that the interaction energies of the damaged nucleoside with cytosine differ from that of undamaged base. Indeed, the equilibrium association constant, determined by NMR titrations, is found to be ca. 103 M−1, about one order of magnitude less than that for guanosine [Citation41].
In addition to complex lesions produced by a single radical, ionizing radiations produce lesions in cluster, also called multiple damage sites (MDS), constituted of several modifications (including oxidized bases, strand breaks, abasic sites, etc…) within one or two helix turns. When LET (Linear Energy Transfer) increases the complexity (number of individual lesions in one multiple damage site) increases, since more ionization events take place in a defined volume. In a close collaboration with Iliakis’ (Germany), Ravanat’s (France), and Martin’s (Australia) groups, the DNA Damage Laboratory of A. Georgakilas (NTUA, Greece) has proposed an improvement of methodologies used for the detection of oxidative clustered DNA lesions in vitro and in vivo with special emphasis on the in situ detection including bystander effects [Citation62–65]. In addition, bioinformatics has been used to better understand the DNA damage response mechanisms relating to ionizing radiation-induced DNA damage and their association with inflammatory and immune response pathways [Citation66–71]. Collaboration between the groups of Monari and Dumont (France), and the Georgakilas group, through the application of Molecular Dynamics (MD) simulations, gave mechanistic insights on the processing of clustered DNA lesions especially for cases where it is very difficult to acquire analytical experimental data [Citation72,Citation73]. By using classical molecular dynamics, we have analyzed the position and sequence-dependent structural modification induced on B-DNA by the presence of a bistranded clustered abasic lesions. In particular, we have seen that the deformation may lead to the loss of the abasic lesions signature, hence preventing recognition by a repair enzyme such as bacterial Nfo. On the contrary, human repair enzymes such as APE1 exert much stronger mechanical constraints and are characterized by higher repair rates. There are a few exceptions that have been examined in these papers. In addition, through the use of Monte Carlo (MD) simulations, a special biophysical model was developed for the simulation of radiation-induced systemic effects and the interpretation of various nontargeted effects [Citation67]. Last but not least, in a series of publications, Georgakilas’ group within its participation in the network of the Halifax Project contributed toward the designing of a broad-spectrum integrative approach for cancer prevention and treatment as reviewed in [Citation73,Citation74]. Overall, such a work within this COST Action has been the development of a solid mechanistic basis for the induction and propagation of oxidative clustered DNA damage in tissues and organs as the result of ionizing radiation exposure or tumor development, where oxidative stress is involved. Special emphasis was given in the delineation of cellular and organism mechanisms implicated in the confrontation of complex DNA damage and the result of deficient repair [Citation75].
Since DNA is a target of several medical approaches aiming at killing undesired cells, for example for cancer therapy (using chemo- or radio-therapeutic approaches, as well as photodynamic therapy), increasing our basic knowledge in this field would certainly allow to improve the efficacy of such treatments.
One of approaches that help to overcome the hypoxia-related radioresistance of tumor cells is to substitute their DNA with sensitizing nucleosides. This is why the reactivity of halogenated 2′-deoxynucleosides (brominated (BrdX) or iodinated (IdX)) toward organic radicals and solvated electrons (both types of species are produced during the radiolysis of cellular environment) was investigated in the current project.
Using HPLC and LC-MS techniques, it was demonstrated that beside the substitution of bromine atom by 2-hydroxypropyl radical (OHisop•), electron transfer from OHisop• to BrdX takes place only for 5-bromo-2′-deoxyuridine (BrdU) and 5-bromo-2′-deoxycytydine (BrdC) due to larger electron affinity (EA) of pyrimidines than purines [Citation76]. The larger EA of brominated pyrimidines also accounts for more efficient damage induced by hydrated electrons in the trimeric oligonucleotides, TBrdXT, labeled with BrdU or BrdC [Citation77]. Moreover, the extent of damage, especially the formation of cytotoxic single-strand breaks induced by hydrated electrons is doubled in the trimeric oligonucleotides labeled with IdU or IdC compared to their counterparts labeled with BrdU or BrdC [Citation78]. Our studies showed unequivocally that hydrated electrons are a negligible factor in damage to native DNA even for a ionizing radiation dose exceeded many times the doses used in radiotherapy [Citation77]. Therefore, some kind of radiosensitization seems to be an indispensable component of any efficient radiotherapy.
In WG2, the Kellet laboratory focused on the development of nucleic acid-interacting inorganic materials for biochemical and therapeutic application. Since the discovery of the first chemical nuclease, [Cu(phen)2]+ (where phen =1,10-phenanthroline), this agent has served as a template for the rational design of new DNA oxidants and has been widely applied as a footprinting reagent. Recently, we reported [Cu(phen)2]+ as a novel agent for protein engineering when applied under Fenton-type conditions against a recombinant antibody fragment specific for prostate-specific antigen (PSA) for the generation of recombinant mutagenesis libraries. This study was highlighted as a front cover article in Chemical Communications and may now open up new applications for inorganic DNA oxidants [Citation79]. By structurally modifying this chemotype, bis-chelate Cu(II) phenanthroline-phenazine complexes [Cu(DPQ)(phen)]2+, [Cu(DPPZ)(phen)]2+ and [Cu(DPPN)(phen)]2+ were recently reported to improve DNA intercalation and sequence selectivity [Citation80]. This complex series displayed high-affinity DNA binding at both the major and minor grooves, where the most active derivatives (DPQ and DPPZ) demonstrated the highest known binding constants for Cu(II) complexes at the time of publication. DNA oxidation was further quantified through an “on-chip” microfluidic protocol [Citation80]. Further mechanistic investigation of this class, reported in collaboration with the Chatgilialoglu group [Citation81], revealed the DNA cleavage mechanism was linked to metal-oxo or free hydroxyl (•OH) radical production that produced the oxidative lesion 8-oxo-dG. Metal-catalyzed oxidative DNA damage of oligonucleotide templates were further found to inhibit the polymerase chain reaction (PCR); this reaction was particularly impeded within A·T transcripts [Citation81]. Since copper(II) phenanthroline complexes also possess therapeutic potential, the anticancer activity of a square planar dicarboxylate complex [Cu(o-phthalate)(phen)] was recently reported [Citation82]. Submitted to the National Cancer Institute (NCI) 60 cancer cell line screen, the complex exhibited broad-spectrum anticancer activity by exerting mitochondrial and nuclear DNA damage via superoxide-mediated ROS production [Citation82]. The Kellett group has also pursued platinum(II) drug research in collaboration with the group of N. P. Farrell: fully trans-symmetric tri-platinum(II) (Triplatin) complexes with varying aliphatic diam(m)ine linkers were reported [Citation83]. The architecture of this chemotype facilitates a noncovalent surface interaction called the “phosphate clamp” that results in two binding motifs: backbone tracking and groove spanning. This work revealed the two limiting modes of phosphate clamping are sequence selective and could be distinguished in solution through conformational changes. Triplatin-DNA binding also prevented endonuclease activity by blocking the action of site-selective type II restriction enzymes [Citation83].
The above reported results obtained by participants of the WG2 highlight the importance of networks bringing together scientists having different and complementary expertise and working in a similar field of interest, such as radiation-induced damage to DNA. Exchanges of ideas or experimental/theoretical competences during presentations and discussions through frequent meetings organized by such a network promote collaborations between physicists, chemists, and biologists who usually do not have the opportunity to discuss together. Multidisciplinary approaches are required to better understand the effects of radiation on DNA, keeping also in mind that cells are constituted not only of nucleic acids but also of lipids and proteins. In addition, such a network facilitates the involvement of young scientists who represent the future of the European research community.
