Abstract
Oxidative stress is an important pathophysiological mechanism in the development of numerous cardiovascular disorders. To improve therapy and preventive strategies, clinicians need a better understanding of the underlying pathophysiological mechanisms of congenital heart diseases (CHD). The objective of this meta-analysis was to determine whether oxidative stress is elevated in patients with CHD compared to healthy controls, and to evaluate whether a difference in oxidative stress parameters can be observed between patients with cyanotic (cCHD) and acyanotic CHD (aCHD). Therefore, 21 studies investigating oxidative stress in peripheral blood of both children and adults with CHD were reviewed. Different methods to assess the oxidant status were compared and divided into three categories: pro-oxidative or anti-oxidative stress markers and the ratio of pro-to-anti oxidative stress markers. This meta-analysis showed elevated oxidative stress levels in patients with CHD, and more specifically in patients with cCHD. Moreover, this indicates that there could be potential in oxidative stress measurements as a new biomarker of disease severity. Further research will be needed to clarify the exact role of oxidative stress and its contributors in CHD in order to get a better and more in-depth understanding of the underlying pathophysiology of CHD, especially the higher susceptibility of the right ventricle (RV) to progress to heart failure (HF). This could facilitate the development of antioxidant treatments and RV-specific HF therapies, which are necessary to improve survival in these patients and could be of particular importance in cCHD.
Introduction
Congenital heart diseases (CHDs) compromise a wide range of cardiac malformations [Citation1]. Medical and surgical advances have dramatically increased the survival of patients with CHD leading to a continuously growing prevalence of CHD in children, adolescents and adults [Citation2,Citation3]. Nevertheless, patients suffering from CHD have lower physical fitness levels [Citation3–5], reduced quality-of-life [Citation6] and worse prognosis [Citation7,Citation8] compared to healthy individuals of similar age. In addition to evaluation of the left ventricle (LV), life-long evaluation of the less studied right ventricle (RV) has shown to be a cornerstone in managing CHD prognosis in such patients [Citation1,Citation4,Citation6,Citation9]. To improve therapy and preventive strategies, clinicians need a better understanding of the underlying pathophysiological mechanisms of CHD [Citation10].
Development and progression of heart failure (HF) is the main cause of morbidity and mortality in this population [Citation11,Citation12]. Patients with HF induced by CHD and patients with HF induced by other etiologies share many characteristics [Citation10]. In patients with HF induced by other etiologies, increased oxidative stress is implicated in pathogenesis of cardiac injury and disease progression [Citation13,Citation14]. Moreover, a continuously growing body of evidence shows that oxidative stress is important in the pathogenesis of several other types of cardiovascular diseases [Citation15]. Accordingly, oxidative stress becomes an attractive therapeutic target [Citation15].
Oxidative stress refers to an imbalance between reactive oxygen species (ROS) production and the body’s antioxidant defenses [Citation16]. Oxidative stress may be of particular concern in the pathogenesis of CHD. First, oxidative stress during pregnancy can be teratogenic and contribute to CHD in newborns [Citation17]. Second, although cellular remodeling in response to pressure overload is similar in the RV and LV, the RV is more susceptible to oxidative stress [Citation18,Citation19]. In many patients with repaired or palliated CHD and in patients with pulmonary hypertension, the RV of the heart is uniquely at risk. Third, as hypoxic conditions favor an increase in ROS generation [Citation20], one can expect that cyanosis leads to an even higher susceptibility to oxidative stress in patients with cyanotic CHD (cCHD), who possess poorer clinical outcomes compared with those having acyanotic CHD (aCHD) [Citation21]. Therefore, identifying and developing new biomarkers of disease severity in CHD and progression to HF should be considered, given the limitations of clinical assessment and imaging modalities in determining the optimal timing for surgical intervention [Citation18]. Moreover, understanding the molecular mechanisms for the higher susceptibility of the RV to progress to HF in patients with CHD could facilitate the development of RV-specific HF therapies, which is a critical need given that standard HF therapies are ineffective in treating RV failure and have failed to improve survival in these patients.
The objective of this meta-analysis was to determine whether oxidative stress is elevated in patients with CHD compared to healthy controls, and to evaluate whether a difference in oxidative stress parameters can be observed between patients with cCHD and aCHD. Therefore, studies investigating oxidative stress in peripheral blood of both children and adults with CHD were reviewed. Different methods to assess the oxidant status were compared and divided into three categories: pro-oxidative or anti-oxidative stress markers and the ratio of pro-to-anti oxidative stress markers.
Methods
This meta-analysis was written following the guidelines of the Preferred Reporting Items for Systematic Reviews and Meta-Analysis (PRISMA) statement [Citation22].
Search strategies and study selection
Papers and/or abstracts published in English were searched using PubMed and TRIP database (free version) between October 2022 and December 2022 and updated in May 2023, including the respective keywords and respective MeSH terms mentioned in . The searches yielded 247 papers on PubMed and 128 on TRIP database. Duplicates were eliminated and remaining reports were screened based on title and abstract. depicts the in- and exclusion criteria. In case of ambiguity or doubt, the report was kept and full text was screened.
Table 1. In- and exclusion criteria for papers in this meta-analysis.
Only controlled studies that compared oxidative stress markers in patients with CHD with healthy controls or in patients with cCHD versus aCHD were included. However, papers referenced in systematic reviews related to broader topics (e.g. the top most cited biomarkers in CHD) or broader patient populations (e.g. cardiovascular disease) were also screened and included when fulfilling the above mentioned criteria.
Because oxidative stress is part of the stress response during and after surgery and cardiopulmonary bypass [Citation16,Citation23], studies measuring oxidative stress during and shortly after surgery or cardiopulmonary bypass were excluded. However, when in these studies a baseline (i.e. pre-operative) comparison was made in oxidative stress levels of patients with CHD with a control group or between cCHD and aCHD, this baseline comparison was included in this meta-analysis.
The reference lists of the included articles were also checked which identified five additional relevant studies. Of these studies, one article [Citation24] was not retained because the data presented were the same as those in a previous paper of the same authors [Citation25]. The systematic reviewing process has been performed by an expert on both oxidative stress and CHD (IV). shows the flowchart of the systematic review procedure.
Data extraction
Data were entered independently by two reviewers (IV and WH) into an a priori designed data extraction table. Inter-reviewer differences were discussed until consensus was reached. The following study characteristics were extracted from the articles: name of first author, publication year, measured parameters of oxidative stress and its exact data in healthy controls and CHD. It was always mentioned which types of CHD were studied, whether there was a history of surgery and the age group involved. When a division between cCHD and aCHD had already been made in the articles, this subdivision was adopted as such. If no statement on cyanosis was made by the authors of an included manuscript, patients were categorized according to their CHD at birth (e.g. tetralogy of Fallot as cCHD and left-to-right shunts as aCHD). Furthermore, the number of subjects per group was noted as well as the exact p-value in case of statistical significance.
Whenever methods or outcome data were not reported clearly, the corresponding author was contacted. In case the exact outcome data were not shown or could not be obtained from the corresponding author, data were derived from the figures or graphs. To avoid artificially increased certainty and thus erroneously small 95% confidence intervals, group numbers were adapted, in case of data extracted from multi-armed studies. When results from an included study were reported in medians and percentiles or inter quartile ranges, a fixed protocol was followed to convert these data to means and standard deviations (). depicts all data and related information necessary to conduct the meta-analyses.
Figure 2. Protocol followed to convert data to mean ± standard deviation. Abbreviations: m: median; max: maximum; min: minimum; p5: 5th percentile; p95: 95th percentile; Q1: 1st quartile; Q3: 3rd quartile; var: variance; µ: mean; σ: standard deviation [Citation26–28].
![Figure 2. Protocol followed to convert data to mean ± standard deviation. Abbreviations: m: median; max: maximum; min: minimum; p5: 5th percentile; p95: 95th percentile; Q1: 1st quartile; Q3: 3rd quartile; var: variance; µ: mean; σ: standard deviation [Citation26–28].](/cms/asset/1206f353-7717-446b-aa98-2da14d086146/ifra_a_2284639_f0002_c.jpg)
Table 2. Summary of studies on the presence of oxidative stress in peripheral blood of patients with CHD (grouped by congenital pathology).
Methodological quality assessment
Two independent investigators (IV and WH) assessed the quality of the included studies with The Quality Assessment Tool for Observational Cohort and Cross-Sectional Studies from the National Heart, Lung, and Blood Institute [Citation32] (). The “exposures of interest” in the included papers were the specific CHD and the “the outcomes being investigated” were the oxidative stress parameters. Because CHD is present at birth and the assessment of oxidative stress parameters always takes place later in life, the answers to questions 6 and 7 of this assessment tool were always “yes.” By “different levels of the exposure” we understood different types of CHD or degrees of severity, whether expressed as categories (e.g. cCHD versus aCHD, or with or without pulmonary arterial hypertension) or continuous variables (e.g. correlations with 6 min walking distance or echocardiographic parameters of ventricular function).
Table 3. Methodologic quality of each included article, scored with the quality assessment tool for observational cohort and cross-sectional studies from the National heart, Lung, and blood Institute.
Question 9 of the assessment tool asked if exposure measures were clearly defined and implemented consistently across all study participants. If a study focused on one or more specific or relatively homogeneous groups of CHD (e.g. tetralogy of Fallot, ventricular septal defect, …), the answer was “yes.” However, if the study population was a mix of different CHD the answer was “no” because of the heterogeneity between the different CHD and therefore inconsistency across the study participants. Finally, since the point of interest was differences in oxidative stress status depending on the presence of CHD (CHD versus no CHD or cCHD versus aCHD), and the included studies therefore involve one-off measurements of oxidative stress parameters, questions 10 and 13 were not applicable. One article [Citation36] was published in Chinese. Scoring was conducted on the basis of the English abstract, and filled in “cannot determine” where the information was missing. No articles were excluded based on quality assessment.