Working Group 3: membrane stress, signaling, and defenses
Membrane organization as a phospholipid double layer and its chemical and biochemical reactivity attracted more research interest starting from the 1990s, as shown by a Web of Science search [Citation84]. However, the importance of cell membrane formation given by spontaneous phospholipid assembly and the connection between phospholipid structures and functions were already well understood in the 1970s, as explained by the fluid mosaic model of Singer and Nicolson [Citation85]. Only many years later a larger view of the phospholipid molecules, reactivity, and consequences started to be addressed by appropriate research, in particular evidencing free radical reactivity with lipids as a crucial process to occur under stress conditions, accompanying other biochemical and chemical stress processes regarding all cell components. The activity of Working Group 3 was directed toward an interdisciplinary approach to address the research described below.
Lipid peroxidation, membrane signaling, and antioxidant mechanism
Lipid peroxidation mainly involves polyunsaturated fatty acids (PUFA) and leads to their transformation and degradation [Citation86]. One of the interesting products is the family of α,β-unsaturated aldehydes, the 4-hydroxyalkenals [e.g. 4-hydroxynonenal (4-HNE)], which derive from the omega-6 reactivity, as well as two other members of this family, 4-hydroxy-2E-hexenal (4-HHE) and 4-hydroxy-2E,6Z-dodecadienal (4-HDDE) [Citation87,Citation88]. They are chemically reactive, forming covalent adducts with amino or thiol moieties in proteins, phospholipids, and nucleic acids, and the toxicity of the adducts can determine different pathological and cytotoxic processes. However, there is also an important signaling role for these aldehydes that was discovered studying beta pancreatic cells after glucose stimulation. In fact, 4-HNE signaling stimulates the cell response for insulin production, after liberation of the precursor omega-6 fatty acid from cell membranes and transformation by peroxidation [Citation89–91]. Another interesting molecular mechanism was discussed in the Working Group, determining that by the interaction of these aldehydes with amino-containing membrane phospholipids (phosphatidyl ethanolamine, PE) lipid adducts are formed, with consequences for the transmembrane translocation process of proton and potassium ions [Citation92].
The research of Working Group 3 has clearly evidenced membrane participation in stress processes, which include signaling and other biological consequences. Indeed, a large number of signaling cascades start from the detachment of fatty acids from membrane phospholipids and this phenomenon causes a profound membrane fatty acid remodeling for the phospholipid replacement, known as Land’s cycle [Citation93]. This natural remodeling cycle accompanying each cascade is an opportunity for membrane shaping and controlled phospholipid replacement following the availability of fatty acids in the organism. The WG3 expertise of several groups (Rome Catholic University, Hebrew University of Jerusalem, Consiglio Nazionale delle Ricerche, University of Patras) provided a multidisciplinary environment that led to an understanding of remodeling (evaluated by fatty acid-based membrane lipidomics and analysis of fatty acid metabolites such as 4-HNE) and its consequences on membrane physical parameters (evaluated with biophysical methods) in two case studies: pancreatic beta-cells exposed to different glucose and palmitic acid concentrations, and red blood cells of genetic mouse models defective in lipoproteins [Citation94]. The importance of the membrane molecular variations associated with signaling cascades will be surely deepened in further research focused on inflammatory and metabolic processes. This result will trigger also new perspectives in the fields of nutritional and physiochemical conditions to control cell membrane compositions, which can contribute to the control of degenerative processes that accompany stress and related responses.
The role of lipid peroxidation as a free radical-mediated mechanism has been better and better understood, nowadays seen not only as damage caused to cells and their membranes, precisely by the reaction of unsaturated fatty acid moieties, but also as a trigger of cellular stress response. This implies also the activation of enzymatic defenses and the role of antioxidants, in a complex balance involved in pathological conditions such as inflammation, as well as in the progression of the aging. The Working Group 3 of the Action was very active on this aspect. In particular, the antioxidant mechanism was thoroughly studied by Valgimigli’s group as related to anthocyanins [Citation95] and to the redox balance connected with longevity [Citation96]. In the field of antioxidants, some studies addressed analytical detection of oxidative stress in humans. New methods have been reported such as a noninvasive EPR methodology [Citation97], as well as antioxidant evaluation for in vitro activity [Citation98].
The evaluation of the protection from oxidative stress given by a number of vitamins and cofactors in animal models and humans was also addressed in the WG3 activities. A collaborative research between Italy, Greece, and France was related to a mitochondrial-targeted antioxidant used in rats fed an obesogenic diet [Citation99], establishing positive protective effects on the polyunsaturated moieties of cardiolipin, the typical lipid of mitochondria. The model of diabetic rats was used to investigate the effect of SOD-mimics and L-arginine on skin injuries, based on the correction of the ratio between superoxide radical anion and nitric oxide, proving that restitution of redox balance can be an approach for the resolution of such dermatological problems [Citation100].
Children affected by autism spectrum disorders (ASD) were considered for their levels of thiamine and related phosphorylated metabolites in plasma and urine in a collaborative study between Warwick and Bologna Universities. A significant decrease (24%) of thiamine pyrophosphate in the patients was evidenced compared to controls [Citation101].
Determination of antioxidant status in diseases was addressed in a collaborative paper between Akdeniz University of Antalya, Turkey and University of Modena and Reggio Emilia, Italy. They studied the dynamic thiol/disulfide homeostasis in prostate cancer patients. Indeed, patients showed less thiol and total antioxidant status (TAS) than controls and there was a negative correlation between the prostate specific antigen (PSA) and thiol levels [Citation102].
Lipid isomerization
The cis-trans isomerization process discovered in 1999 occurs by free radicals adding reversibly to lipid double bonds, thus transforming the naturally occurring cis geometry of unsaturated lipids into the thermodynamically more stable trans configuration, without shifting the double-bond position [Citation103]. In this process, the bent molecular shape of the cis isomer is converted into the almost straight structure of the trans isomer. This molecular conversion has been an important focus of Working Group 3 and review articles summarized the state-of-art in the field [Citation104]. Collaborative research in the COST Action was directed toward the details of isomerization processes in different biomimetic models, such as liposomes and micelles.
Micelles formed by linoleic acid were studied for their reactivity under irradiation, in aerobic and anaerobic conditions, in the presence of aromatic amines and thiols [Citation105]. Depending from the reaction conditions, isomerization and peroxidation processes could be examined and compared, confirming that both processes contribute to the fatty acid changes under free radical conditions, as previously detected only with thiol [Citation106]. In the presence of amines, the cooperative production of thiyl radicals was evidenced, that contributes to increase the cis-trans double-bond conversion. The isomerization vs. peroxidation processes were also evaluated in this system in the presence of antioxidants such as resveratrol, ascorbic acid, and alpha-tocopherol, finding that trapping of the initiating radicals, generated by ionizing radiation in the water phase, and co-localization of the antioxidant at the hydrophobic core of the micelle are necessary for an effective inhibition of lipid degradation [Citation107]. In liposomes made of phospholipids with unsaturated fatty acid residues, the isomerization process was also evaluated in detail, comparing also different system of liposome formation (injection vs. extrusion methodologies), as well as the inhibition effect of the most common antioxidants [Citation108].
Fatty acid-based membrane lipidomics applied to erythrocyte membrane mapping
In the activities of the COST Action CM1201, the training of early stage researchers (ESR) was an important objective achieved by learning protocols to examine the membrane effects at the level of fatty acid residues in phospholipids. This included a thorough training in protocols for the isolation and analytical determination of fatty acids.
The WG3 activities, involving the work of 3 ESRs, led to the discovery of a new fatty acid biosynthetic transformation that occurs in human metabolism. Fatty acid biosynthesis is known to start from palmitic acid (hexadecanoic acid, C16:0), obtained by de novo synthesis, that is subsequently converted to palmitoleic acid (9-cis-hexadecenoic acid, C16:1) by the intervention of stearoyl CoA-desaturase enzyme. An increase of this metabolism has been correlated to diseases such as obesity and cancer. In the research developed in this COST Action, a new conversion of palmitic acid was discovered that is carried out by the delta-6 desaturase, normally operating with PUFA. In the case that delta-6 desaturase works on palmitic acid, it leads to the formation of a monounsaturated fatty acid, that is positional isomer of palmitoleic acid, namely sapienic acid (6-cis-hexadecenoic acid, C16:1) [Citation109]. The presence of such isomer was not known previously in human blood, and this was a discovery carried out in the frame of the COST Action. After setting-up a protocol for the effective separation and recognition of fatty acids in plasma, red blood cell and lipoprotein lipids, the presence of positional and geometrical (cis and trans isomers) isomers of C16:1 was achieved applying specific conditions for the full resolution by gas chromatography of the hexadecenoic fatty acid family. This research arose an important awareness for lipidomic studies concerning the identification of positional and geometrical isomers possessing the same molecular weight, that are nowadays proposed as biomarkers in several diseases. Subtle differences of mass fragmentation percentages might be difficult to evidence or interpret, especially in biological samples where lipids are mixed and in low quantities. Therefore, a combined approach was studied to corroborate mass spectrometry analysis with other structure determination methods to arrive to an unambiguous determination. The research in this COST Action research achieved a high resolution protocol for positional and geometrical isomers. An important application was the analysis of fatty acid components of red blood cell membrane phospholipids and plasma cholesteryl esters in healthy human subjects and morbidly obese patients, revealing that sapienic acid is an interesting biomarker of metabolism diversion in obese subjects versus controls. These findings are important for deepening biological studies of lipid enzymes, such as delta-9 and delta-6 desaturases as well as LCAT (lecithin cholesterol acyl transferase), that are certainly key steps of metabolism differentiation in health and disease [Citation110].
In a collaborative research between Italy and Argentina, fostered by the COST Action environment, the erythrocyte membrane lipidome in infertile men was considered in a representative group of 11 subjects. Significant differences with healthy population were evidenced, especially highlighting an unbalanced membrane status, with loss of important components such as omega-3 fatty acids. After a supplementation of these fatty acids effected for 3 months, a second analysis on plasma and sperm membrane lipids was carried out thus evidencing that changes of membrane lipidome occurred mostly in the erythrocytes, with increased levels of the supplemented fatty acids [Citation111]. This study is also important for nutraceutical applications, suggesting to approach by membrane lipidomics the bioavailability of supplemented or dietary fatty acids for sperm cells.
Another issue addressed by the WG3 research in lipidomics was the importance of building up integrated panels with other molecular methodologies, such as for example, the measurement of DNA damage, foreseeing the use for diagnostics taking into account the full monitoring of physio-pathological status of cells related to diseases. Using auto-modified poly(ADP-ribose)polymerase (PAR-PARP), a well-known early DNA damage sensor, and erythrocyte membrane fatty acid composition of 95 subjects with different health conditions, the statistical correlation between disease presence and alteration of these molecular indicators was evaluated. Statistical significant correlations were found in some conditions between these two indicators, generally in agreement with the knowledge that PARP activation is connected with upregulation of proinflammatory gene expression for which PUFA are the signaling molecules. In particular, positive correlation was found between PAR-PARP levels and DHA (omega-3 fatty acid) in thyroid diseases; in Hashimoto’s disease, increased EPA (omega-3 fatty acid) correlated positively with PAR-PARP levels; in patients affected by allergy, PAR-PARP corresponded to an increase of DGLA (omega-6 fatty acid) and DHA levels; in patients affected by HCV (Hepatitis C virus), PAR-PARP levels were inversely correlated to EPA and DHA values [Citation112].
Free radical biomimetic and biological models
In the frame of the interdisciplinarity of free radical research in biosciences, that is one of the main objectives of the COST Action CM1201, a combination between biomimetic and biological models was successfully obtained by a collaborative work on the mechanism of the bleomycin-induced phospholipid transformation [Citation113,Citation114]. Bleomycin is an antitumoral drug, with a well-known mechanism of intercalation in the DNA sequence and generation of hydroxyl radicals that cause DNA strand break. In a collaboration in the WG3 between the Ferreri (Italy) and Ozben (Turkey) groups, it was found for the first time that bleomycin is able to damage membrane lipids, both via inducing peroxidation and, in the presence of thiols, isomerization processes. The mechanism and influencing factors were tested in biomimetic models of liposomes, containing mono- and poly-unsaturated fatty acids. In parallel, testicular cancer germ cells were cultured with bleomycin, showing that lipid peroxidation and isomerization do occur as seen in the models. Also the signaling related to such transformation has been examined in some detail.
Another biomimetic model that has been studied in the collaborative environment of WG3 was related to the generation of radicals on peptide sequences, in particular to understand the pathways of transformation involving sulfur-containing moieties. The role of methionine (Met) as amino acid and in peptides was thoroughly studied by the group of Chatgilialoglu, and in this COST action the Gly-Met-Gly sequence and its N-acetyl derivative, which simulate the “internal” disposition of Met, were used for an interdisciplinary approach, including kinetic, mechanistic, computational, and product studies [Citation115]. Significant differences of the radical generation and fate were detected compared to previous studies carried out with free Met. Mechanistic pathways and a full scenario of the reactivity were obtained by pulse radiolysis detection of transient species, radiolytical, and electrochemical transformations of the tripeptide and a detailed product analysis.
The 4 years of collaborative research of Working Group 3 evidenced the crucial role of membrane lipids and membrane processes in the free radical transformations of biological importance, and this increased knowledge will certainly be relevant for a large scientific audience. These achievements acquired an important applicative meaning, due to the participation of companies in this Working Group, which were also as co-author of some papers [Citation90,Citation110,Citation112]. The presence of companies certainly contributed to the discussion of results in terms of scientific innovations that have to be brought to the market for amelioration of the products and improving benefits to consumers.
The final goal of Working Group 3 dedicated to the membrane field was to create a bridge between chemical models and biological systems for the study of the influence of membrane organization and structures. This included the effects of chemical and biochemical transformations, taking into account that the main signaling activities depart from membranes with cascades involved in metabolic processes such as stress, inflammation, and aging, as well as the protection given by specific compounds with antioxidant/anti-isomerizing properties. The two main lipid transformations, peroxidation and isomerization, and the lipid remodeling were focused upon and the Working Group discovered several new aspects of the fatty acid influence on the favorable recovery of the whole membrane functioning and homeostatic balance. Actually bringing the aspect of membrane lipidomics into molecular sciences was thoroughly discussed among the Action participants, aiming at having a complete scenario of the cellular activities, taking account of the undoubted role of cell membranes for replication and life.
Discussion of the above reported results with the other Working Groups brought about very productive interactions and new collaborations, thus creating innovative research networks that can apply to the Horizon2020 calls.