Statistical analysis
The extracted data were entered into the CMA-2 software (Comprehensive Meta-Analysis second version, Biostat, Englewood, USA). Expecting an important degree of between studies heterogeneity (due to different measurement techniques and specific patient characteristics, e.g. age), a random-effects model with inversed variance method was chosen to pool the individual study results and to examine the overall weighted mean effect size of levels of (anti)oxidative stress markers between groups. Effect sizes were calculated as standardized mean differences and expressed as Hedges’ g to correct for overestimating the true effect in small study samples. The 95% confidence intervals (95% CI) were calculated around the effect sizes of the individual studies and the overall weighted mean estimate. The Cochran’s Q test, its degrees of freedom (df) and its corresponding p-value were used to test for between study heterogeneity. Higgins’ I2 statistic was assessed to evaluate the amount of heterogeneity across studies. I2 values around 75, 50 and 25% were interpreted as high, moderate and low respectively.
Results of the meta-analyses were presented as forest-plots showing the effect-sizes of the individual studies and the overall weighted mean estimate (diamond) with corresponding confidence intervals. Sensitivity analyses, omitting articles with extremely large effect sizes or studies for which results were extracted from graphs or for which results were recalculated from medians to means, were conducted to evaluate the robustness of the calculated overall weighted mean effect size against such flawed study results. p-Values less than 0.05 were considered significant for all statistical analyses.
Two meta-analyses were conducted based on 21 papers. One meta-analysis assessed the difference in oxidative stress between CHD patients and healthy controls while another meta-analysis evaluated the difference in oxidative stress markers between patients with cCHD and patients with aCHD.
Results
Methods to assess oxidative stress in blood from patients with CHD
Different approaches and methodologies have been used to study oxidative stress in blood of patients with CHD (). ROS are a natural byproduct of oxygen metabolism. Under physiological conditions, ROS regulate multiple cellular processes like proliferation, migration, and differentiation [Citation37], and superoxide anion radical (O2•−) is the most common oxygen free radical in the human body [Citation10]. O2•− induces the formation of several other reactive species, like peroxynitrite (ONOO-), or hydroxyl radical (HO•) [Citation38]. ROS lead to oxidation of biomolecules like DNA, proteins and lipids [Citation38]. This oxidation of DNA has been evaluated in patients with CHD by measuring blood levels of 8-hydroxy-2′-deoxyguanosine (8-OHdG) and lymphocytic comet tail assay, protein oxidation via detection of levels of carbonyl moieties and protein carbonyl (PCO) and via activity of low-molecular-weight protein tyrosine phosphatase (LMW-PTP), and lipid oxidation by determining serum levels of thiobarbituric acid reactive substances (TBARS) and malondialdehyde (MDA) respectively.
Figure 3. Schematic figure of the used methods to assess oxidative stress in blood of patients with CHD. Abbreviations: 8-OHdG: 8-hydroxy-2'-deoxyguanosine; ADMA: asymmetric dimethylarginine; eNOS: endothelial nitric oxide synthase; FA: folic acid; γGT: gammaglutamyl transferase; GSH: glutathione; GSSG: glutathione disulfide; GP: glutathione peroxidase; H2O: water; H2O2: hydrogen peroxide; H2S: hydrogen sulfide; (Met-)Hb: (met)hemoglobin; Hcy: homocysteine; HO•: hydroxyl radical; im: ischemia-modified; LMW-PTP: low-molecular-weight protein tyrosine phosphatase; Met(-SO): methionine (sulfoxide); MHR: methemoglobin reductase; NO: nitric oxide; O2: oxygen; O2•−= superoxide anion radical; ONOO-: peroxynitrite; PCO: protein carbonyl; ROS: reactive oxygen species; SDMA: symmetric dimethylarginine; SOD: superoxide dismutase; TBARS: thiobarbituric acid reactive substances.
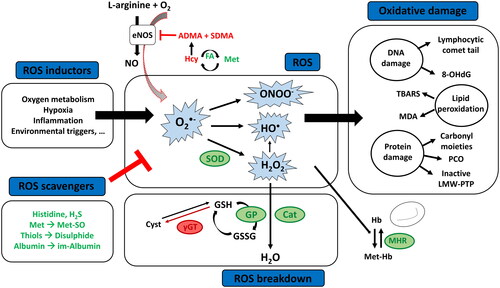
Increased blood levels of ROS are formed during hypoxia [Citation25], inflammation, vascular calcification [Citation37], and environmental triggers [Citation39]. Moreover, endothelial nitric oxide synthase (eNOS) produces O2•− instead of transforming L-arginine into nitric oxide (NO), when there is a deficiency of L-arginine or an accumulation of its analogs and endogenous eNOS inhibitors asymmetrical or symmetric dimethylarginine (ADMA, SDMA) [Citation40]. Homocysteine (Hcy) inhibits the enzyme responsible for hydrolyzation of ADMA, thereby causing an accumulation of ADMA which also makes Hcy a blood marker of oxidative stress in humans [Citation41].
ROS or the damage caused by ROS are mitigated by antioxidants, which can be enzymatic or non-enzymatic [Citation39]. The “first line defense antioxidants” obstruct free radicals’ formation in which three key enzymes are involved: superoxide dismutase (SOD), catalase and glutathione peroxidase (GP). These enzymes respectively dismutate O2•− and breakdown hydrogen peroxides and hydroperoxides to harmless molecules [Citation38]. Glutathione (GSH) acts as a coenzyme for GP and is hydrolyzed by gamma-glutamyl transferase (γGT) making γGT another blood marker of oxidative stress in patients with CHD [Citation30].