Working Group 4: bio-inspired synthetic strategies
Working Group 4 was concerned with the development of bioinspired synthetic organic strategies having free radicals as central intermediates. Nature uses free radicals to accomplish notoriously difficult biosynthetic transformations to produce the molecules it needs for signaling, metabolism, and host defense. To accomplish that, Nature applies, on one hand, enzymatic catalysis to bring about these transformations very specifically. On the other hand, spontaneous free radical-based events, such as autoxidative or single-electron transfer processes occur frequently. Both of these free radical-based pathways have implications for the design of synthetic strategies. In contrast to Nature, which applies free radicals in a very specific environment, organic chemistry is ideally suited to probe the generality of free radical chemistry. This means that a much larger chemical space can be covered by synthetic endeavors. Small-molecule catalysts can be discovered and varied in wide margins, may they be organic or metal-based. Light can be used to trigger radical generation, biomolecules may be selectively modified by free radical C-H functionalization, and radical reactivity can be modulated by the electronic and steric properties of substituents. This leads to new insights into the physical parameters of free radical reactions. Here, collaboration with computational chemists is very important for providing rationales for the observed reactivity patterns. This reasoning formed the basis for the collaboration among the Working Groups in the COST action and has been amply demonstrated by the results obtained by the members of the Working Group as illustrated below.
The synergistic combination of expertise was accomplished in an inter-working group mode, as documented by the collaborative work of the Ferreri and Lykakis groups, who studied the biomimetic thiyl radical chemistry [Citation105,Citation106] and the protection of phospholipids from thiyl radicals by antioxidants [Citation107,Citation108], as explained previously in the Working Group 3 activities. Another important collaboration concerned the investigation of iron-based 1-aminocyclopropane-1-carboxylic acid oxidase mimics, whose kinetic and mechanistic features were experimentally and computationally determined [Citation116].
A major effort in WG4 was directed toward the discovery of catalytic radical reactions. The Ollivier, Goddard, and Fensterbank group reported photoredox-catalytic Barton-McCombie reactions as an alternative to the traditional method mediated by toxic tributyltin hydride [Citation117]. Inspiring dual photoredox- and nickel-catalyzed cross-coupling reactions of benign alkylsilicates provided interesting products [Citation118]. The Lykakis group investigated heterogeneous catalysts and especially the photocatalytic activity of mesoporous ternary polyoxometalate-Ag2S-CdS semiconductors [Citation119]. The Jahn group provided the first examples of oxidative electron transfer-catalyzed polar-radical crossover reactions, in which the necessary stoichiometric sacrificial oxidant is at the same time an oxygenation reagent and allows more atom-economic reactions [Citation120]. The Speier/Kaizer group reported bioinspired organocatalytic oxidations of thiols [Citation121] as well as 1,3,2-oxazaphosphole catalysts as flavin mimics [Citation122].
The power of radical chemistry to contribute to the development of green and sustainable chemistry was demonstrated. The Speier/Kaizer group reported the use of greenhouse gas CO2 as an oxidant and a C1 building block in organic chemistry [Citation123]. A green method for the oxidation of alcohols using heterogeneous photocatalysis was developed by the Lykakis group [Citation124].
The potential of changing the properties of biomolecules by radical chemistry is another focus in the Working Group as exemplified by an approach to selective oxidative intramolecular modifications of cyclodextrins [Citation125].
Last, but not least, organic synthesis with the help of free radical was significantly developed in the action. Such approaches open up new avenues for medicinal chemistry to increase the potential of three-dimensional biologically active entities. The Renaud group described a radical cascade reaction for the preparation of pyrrolidine derivatives, using thiyl radical addition to a terminal alkyne function as the first step, which was followed by a 1,5-hydrogen transfer, translocating the radical center for the subsequent cyclization to afford the heterocyclic ring [Citation126]. The Herrera group demonstrated that normally hard to functionalize methyl groups can be easily activated for cyclizations to pyrrolidines by the iodine/iodobenzene diacetate reagent system [Citation127]. Antiviral cyclopentane derivatives became easily accessible by new complex tandem reactions consisting of polar organometallic additions coupled with radical cyclizations and oxygenations [Citation128,Citation129] and a new approach toward the total synthesis of bridged diketopiperazine alkaloids using the persistent radical effect emerged [Citation130]. Alternatively, the central diazabicyclo[2.2.2]octane core of several alkaloids can be approached by reaction sequences proceeding through single electron transfer (SET) oxidation steps, thus switching among multiple intermediate types of different oxidation state in one pot [Citation131].
The importance of free radical processes in stereoselective synthesis for the transformation of enantiomerically pure substrates without racemization was also addressed in the WG4 research, as beautifully reviewed by Renaud’s group [Citation132].
These examples document the central role of organic synthesis based on free radicals to develop more efficient processes, to enable new and more benign approaches to organic compounds, new methodology for medicinal and material chemistry, as well as to provide new tools and standards for biology.
Conclusions
The summary of the activities carried out by the COST Action Working Groups demonstrates the successful implementation of the proposal to bring free radical research at the cross-road of life and material sciences, and the capability of scientists to interact efficiently and productively for the ultimate benefit of the proposed research. Many of the results obtained have been inspired and influenced by the discussions at meetings and subsequent collaborations, partly through STSM grants. The interdisciplinary environment created by the COST Action has already given results in bilateral or multilateral proposals presented to funding agencies.
The success of the COST Action initiative was due also to the extremely efficient and wise management provided by the Chairman Chryssostomos Chatgilialoglu, the Vice Chairman Philippe Renaud, as well as by the Grant Holder and the COST Office staff.
Disclosure statement
The authors report no conflicts of interest. The authors alone are responsible for the content and writing of this article.
References
- Rabus R, Boll M, Heider J, Meckenstock RU, Buckel W, Einsle O, et al. Anaerobic microbial degradation of hydrocarbons: from enzymatic reactions to the environment. J Mol Microbiol Biotechnol 2016;26:5–28.
- Buckel W, Golding BT. Radical enzymes. In: Chatgilialoglu C, Studer A, eds. Handbook of radicals in chemistry, biology & materials. New York: Wiley; 2012:ch. 50.
- Wilkes H, Buckel W, Golding BT, Rabus R. Metabolism of hydrocarbons in n-alkane-utilizing anaerobic bacteria. J Mol Microbiol Biotechnol 2016;26:138–151.
- Bariotaki A, Kalaitzakis D, Smonou I. Enzymatic reductions for the regio- and stereoselective synthesis of hydroxy-keto esters and dihydroxy esters. Org Lett 2012;14:1792–1795.
- (a) Heider J, Szaleniec M, Martins BM, Seyhan D, Buckel W, Golding BT. Structure and function of benzylsuccinate synthase and related fumarate-adding glycyl radical enzymes. J Mol Microbiol Biotechnol 2016;26:29–44. (b) Seyhan D, Friedrich P, Szaleniec M, Buckel W, Golding BT, Heider JD. Elucidating the stereochemistry of enzymatic benzylsuccinate synthesis with chirally labeled toluene. Angew Chem Int Ed Engl 2016;55:11664–11667.
- Goncharenko KV, Vit A, Blankenfeldt W, Seebeck FP. Structure of the sulfoxide synthase EgtB from the ergothioneine biosynthetic pathway. Angew Chem Int Ed Engl 2015;54:2821–2824.
- Goncharenko KV, Seebeck FP. Conversion of a non-heme iron-dependent sulfoxide synthase into a thiol dioxygenase by a single point mutation. Chem Commun 2016;52:1945–1948.
- Dowling DP, Vey JL, Croft AK, Drennan CL. Structural diversity in the AdoMet radical enzyme superfamily. Biochim Biophys Acta 2012;1824:1178–1195.
- Dragičević I, Barić D, Kovačević B, Golding BT, Smith DM. Non-enzymatic ribonucleotide reduction in the prebiotic context. Chemistry 2015;21:6132–6143.
- Eleftheriadis N, Neochoritis CG, Leus NG, van der Wouden PE, Dömling A, Dekker FJ. Rational development of a potent 15-lipoxygenase-1 inhibitor with in vitro and ex vivo anti-inflammatory properties. J Med Chem 2015;58:7850–7862.
- Wisastra R, Dekker FJ. Inflammation, cancer and oxidative lipoxygenase activity are intimately linked. Cancers (Basel) 2014;6:1500–1521.
- Faus I, Reinhard A, Rackwitz AS, Wolny JA, Schlage K, Wille H-C, et al. Isoprenoid biosynthesis in pathogenic bacteria: nuclear resonance vibrational spectroscopy provides insight into the unusual [4Fe-4S] cluster of the E. coli LytB/IspH protein. Angew Chem Int Ed Engl 2015;54:12584–12587.
- Janthawornpong K, Krasutsky S, Chaignon P, Rohmer M, Poulter CD, Seemann M. Inhibition of IspH, a [4Fe-4S]2+ enzyme involved in the biosynthesis of isoprenoids via the methylerythritol phosphate pathway. J Am Chem Soc 2013;135:1816–1822.
- Krych J, Gebicka L. Catalase is inhibited by flavonoids. Int J Biol Macromol 2013;58:148–153.
- Krych J, Gebicki JL, Gebicka L. Flavonoid-induced conversion of catalase to its inactive form – compound II. Free Radic Res 2014;48:1334–1341.
- Neacsu MV, Matei I, Micutz M, Staicu T, Precupas A, Popa VT, et al. Interaction between albumin and pluronic F127 block copolymer revealed by global and local physicochemical profiling. J Phys Chem B 2016;120:4258–4267.
- Matei I, Ariciu AM, Neacsu MV, Collauto A, Salifoglou A, Ionita G. Cationic spin probe reporting on thermal denaturation and complexation-decomplexation of BSA with SDS. Potential applications in protein purification processes. J Phys Chem B 2014;118:11238–11252.
- Lojek A, Denev P, Číž M, Vasicek O, Kratchanova M. The effects of biologically active substances in medicinal plants on the metabolic activity of neutrophils. Phytochem Rev 2014;13:499–510.
- Cadet J, Ravanat J-L, Martinez G, Medeiros M, Di Mascio P. Singlet oxygen oxidation of isolated and cellular DNA: product formation and mechanistic insights. Photochem Photobiol 2006;82:1219–1225.
- Ravanat J-L, Martinez GR, Medeiros MGH, Di Mascio P, Cadet J. Singlet oxygen oxidation of 2′-deoxyguanosine. Formation and mechanistic insights. Tetrahedron 2016;62:10709–10715.
- Sheu C, Foote CS. Endoperoxide formation in a guanosine derivative. J Am Chem Soc 1993;115:10446–10447.
- Dumont E, Gruber R, Bignon E, Morell C, Moreau Y, Monari A, Ravanat J-L. Probing the reactivity of singlet oxygen with purines. Nucleic Acids Res 2015;44:56–62.
- Dumont E, Grüber R, Bignon E, Morell C, Randa J, Ravanat J-L, Tunon I. Singlet oxygen addition onto guanine: reactivity and structural signature within the B-DNA helix. Chem Eur J 2016;22:12358–12362.
- Grüber R, Monari A, Dumont E. Stability of the guanine endoperoxide intermediate: a computational challenge for density functional theory. J Phys Chem A 2014;118:11612–11619.
- Grüber R, Dumont E. DFT investigation of the formation of linear aminols as the first step toward the induction of oxidatively-generated interstrand cross-link DNA lesions. Theor Chem Acc 2015;134:26.
- Dumont E, Monari A. Interaction of palmatine with DNA: an environmentally controlled phototherapy drug. J Phys Chem B 2015;119:410–419.
- Dumont E, Monari A. Benzophenone and DNA: evidences for a double insertion mode and its spectral signature. J Phys Chem Lett 2013;4:4119–4124.
- Gattuso H, Assfeld X, Monari A. Modeling DNA electronic circular dichroism by QM/MM methods and Frenkel Hamiltonian. Theor Chem Acc 2015;134:36.
- Huix-Rotllant M, Dumont E, Ferré N, Monari A. Photophysics of acetophenone interacting with DNA: why the road to photosensitization is open. Photochem Photobiol 2015;91:323–330.
- Marazzi M, Mai S, Roca-Sanjuan D, Delcey MG, Lindh R, Gonzalez L, Monari A. Benzophenone ultrafast triplet population: revisiting the kinetic model by surface-hopping dynamics. J Phys Chem Lett 2016;7:622–626.
- Marazzi M, Wibowo M, Gattuso H, Dumont E, Roca-Sanjuan D, Monari A. Hydrogen abstraction by photoexcited benzophenone: consequences for DNA photosensitization. Phys Chem Chem Phys 2016;18:7829–7836.
- Gattuso H, Spinello A, Terenzi A, Assfeld X, Barone G, Monari A. Circular dichroism of DNA G-quadruplexes: combining modeling and spectroscopy to unravel complex structures. J Phys Chem B 2016;120:3113–3121.
- Gattuso H, Besancenot V, Grandemange S, Marazzi M, Monari A. From non-covalent binding to irreversible DNA lesions: Nile blue and Nile red as photosensitizing agents. Sci Rep 2016;6:28480.
- Gattuso H, Dumont E, Marazzi M, Monari A. Two-photon absorption DNA sensitization via solvated electron production: unravelling photochemical pathways by molecular modelling and simulations. Phys Chem Chem Phys 2016;18:18598–18606.
- Dumont E, Wibowo M, Roca-Sanjuan D, Garavelli M, Assfeld X, Monari A. Resolving the benzophenone DNA-photosensitization mechanism at QM/MM level. J Phys Chem Lett 2015;6:576–580.
- Bignon E, Gattuso H, Morell C, Dumont E, Monari A. DNA photosensitization by an insider. Photophysics and triplet energy transfer of 5-methyl-2-pyrimidone deoxyribonucleoside. Chem Eur J 2015;21:11509–11516.
- Dumont E, Monari A. Understanding DNA under oxidative stress and sensitization: the role of molecular modeling. Front Chem 2015;3:43.
- Gattuso H, Duchanois T, Besancenot V, Barbieux C, Assfeld X, Becuwe P, et al. Interaction of iron II complexes with B-DNA. Insights from molecular modeling, spectroscopy, and cellular biology. Front Chem 2015;3:67.
- Chatgilialoglu C, Ferreri C, Terzidis MA. Purine 5′,8-cyclonucleoside lesions: chemistry and biology. Chem Soc Rev 2011;40:1368–1382.
- Chatgilialoglu C, Ferreri C, Masi A, Sansone A, Terzidis MA, Tsakos M. A problem solving approach for the diastereoselective synthesis of (5′S)- and (5′R)-5′,8-cyclopurine lesions. Org Chem Front 2014;1:698–702.
- Capobianco A, Caruso T, Fusco S, Terzidis MA, Masi A, Chatgilialoglu C, Peluso A. The association constant of 5′,8-cyclo-2′-deoxyguanosine with cytidine. Front Chem 2015;3:22.