Another important group of antioxidants are the “scavenging antioxidants.” These antioxidants scavenge active radicals to inhibit chain initiation and break chain propagation reactions. They neutralize free radicals by donating electrons to them, and in the process become free radicals themselves but with less damage [Citation38]. Changes in scavenging antioxidants that have been studied in blood of patients with CHD are histidine, hydrogen sulfide (H2S), methionine, thiols and albumin. By neutralizing ROS, the sulfur of methionine can be oxidized to sulfoxide (Met-SO) [Citation42,Citation43] and thiols can undergo oxidation and form disulfide bonds [Citation35], and under conditions of high oxidative stress, a metabolic protein variant known as ischemia-modified albumin is formed [Citation33].
In CHD, two more antioxidants have been studied: methemoglobin reductase (MHR) and folic acid. MHR is responsible for the conversion of methemoglobin (Met-Hb), which is elevated in conditions of oxidative stress, to deoxyhemoglobin [Citation44]; and folic acid is responsible for the conversion of Hcy to methionine.
Instead of measuring these different individual oxidant and antioxidant molecules, some authors chose to determine total oxidant status (TOS) and total antioxidant status/capacity/activity (TAS, TAC or TAOA). The oxidative stress index (OSI) is used to evaluate the balance between oxidation and antioxidation [Citation16].
Presence of oxidative stress in patients with CHD
The meta-analyses () showed that there is more oxidative stress in patients with CHD than in healthy controls. This difference is statistically significant for pro-oxidative stress markers, anti-oxidative stress markers and the ratio of pro-to-anti oxidative stress markers:
Figure 4. Forest plots of the effects found in the individual studies and the overall effect of pro-oxidative (a), anti-oxidative (B) and the ratio pro-to-anti oxidative stress markers (C) between patients born with CHD and healthy controls. Abbreviations: 8-OHDG: 8-hydroxy-2'-deoxyguanosine; ADMA: asymmetric dimethylarginine; CHD: congenital heart disease; CTL: comet tail length; DIS: disulfide; DNA-H: DNA in head; DNA-T: DNA in tail; FA: folic acid; GammaGT: gamma-glutamyl transferase; H2S: hydrogen sulfide; HCY: homocysteine; HD: head diameter; HIS: histidine; IOC: intensity of chemiluminescence; im-ALB: ischemia-modified albumin; L-ARG: L-arginine; LMW-PTP: low-molecular-weight protein tyrosine phosphatase; MDA: malondialdehyde; MET(-SO): methionine (sulfoxide); MHR: methemoglobin reductase; NT: native thiol; OSI: oxidative stress index (TOS/TAC); PAH: pulmonary arterial hypertension; PCO: protein carbonyl; SO: superoxide; SOD: superoxide dismutase; TAC/S: total antioxidant capacity/status; TAOA: total antioxidant activity; TBARS: thiobarbituric acid reactive substances; TCL: total comet length; TOS: total oxidant status; TT: total thiol
Pro-oxidative stress markers
The standardized mean difference (Hedge’s g) of the pro-oxidative stress markers between patients with CHD and healthy controls was 1.23 with a 95% confidence interval (95%CI) ranging from 0.89 to 1.56. The Z-value was 7.19 and the p-value (two tailed) was <0.001. Heterogeneity analysis showed high and statistically significant heterogeneity between studies (Cochran’s Q = 439.752; degrees of freedom of Q (df(Q)) = 45; p < 0.001; I2 = 89.767).
One of the analyzed studies [Citation45] appeared to have a remarkably large effect size. Conducting a sensitivity analysis by removing this study still revealed statistically significant more pro-oxidative stress markers in patients with CHD as compared to healthy controls with Hedge’s g of 1.11 (95%CI = 0.79 to 1.43, p < 0.001), but the heterogeneity did not decrease (Q = 373.14, p < 0.001, I2 = 89.01).
Even when all studies for which we had to extract data from figures or to convert median to mean values [Citation30,Citation34,Citation45,Citation46] were excluded in a second sensitivity analysis, neither the effect size (Hedge’s g = 1.22, 95%CI = 0.79–1.66, p < 0.001) nor the heterogeneity (Q = 345.31, p < 0.001, I2 = 89.57) changed importantly.
Anti-oxidative stress markers
The standardized mean difference (Hedge’s g) of the anti-oxidative stress markers between CHD and healthy controls was −0.83 with a 95% CI of −1.21 to −0.46. The Z-value was −4.34 and the p-value (two tailed) was <0.001. Heterogeneity analysis showed high and statistically significant heterogeneity between studies (Cochran’s Q = 147.61; df(Q) = 24; p < 0.001; I2 = 83.74).
Again, one of the analyzed studies [Citation45] appeared to have a remarkably large effect size. Conducting a sensitivity analysis by removing this study still revealed statistically significant less anti-oxidative stress markers in patients with CHD as compared to healthy controls, but the effect size decreased to Hedge’s g of −0.56 (95%CI = −0.85 to −0.27, p < 0.001). By performing this analysis, also heterogeneity decreased, however only slightly, to Q = 79.48 (p < 0.001, I2 = 74.84).
In a second sensitivity analysis another study was also removed [Citation46] because reported data had to be converted to mean ± standard deviation. This analysis did not change importantly Hedge’s g (−0.58 with 95% = −0.88 to −0.28), but the heterogeneity decreased slightly (Q = 77.21, p < 0.001, I2 = 75.39).
Ratio pro-to-anti oxidative stress markers
The standardized mean difference (Hedge’s g) of the ratio of pro- to anti-oxidative stress markers between patients with CHD compared to healthy controls was 1.30 with a 95% CI ranging from 0.34 to 2.26. The Z-value was 2.66 and the p-value (two tailed) was <0.001. Heterogeneity analysis showed high and statistically significant heterogeneity between studies (Cochran’s Q = 138.22; df(Q) = 8; p < 0.001; I2 = 94.21).
In a first sensitivity analysis, one study [Citation47] was excluded because of a remarkably large effect size compared to the other studies. Overall effect size decreased to Hedge’s g of 0.55 (95% CI = 0.20–0.90, p = 0.002) and to a lower heterogeneity (Q = 15.83, p = 0.027, I2 = 55.79).
In a second sensitivity analysis, an additional study was removed [Citation34] because data were extracted from figures. This analysis did not importantly change Hedge’s g (0.54 with 95%CI = 0.13–0.94, p = 0.010) nor the heterogeneity (Q = 15.73, p = 0.015, I2 = 61.86).
Effect of cyanosis
The meta-analyses () showed evidence for more oxidative stress in patients with a cCHD compared to patients with aCHD. This difference is statistically significant for pro-oxidative stress markers and the ratio of pro-to-anti oxidative stress markers, but not for anti-oxidative stress markers:
Figure 5. Forest plots of the effects found in the individual studies and the overall effect of pro-oxidative (a), anti-oxidative (B) and the ratio pro-to-anti oxidative stress markers (C) between patients born with cCHD and aCHD. Abbreviations: ADMA: asymmetric dimethylarginine; (a/c)CHD: (acyanotic/cyanotic) congenital heart disease; CM: carbonyl moieties; CTL: comet tail length; DIS: disulfide; DNA-H: DNA in head; DNA-T: DNA in tail; FA: folic acid; HCY: homocysteine; HD: head diameter; IOC: intensity of chemiluminescence; MDA: malondialdehyde; NT: native thiol; OSI: oxidative stress index (TOS/TAC); PAH: pulmonary arterial hypertension; PCO: protein carbonyl; SO: superoxide; SOD: superoxide dismutase; TAC/S: total antioxidant capacity/status; TAOA: total antioxidant activity; TBARS: thiobarbituric acid reactive substances; TCL: total comet length; TOS: total oxidant status; TT: total thiol
Pro-oxidative stress markers
The standardized mean difference (Hedge’s g) of the pro-oxidative stress markers between cCHD and aCHD was 0.56 with a 95% CI ranging from 0.16 to 0.96. The Z-value was 2.75 and the p-value (two tailed) was 0.006. Heterogeneity was high and statistically significant (Cochran’s Q = 67.70; df(Q) = 19; p < 0.001; I2 = 71.94).
One of the analyzed studies [Citation45] appeared to have a remarkably large effect size. Conducting a sensitivity analysis by removing this study still revealed statistically significant more pro-oxidative stress markers in cCHD with Hedge’s g of 0.64 (95%CI = 0.37 to 0.91, p < 0.001) but low to moderate heterogeneity (Q = 27.25, p = 0.055, I2 = 37.60).
Excluding both studies for which data were extracted from figures and median was converted to mean [Citation29,Citation45] in a second sensitivity analysis, did not importantly change the effect size (Hedge’s g = 0.64, 95%CI = 0.33–0.966, p < 0.001) nor the heterogeneity (Q = 27.04, p = 0.028, I2 = 44.52).
Anti-oxidative stress markers
The anti-oxidative stress markers were not statistically significant different between patients with cCHD compared to aCHD. The standardized mean difference (Hedge’s g) of the anti-oxidative stress markers between these groups was 0.24 with a 95% CI of −0.63 to 1.12. The Z-value was 0.55 and the p-value (two tailed) was 0.584. Heterogeneity analysis showed high and statistically significant heterogeneity between studies (Cochran’s Q = 69.885; df(Q) = 7; p < 0.001; I2 = 89.98).
Again, the same one study [Citation45] appeared to have a remarkably large effect size. Conducting a sensitivity analysis by removing this study lead to a lower heterogeneity (Q = 14.90, p = 0.011; I2 = 66.45), but did not importantly change the effect size (Hedge’s g = 0.13, 95%CI = −0.34 to 0.61, p = 0.586).