- Pieraccini S, Terzidis MA, Baldassarri EJ, Fragneto G, Mariani P, Masiero S, Chatgilialoglu C. A lipophilic “fully-anti” dodecamer from a (5′S)-5′,8-cyclo-2′-deoxyguanosine. Chem Commun 2014;50:10722–10725.
- Terzidis MA, Chatgilialoglu C. An ameliorative protocol for the quantification of purine 5′,8-cyclo-2′-deoxynucleosides in oxidized DNA. Front Chem 2015;3:47.
- Terzidis MA, Ferreri C, Chatgilialoglu C. Radiation-induced formation of purine lesions in single and double stranded DNA: revised quantification. Front Chem 2015;3:18.
- Terzidis MA, Prisecaru A, Molphy Z, Barron N, Randazzo A, Dumont E, et al. Radical-induced purine lesion formation is dependent on DNA helical topology. Free Radical Res 2016; in press. doi: 10.1080/10715762.2016.1244820
- Chatgilialoglu C, Krokidis MG, Papadopoulos K, Terzidis MA. Purine 5′,8-cyclo-2′-deoxynucleoside lesions in irradiated DNA. Rad Phys Chem 2016;128:74–80.
- Kropache K, Ding S, Terzidis MA, Masi A, Liu Z, Cai Y, et al. Structural basis for the recognition of diastereomeric 5′,8-cyclo-2′-deoxypurine lesions by the human nucleotide excision repair system. Nucleic Acids Res 2014;42:5020–5032.
- Cai Y, Kropachev K, Terzidis MA, Masi A, Chatgilialoglu C, Shafirovic V, et al. Differences in the access of lesions to the nucleotide excision repair machinery in nucleosomes. Biochemistry 2015;54:4181–4185.
- Xu M, Lai Y, Jiang Z, Terzidis MA, Masi A, Chatgilialoglu C, Liu Y. A 5′,8-cyclo-2′-deoxypurine lesion induces trinucleotide repeat deletion via a unique lesion bypass by DNA polymerase beta. Nucleic Acids Res 2014;42:13749–13763.
- Jiang Z, Xu M, Lai Y, Laverde EE, Terzidis MA, Masi A, et al. Bypass of a 5′,8-cyclopurine-2′-deoxynucleoside by DNA polymerase beta during DNA replication and base excision repair leads to nucleotide misinsertions and DNA strand breaks. DNA Repair 2015;33:24–34.
- Ravanat JL, Breton J, Douki T, Gasparutto D, Grand A, Rachidi W, Sauvaigo S. Radiation-mediated formation of complex damage to DNA: a chemical aspect overview. BJR 2014;87:20130715.
- Cadet J, Ravanat J-L, Taverna-Porro M, Menoni H, Angelov D. Oxidatively generated complex DNA damage: tandem and clustered lesions. Cancer Lett 2012;327:5–15.
- Cadet J, Douki T, Ravanat J-L. One-electron oxidation of DNA and inflammation processes. Nat Chem Biol 2006;2:348–349.
- Perrier S, Hau J, Gasparutto D, Cadet J, Favier A, Ravanat J-L. Characterization of lysine-guanine cross-links upon one-electron oxidation of a guanine-containing oligonucleotide in the presence of a trilysine peptide. J Am Chem Soc 2006;128:5703–5710.
- Milligan JR, Aguilera JA, Ly A, Tran NQ, Hoang O, Ward JF. Repair of oxidative DNA damage by amino acids. Nucleic Acids Res 2003;31:6258–6263.
- Milligan JR, Tran NQ, Ly A, Ward JF. Peptide repair of oxidative DNA damage. Biochemistry 2004;43:5102–5108.
- Silerme S, Bobyk L, Taverna-Porro M, Cuier C, Saint-Pierre C, Ravanat JL. DNA-polyamine crosslinks generated upon one electron oxidation of DNA. Chem Res Toxicol 2014;27:1011–51018.
- Gomez Mendoza M, Banyasz A, Douki T, Markovitsi D, Ravanat JL. Direct oxidative damage of naked DNA generated upon absorption of UV radiation by nucleobases. J Phys Chem Lett 2016;7:3945–3948.
- Ravanat JL. Chromatographic methods for the analysis of oxidatively damaged DNA. Free Radic Res 2012;46:479–5491.
- Capobianco A, Caruso T, D’Ursi AM, Fusco S, Masi A, Scrima M, et al. Delocalized hole domains in guanine-rich DNA oligonucleotides. J Phys Chem B 2015;119:5462–5466.
- Borrelli R, Capobianco A, Landi A, Peluso A. Vibronic couplings and coherent electron transfer in bridged systems. Phys Chem Chem Phys 2015;17:30937–30945.
- Nikitaki Z, Nikolov V, Mavragani IV, Mladenov E, Mangelis A, Laskaratou DA, et al. Measurement of complex DNA damage induction and repair in human cellular systems after exposure to ionizing radiations of varying linear energy transfer (LET). Free Rad Res 2016; doi: 10.1080/10715762.2016.1232484.
- Nikitaki Z, Hellweg CE, Georgakilas AG, Ravanat JL. Stress-induced DNA damage biomarkers: applications and limitations. Front Chem 2015;3:35.
- Georgakilas AG, Redon CE, Ferguson NF, Kryston TB, Parekh P, Dickey JS, et al. Systemic DNA damage accumulation under in vivo tumor growth can be inhibited by the antioxidant Tempol. Cancer Lett 2014;353:248–4257.
- Pavlopoulou A, Savva GD, Louka M, Bagos PG, Vorgias CE, Michalopoulos I, Georgakilas AG. Unraveling the mechanisms of extreme radioresistance in prokaryotes: lessons from nature. Mutat Res Rev Mutat Res 2016;767:92–4107.
- Pavlopoulou A, Oktay Y, Vougas K, Louka M, Vorgias CE, Georgakilas AG. Determinants of resistance to chemotherapy and ionizing radiation in breast cancer stem cells. Cancer Lett 2016;380:485–4493.
- Nikitaki Z, Mavragani IV, Laskaratou DA, Gika V, Moskvin VP, Theofilatos K, et al. Systemic mechanisms and effects of ionizing radiation: a new ‘old’ paradigm of how the bystanders and distant can become the players. Semin Cancer Biol 2016;37:438, 77–95.
- Pateras IS, Havaki S, Nikitopoulou X, Vougas K, Townsend PA, Panayiotidis MI, et al. The DNA damage response and immune signaling alliance: is it good or bad? Nature decides when and where. Pharmacol Ther 2015;154:36–56.
- Nikitaki Z, Michalopoulos I, Georgakilas AG. Molecular inhibitors of DNA repair: searching for the ultimate tumor killing weapon. Future Med Chem 2015;7:1543–1558.
- Georgakilas AG, Pavlopoulou A, Louka M, Nikitaki Z, Vorgias CE, Bagos PG, Michalopoulos I. Emerging molecular networks common in ionizing radiation, immune and inflammatory responses by employing bioinformatics approaches. Cancer Lett 2015;368:164–172.
- Bignon E, Gattuso H, Morell C, Dehez F, Georgakilas AG, Monari A, Dumont E. Correlation of bistranded clustered abasic DNA lesion processing with structural and dynamic DNA helix distortion. Nucleic Acids Res 2016;44:8588–8599.
- Gattuso H, Durand E, Bignon E, Morell C, Georgakilas AG, Dumont E, Chipot C, et al. Repair rate of clustered abasic DNA lesions by human endonuclease: molecular bases of sequence specificity. J Phys Chem Lett 2016;7:3760–3765.