Ratio pro-to-anti oxidative stress markers
In addition to the pro-oxidative stress markers, also the ratio of pro-to-anti oxidative stress markers showed arguments for more oxidative stress in blood of patients with cCHD as compared to patients with aCHD. The standardized mean difference (Hedge’s g) of the ratio of pro- to anti-oxidative stress markers was 0.60 with a 95% CI ranging from 0.05 to 1.16. The Z-value was 2.13 and the p-value (two tailed) was 0.033. Heterogeneity analysis showed moderate albeit not statistically significant heterogeneity between studies (Cochran’s Q = 5.124; df(Q) = 2; p = 0.077; I2 = 60.965). Because of the low number of studies, no sensitivity analysis has been performed.
Discussion
There are only nineteen reports presenting data comparing oxidative stress parameters in blood of CHD patients with healthy controls and nine reports comparing cCHD and aCHD. Studies have been performed in broad patient populations consisting of multiple CHDs, as well as in narrow patient populations focused on one particular type of CHD. The study populations included in the present meta-analysis consisted of children (<18 years of age) in fifteen and of adults (≥18 years) in four out of 21 reports respectively. One author group [Citation41] examined children and young adults (18–26 years) and another paper [Citation30] included adolescents (14–18 years) and adults.
To date, most (i.e. fifteen out of nineteen) studies claim that there is more oxidative stress in the peripheral blood of patients with CHD than in healthy controls. This was confirmed by this meta-analysis, suggesting that oxidative stress is common in patients suffering from CHD and might be important in its pathophysiology. Only four studies included in this meta-analysis showed that there was no significant difference in oxidative stress in the peripheral blood of patients with CHD and healthy individuals [Citation10,Citation16,Citation34,Citation48] of which two [Citation10,Citation48] have studied an adult population. Unfortunately, the groups were too small to perform a sub-analysis by age or specific type of CHD. Nevertheless, we were able to perform a separate analysis for cyanosis. This meta-analysis showed that there is more oxidative stress in patients with cCHD compared to patients with aCHD. Chronic hypoxia of cCHD results in a downregulation of antioxidant defenses and hence leads to a greater susceptibility to oxidative stress [Citation21,Citation49,Citation50]. In fact, oxygen saturations were found to be negatively correlated with MDA and protein carbonyl [Citation21] and oxygen saturations and hemoglobin levels positively correlated with methionine sulfoxide/methionine values [Citation43]. However, as oxidative stress was observed in both conditions of cCHD and aCHD (), it would not be the only factor responsible for oxidative stress in patients with CHD. On the other hand, since it is known that children having cCHD also possess poorer clinical outcomes compared with those having aCHD [Citation21], there could be potential in oxidative stress measurements as a new biomarker of disease severity. Identifying and developing new clinical blood biomarkers of the progression to HF is recommended, especially for the RV, given the limitations of clinical assessment and imaging modalities in determining the optimal timing for surgical intervention [Citation18].
Nevertheless, there are numerous aspects to be considered in the evaluation of oxidative stress in blood of humans. The oxidative stress status assessment is challenging, due to the extremely variable and dynamic nature of the pathophysiological mechanisms underlying oxidative stress. There is no biomarker in absolute that is more reliable than another. Therefore, no oxidative stress biomarker can be employed as a “gold standard.” In addition, many other aspects regarding preanalytical (e.g. stability, interferences, handling and storage), analytical (e.g. assay/method choice, quality control), and postanalytical (e.g. reference ranges-at least cut-off) issues remain to be further evaluated [Citation51]. Furthermore, oxidative stress leads to cellular damage and affects all organs and tissues [Citation47]. The goal of this meta-analysis was to study oxidative stress levels in the blood of patients with CHD, which provides a representation of possible general stress originating from blood cells and the endothelium. It is important to note that these measurements in peripheral blood may reflect the contribution of multiple organs, but do not indicate changes in specific tissues or organs. Nevertheless, although beyond the scope of this meta-analysis, there are already indications for oxidative stress in, for example, the myocardial tissue of patients with CHD [Citation52,Citation53].
Since oxidative stress is believed to be an integrated part of the surgical stress response during and after surgical procedures and cardiopulmonary bypass is known to mediate oxidative stress [Citation16,Citation23], studies measuring oxidative stress during and shortly after (cardiopulmonary bypass) surgery were excluded from this meta-analysis. An interesting review about oxidative stress blood markers after pediatric cardiac surgery for CHD is provided by Fudulu et al. [Citation54]. Various interventions to manipulate this oxidative stress (e.g. exogenous antioxidants, use of steroids, cardioplegia, blood prime strategies, use of different anesthetic agents or miniaturization of the cardiopulmonary bypass circuit) have been discussed in two papers [Citation15,Citation54]. However, up to now, it is unclear if modulation of the redox pathways can alter clinical outcomes [Citation54].