- Samadi AK, Bilsland A, Georgakilas AG, Amedei A, Amin A, Bishayee A, et al. A multi-targeted approach to suppress tumor-promoting inflammation. Semin Cancer Biol 2015;35:S151–S184.
- Block KI, Gyllenhaal C, Lowe L, Amedei A, Amin AR, Amin A, et al. Designing a broad-spectrum integrative approach for cancer prevention and treatment. Semin Cancer Biol 2015;35:S276–S304.
- Nikitaki Z, Nikolov V, Mavragani IV, Plante I, Emfietzoglou D, Iliakis G, Georgakilas AG. Non-DSB clustered DNA lesions. Does theory colocalize with the experiment? Rad Phys Chem 2016;128:26–35.
- Zdrowowicz M, Chomicz L, Miloch J, Wiczk J, Rak J, Kciuk G, Bobrowski K. Reactivity pattern of bromonucleosides induced by 2-hydroxypropyl radicals: photochemical, radiation chemical, and computational studies. J Phys Chem B 2015;119:6545–6554.
- Westphal K, Wiczk J, Miloch J, Kciuk G, Bobrowski K, Rak J. Irreversible electron attachment-a key to DNA damage by solvated electrons in aqueous solution. Org Biomol Chem 2015;13:10362–10369.
- Westphal K, Skotnicki K, Bobrowski K, Rak J. Radiation damage to single stranded oligonucleotide trimers labelled with 5-iodopyrimidines. Org Biomol Chem 2016;14:9331–9337.
- Larragy R, Fitzgerald J, Prisecaru A, McKee V, Leonard P, Kellett A. Protein engineering with artificial chemical nucleases. Chem Commun (Camb) 2015;51:12908–12911.
- Molphy Z, Prisecaru A, Slator C, Barron N, McCann M, Colleran J, et al. Copper phenanthrene oxidative chemical nucleases. Inorg Chem 2014;53:5392–5404.
- Molphy Z, Slator C, Chatgilialoglu C, Kellett A. DNA oxidation profiles of copper phenanthrene chemical nucleases. Front Chem 2015;3:28.
- Slator C, Barron N, Howe O, Kellett A. [Cu(o-phthalate)(phenanthroline)] exhibits unique superoxide-mediated NCI-60 chemotherapeutic action through genomic DNA damage and mitochondrial dysfunction. ACS Chem Biol 2016;11:159–171.
- Prisecaru A, Molphy Z, Kipping RG, Peterson EJ, Qu Y, Kellett A, Farrell NP. The phosphate clamp: sequence selective nucleic acid binding profiles and conformational induction of endonuclease inhibition by cationic Triplatin complexes. Nucleic Acids Res 2014;42:13474–13487.
- Ferreri C, Omega 3 fatty acids and bioactive foods: from biotechnology to health promotion. In: Ronald W, ed. Bioactive food as dietary interventions for liver and gastrointestinal disease. London: Academic Press; 2013:401–420.
- Singer SJ, Nicolson GL. The fluid mosaic model of the structure of cell membranes. Science 1972;175:720–731.
- Niki E, Yoshida Y, Saito Y, Noguchi N. Lipid peroxidation: mechanisms, inhibition, and biological effects. Biochem Biophys Res Commun 2005;338:668–676.
- Schaur RJ, Siems W, Bresgen N, Eckl PM. 4-Hydroxy-nonenal – a bioactive lipid peroxidation product. Biomolecules 2015;5:2247–2337.
- Riahi Y, Cohen G, Shamni O, Sasson S. Signaling and cytotoxic functions of 4-hydroxyalkenals. Am J Physiol Endocrinol Metab 2010;299:E879–E886.
- Cohen G, Riahi Y, Shamni O, Guichardant M, Chatgilialoglu C, Ferreri C, et al. Role of lipid peroxidation and PPAR-δ in amplifying glucose-stimulated insulin secretion. Diabetes 2011;60:2830–2842.
- Cohen G, Riahi Y, Sunda V, Deplano S, Chatgilialoglu C, Ferreri C, et al. Signaling properties of 4-hydroxyalkenals formed by lipid peroxidation in diabetes. Free Radic Biol Med 2013;65:978–987.
- Cohen G, Shamni O, Avrahami Y, Cohen O, Broner EC, Filippov-Levy N, et al. Beta cell response to nutrient overload involves phospholipid remodelling and lipid peroxidation. Diabetologia 2015;58:1333–1343.
- Jovanovic O, Pashkovskaya AA, Annibal A, Vazdar M, Burchardt N, Sansone A, et al. The molecular mechanism behind reactive aldehyde action on transmembrane translocations of proton and potassium ions. Free Radical Biol Med 2015;89:1067–1076.
- Lands WE. Metabolism of glycerolipides: a comparison of lecithin and triglyceride synthesis. J Biol Chem 1958;231:883–888.
- Maulucci G, Cohen O, Daniel B, Sansone A, Petropoulou PI, Filou S, et al. Fatty acid-related modulations of membrane fluidity in cells: detection and implications. Free Radic Res 2016; http://dx.doi.org/10.1080/10715762.2016.1231403.
- Matera R, Gabbanini S, Berretti S, Amorati R, De Nicola GR, Iori R, Valgimigli L. Acylated anthocyanins from sprouts of Raphanus sativus cv. Sango: isolation, structure elucidation and antioxidant activity. Food Chem 2015;166:397–406.
- Canistro D, Boccia C, Falconi R, Bonamassa B, Valgimigli L, Vivarelli F, et al. Redox-based flagging of the global network of oxidative stress greatly promotes longevity. J Gerontol A Biol Sci Med Sci 2015;70:936–943.
- Valgimigli L, Sapone A, Canistro D, Broccoli M, Gatta L, Soleti A, Paolini M. Oxidative stress and aging: a non-invasive EPR investigation in human volunteers. Aging Clin Exp Res 2015;27:235–238.
- Amorati R, Valgimigli L. Advantages and limitations of common testing methods for antioxidants. Free Radic Res 2015;49:633–649.
- Fouret G, Tolika E, Lecomte J, Bomafos B, Aoun M, Murphy MP, et al. The mitochondrial-targeted antioxidant, MitoQ, increases liver mitochondrial cardiolipin content in obesogenic diet-fed rats. BBA Bioenerget 2015;1847:1025–1035.
- Jankovic A, Ferreri C, Filipovic M, Ivnovic-Burmazovic I, Stancic A, Otasevic V, et al. Targeting the superoxide/nitric oxide ratio by L-arginine and SOD mimic in rat diabetic skin. Free Radic Res 2016; http://dx.doi.org/10.1080/10715762.2016.1232483.
- Anwar A, Marini M, Abruzzo PM, Bolotta A, Ghezzo A, Visconti P, et al. Quantitation of plasma thiamine, related metabolites and plasma protein damage markers in children with autism spectrum disorders and healthy controls. Free Radic Res 2016; http://dx.doi.org/10.1080/10715762.2016.1239821.
- Hanikoglu F, Hanikoglu A, Kucuksayan E, Alisik M, Gocener AA, Erel O, et al. Dynamic thiol/disulphide homeostasis before and after radical prostatectomy in patients with prostate cancer. Free Radic Res 2016; http://dx.doi.org/10.1080/10715762.2016.1235787.
- Ferreri C, Costantino C, Landi L, Mulazzani QG, Chatgilialoglu C. The thiyl radical mediated isomerization of cis-monounsaturated fatty acid residues in phospholipids: a novel path of membrane damage? Chem Commun 1999;407–408. doi: 10.1039/A809674K.