Regardless of the operative setting, no antioxidant treatments have yet been tested to reduce oxidative stress in patients suffering from CHD. In addition to CHD, oxidative stress is a component of many other (cardiovascular) diseases and occurs secondary to the initiation of pathology by other factors. Oxidative stress can disturb various signaling pathways and affect multiple biological processes and can thereby accelerate disease progression and exacerbate the symptoms [Citation55]. Therefore, the development of effective antioxidant therapies might be an important goal. Multiple antioxidant therapeutic strategies are being explored (e.g. N-acetylcysteine, vitamin C and vitamin E, …) in different clinical contexts, some of which are currently undergoing clinical trials [Citation55]. Interestingly, also many common drugs (e.g. statins) already widely used in a clinical setting, exert pleiotropic antioxidant effects. Nevertheless, although numerous small molecules evaluated as antioxidants have exhibited therapeutic potential in preclinical studies, clinical trial results have been disappointing [Citation51,Citation55]. This may be explained by the fact that the effectiveness of antioxidant therapies is limited by the extent to which oxidative stress plays a role in the pathology [Citation55]. Since oxidative stress is mostly a secondary contributor to disease, preventing oxidative stress may not have a major impact on disease progression, even when the antioxidants clearly increase antioxidant defense and decrease markers of oxidative stress [Citation55]. Thus, a greater understanding of the extent to which oxidative stress plays in the pathology of CHD and the mechanisms through which antioxidants act in CHD—and specifically in cCHD—may provide a rational approach that leads to greater pharmacological success [Citation55], which then needs to be extended to antioxidative treatments in specific clinical context.
Conclusions
This meta-analysis showed elevated oxidative stress levels in patients with CHD compared to healthy controls and more specifically in patients with cCHD in comparison with aCHD. Since it is known that children having cCHD also possess poorer clinical outcomes compared with those having aCHD, there could be potential in oxidative stress measurements as a new biomarker of disease severity. Identifying and developing new clinical blood biomarkers of the progression to HF is recommended, especially for the RV, given the limitations of clinical assessment and imaging modalities in determining the optimal timing for surgical intervention. Further research is warranted to clarify the exact role of oxidative stress and its contributors in CHD in order to get a better and more in-depth understanding of the underlying pathophysiology of CHD, especially the higher susceptibility of the RV to progress to HF. This could facilitate the development of dedicated antioxidant treatments and RV-specific HF therapies, which are necessary to improve survival in these patients and could be of particular importance in cCHD.
Disclosure statement
No potential conflict of interest was reported by the author(s).
Additional information
Funding
References
- Kovacs A, Lakatos B, Tokodi M, et al. Right ventricular mechanical pattern in health and disease: beyond longitudinal shortening. Heart Fail Rev. 2019;24(4):511–520. doi: 10.1007/s10741-019-09778-1.
- Canobbio MM. Health care issues facing adolescents with congenital heart disease. J Pediatr Nurs. 2001;16(5):363–370. doi: 10.1053/jpdn.2001.26570.
- Gomes-Neto M, Saquetto MB, da Silva e Silva CM, et al. Impact of exercise training in aerobic capacity and pulmonary function in children and adolescents after congenital heart disease surgery: a systematic review with meta-analysis. Pediatr Cardiol. 2016;37(2):217–224. doi: 10.1007/s00246-015-1270-x.
- Kempny A, Dimopoulos K, Uebing A, et al. Reference values for exercise limitations among adults with congenital heart disease. Relation to activities of daily life–single centre experience and review of published data. Eur Heart J. 2012;33(11):1386–1396. doi: 10.1093/eurheartj/ehr461.
- Diller GP, Dimopoulos K, Okonko D, et al. Exercise intolerance in adult congenital heart disease: comparative severity, correlates, and prognostic implication. Circulation. 2005;112(6):828–835. doi: 10.1161/CIRCULATIONAHA.104.529800.
- Baumgartner H, De Backer J, Babu-Narayan SV, et al. 2020 ESC guidelines for the management of adult congenital heart disease. Eur Heart J. 2021;42(6):563–645. doi: 10.1093/eurheartj/ehaa554.
- Giardini A, Specchia S, Berton E, et al. Strong and independent prognostic value of peak circulatory power in adults with congenital heart disease. Am Heart J. 2007;154(3):441–447. doi: 10.1016/j.ahj.2007.05.009.
- Verheugt CL, Uiterwaal CS, van der Velde ET, et al. Mortality in adult congenital heart disease. Eur Heart J. 2010;31(10):1220–1229. doi: 10.1093/eurheartj/ehq032.
- Warnes CA, Williams RG, Bashore TM, et al. ACC/AHA 2008 guidelines for the management of adults with congenital heart disease: executive summary: a report of the American College of Cardiology/American Heart Association Task force on practice guidelines (writing committee to develop guidelines for the management of adults with congenital heart disease). Circulation. 2008;118(23):2395–2451. doi: 10.1161/CIRCULATIONAHA.108.190811.
- Vanreusel I, Vermeulen D, Goovaerts I, et al. Circulating reactive oxygen species in adults with congenital heart disease. Antioxidants. 2022;11(12):2369. doi: 10.3390/antiox11122369.
- Marino P, de Oliveira Lopes G, Pereira Borges J, et al. Evaluation of systemic microvascular reactivity in adults with congenital heart disease. Congenit Heart Dis. 2018;13(6):978–987. doi: 10.1111/chd.12660.
- Zomer AC, Vaartjes I, Uiterwaal CS, et al. Circumstances of death in adult congenital heart disease. Int J Cardiol. 2012;154(2):168–172. doi: 10.1016/j.ijcard.2010.09.015.