- Chatgilialoglu C, Ferreri C, Melchiorre M, Sansone A, Torreggiani A. Lipid geometrical isomerism: from chemistry to biology and diagnostics. Chem Rev 2014;114:255–284.
- Tartaro Bujak I, Chatgilialoglu C, Ferreri C, Valgimigli L, Amorati R, Mihalievic B. The effect of aromatic amines and phenols in the thiyl-induced reactions of polyunsaturated fatty acids. Rad Phys Chem 2016;124:104–110.
- Chatgilialoglu C, Ferreri C, Lykakis IN, Mihaljevic B. Biomimetic thiyl radical chemistry by gamma-irradiation of micelles and vesicles containing unsaturated fatty acids. Isr J Chem 2014;54:242–247.
- Tartaro Bujak I, Mihaljevic B, Ferreri C, Chatgilialoglu C. The influence of antioxidants in the thiyl radical induced lipid peroxidation and geometrical isomerization in micelles of linoleic acid. Free Radic Res 2016; http://dx.doi.org/10.1080/10715762.2016.1231401.
- Lykakis IN, Ferreri C, Chatgilialoglu C. Biomimetic chemistry on the protection of cis phospholipids from the thiyl radical isomerization by common antioxidants. Arkivoc 2015;2015:140–153.
- Sansone A, Melchiorre M, Chatgilialoglu C, Ferreri C. Hexadecenoic fatty acid isomers: a chemical biology approach for human plasma biomarker development. Chem Res Toxicol 2013;26:1703–1709.
- Sansone A, Tolika E, Louka M, Sunda V, Deplano S, Melchiorre M, et al. Hexadecenoic fatty acid isomers in human blood and their relevance for the obesity lipidomic phenotype. PLoS One 2016;511:e0152378.
- Mendeluk GR, Cohen MI, Ferreri C, Chatgilialoglu C. Nutrition and reproductive health: sperm versus erythrocyte lipidomic profile and ω-3 intake. J Nutr Metab 2015;Article ID 670526.
- Bianchi AR, Ferreri C, Ruggiero S, Deplano S, Valentina S, Galloro G, et al. Automodification of PARP and fatty acid-based membrane lipidome as a promising integrated biomarker panel in molecular medicine. Biomarkers Med 2016;10:229–242.
- Cort A, Ozben T, Sansone A, Barata-Vallejo S, Chatgilialoglu C, Ferreri C. Bleomycin-induced trans lipid formation in cell membranes and in liposome models. Org Biomol Chem 2015;13:1100–1105.
- Cort A, Ozben T, Melchiorre M, Chatgilialoglu C, Ferreri C, Sansone A. Effects of bleomycin and antioxidants on the fatty acid profile of testicular cancer cell membranes. Biochim Biophys Acta 2016;1858:434–441.
- Barata-Vallejo S, Ferreri C, Zhang T, Permentier H, Bischoff R, Bobrowski K, Chatgilialoglu C. Radiation chemical studies of Gly-Met-Gly in aqueous solution. Free Radic Res 2016; http://dx.doi.org/10.1080/10715762.2016.1231402.
- Lakk-Bogath D, Speier G, Surducan M, Silaghi-Dumitrescu R, Simaan AJ, Faure B, Kaizer J. Comparison of heme and nonheme iron-based 1-aminocyclopropane-1-carboxylic acid oxidase mimics: kinetic, mechanistic and computational studies. RSC Adv 2015;5:2075–2079.
- Chenneberg L, Baralle A, Daniel M, Fensterbank L, Goddard JP, Ollivier C. Visible light photocatalytic reduction of O-thiocarbamates: development of a tin-free Barton-McCombie deoxygenation reaction. Adv Synth Catal 2014;356:2756–2762.
- Corcé V, Chamoreau LM, Derat E, Goddard JP, Ollivier C, Fensterbank L. Silicates as latent alkyl radical precursors: visible-light photocatalytic oxidation of hypervalent bis-catecholato silicon compounds. Angew Chem Int Ed Engl 2015;54:11414–11418.
- Kornarakis I, Lykakis IN, Vordos N, Armatas GS. Efficient visible-light photocatalytic activity by band alignment in mesoporous ternary polyoxometalate–Ag2S–CdS semiconductors. Nanoscale 2014;6:8694–8703.
- Kafka F, Holan M, Hidasová D, Pohl R, Císařová I, Klepetářová B, Jahn U. Oxidative catalysis using the stoichiometric oxidant as a reagent: an efficient strategy for single-electron-transfer-induced tandem anion-radical reactions. Angew Chem Int Ed Engl 2014;53:9944–9948.
- Bagi N, Kaizer J, Speier G. Oxidation of thiols to disulfides by dioxygen catalyzed by a bioinspired organocatalyst. RSC Adv 2015;5:45983–45986.
- Bors I, Kaizer J, Speier G. Kinetics and mechanism of PPh3 oxygenation with 3O2 catalyzed by a 1,3,2-oxazaphosphole as flavin mimic. RSC Adv 2014;4:16928–16930.
- Bors I, Kaizer J, Speier G, Giorgi M. Carbon dioxide as a primary oxidant and a C1 building block. RSC Adv 2014;4:45969–45972.
- Symeonidis TS, Tamiolakis I, Armatas GS, Lykakis IN. Green photocatalytic organic transformations by polyoxometalates vs. mesoporous TiO2 nanoparticles: selective aerobic oxidation of alcohols. Photochem Photobiol Sci 2015;14:563–568.
- Alvarez-Dorta D, Leon EI, Kennedy AR, Martin A, Perez-Martin I, Suarez E. Easy access to modified cyclodextrins by an intramolecular radical approach. Angew Chem Int Ed Engl 2015;54:3674–3678.
- Soulard V, Dénès F, Renaud P. Effect of Bronsted Acids on the thiophenol-mediated radical addition-translocation-cyclization process for the preparation of pyrrolidine derivatives. Free Radic Res 2016; http://dx.doi.org/10.1080/10715762.2016.1223294.
- Paz NR, Rodriguez-Sosa D, Valdes H, Marticorena R, Melian D, Copano MB, et al. Chemoselective intramolecular functionalization of methyl groups in nonconstrained molecules promoted by N-iodosulfonamides. Org Lett 2015;17:2370–2373.
- Jagtap PR, Ford L, Deister E, Pohl R, Císařová I, Hodek J, et al. Highly functionalized and potent antiviral cyclopentane derivatives formed by a tandem process consisting of organometallic, transition-metal-catalyzed, and radical reaction steps. Chem Eur J 2014;20:10298–10304.
- Holan M, Pohl R, Císařová I, Klepetářová B, Jones PG, Jahn U. Highly functionalized cyclopentane derivatives by tandem michael addition/radical cyclization/oxygenation reactions. Chem Eur J 2015;20:9877–9888.
- Amatov T, Pohl R, Cisařová I, Jahn U. Synthesis of bridged diketopiperazines by using the persistent radical effect and a formal synthesis of bicyclomycin. Angew Chem Int Ed Engl 2015;54:12153–12157.
- Amatov T, Gebauer M, Pohl R, Cisařová I, Jahn U. Oxidative radical cyclizations of diketopiperazines bearing an amidomalonate unit. Heterointermediate reaction sequences toward the asperparalines and stephacidins. Free Radic Res 2016; http://dx.doi.org/10.1080/10715762.2016.1223295.
- Gloor CS, Dénès F, Renaud P. Memory of chirality in reactions involving monoradicals. Free Radic Res 2016; https://doi.org/http://dx.doi.org/10.1080/10715762.2016.1232485.