- Romuk E, Jacheć W, Kozielska-Nowalany E, et al. Superoxide dismutase activity as a predictor of adverse outcomes in patients with nonischemic dilated cardiomyopathy. Cell Stress Chaperones. 2019;24(3):661–673. doi: 10.1007/s12192-019-00991-3.
- Ellis GR, Anderson RA, Lang D, et al. Neutrophil superoxide anion–generating capacity, endothelial function and oxidative stress in chronic heart failure: effects of short- and long-term vitamin C therapy. J Am Coll Cardiol. 2000;36(5):1474–1482. doi: 10.1016/s0735-1097(00)00916-5.
- Farias JG, Molina VM, Carrasco RA, et al. Antioxidant therapeutic strategies for cardiovascular conditions associated with oxidative stress. Nutrients. 2017;9(9):966. doi: 10.3390/nu9090966.
- Altin FH, Yildirim HA, Tanidir IC, et al. Alterations in antioxidant and oxidant status of children after on-pump surgery for cyanotic and acyanotic congenital heart diseases. Cardiol Young. 2017;27(2):325–332. doi: 10.1017/S1047951116000573.
- Ercan S, Cakmak A, Kösecik M, et al. The oxidative state of children with cyanotic and acyanotic congenital heart disease. Anadolu Kardiyol Derg. 2009;9(6):486–490.
- Reddy S, Bernstein D. Molecular mechanisms of right ventricular failure. Circulation. 2015;132(18):1734–1742. doi: 10.1161/CIRCULATIONAHA.114.012975.
- Si MS, Ohye RG. Stem cell therapy for the systemic right ventricle. Expert Rev Cardiovasc Ther. 2017;15(11):813–823. doi: 10.1080/14779072.2017.1379898.
- McGarry T, Biniecka M, Veale DJ, et al. Hypoxia, oxidative stress and inflammation. Free Radic Biol Med. 2018;125:15–24. doi: 10.1016/j.freeradbiomed.2018.03.042.
- Pirinccioglu AG, Alyan O, Kizil G, et al. Evaluation of oxidative stress in children with congenital heart defects. Pediatr Int. 2012;54(1):94–98. doi: 10.1111/j.1442-200X.2011.03478.x.
- Liberati A, Altman DG, Tetzlaff J, et al. The PRISMA statement for reporting systematic reviews and meta-analyses of studies that evaluate health care interventions: explanation and elaboration. J Clin Epidemiol. 2009;62(10):e1-34–34. doi: 10.1016/j.jclinepi.2009.06.006.
- Kücükakin B, Gögenur I, Reiter RJ, et al. Oxidative stress in relation to surgery: is there a role for the antioxidant melatonin? J Surg Res. 2009;152(2):338–347. doi: 10.1016/j.jss.2007.12.753.
- V G, S HY, Bhat BV, et al. Hypoxia induced DNA damage in children with isolated septal defect and septal defect with great vessel anomaly of heart. J Clin Diagn Res. 2014;8(4):Sc01–3.
- Vidya V. Estimation of DNA damage through comet assay in children with congenital heart disease-case-control study. Current Pediatric Research. 2014;18(1):1–4.
- Hozo SP, Djulbegovic B, Hozo I. Estimating the mean and variance from the median, range, and the size of a sample. BMC Med Res Methodol. 2005;5(1):13. doi: 10.1186/1471-2288-5-13.
- Wan X, Wang W, Liu J, et al. Estimating the sample mean and standard deviation from the sample size, median, range and/or interquartile range. BMC Med Res Methodol. 2014;14(1):135. doi: 10.1186/1471-2288-14-135.
- Perry C, Greig ID. Estimating the mean and variance of subjective distributions in pert and decision analysis. Manage Sci. 1975;21(12):1477–1480. doi: 10.1287/mnsc.21.12.1477.
- Manso PH, Carmona F, Dal-Pizzol F, et al. Oxidative stress markers are not associated with outcomes after pediatric heart surgery. Paediatr Anaesth. 2013;23(2):188–194. doi: 10.1111/pan.12040.
- Martinez-Quintana E, Pardo-Maiza J, Deniz-Alvarado B, et al. Gamma-glutamyl transferase and cardiovascular events in patients with congenital heart disease. Eur J Clin Invest. 2022;52(4):e13720. doi: 10.1111/eci.13720.
- Vidya V, Suma HY, Bhat B, et al. Comparison of DNA damage with the clinical manifestations of children with congenital heart diseases. Current Pediatric Research. 2015;19:13–16.
- National Heart LaBIN. Quality assessment tool for observational cohort and cross-sectional studies. 2013. https://www.nhlbi.nih.gov/health-topics/study-quality-assessment-tools
- Dervisoglu P, Oner T. Usefulness of ischemia-modified albumin for assessment of the effects of small ventricular septal defects on the pulmonary vascular bed. Cardiol Young. 2021;31(7):1207–1212. doi: 10.1017/S1047951121001116.
- Karataş Z, Baysal T, Sap F, et al. The role of tenascin-C and oxidative stress in rheumatic and congenital heart valve diseases: an observational study. Anadolu Kardiyol Derg. 2013;13(4):350–356. doi: 10.5152/akd.2013.102.
- Temel MT, Demiryurek S, Saracaloglu A, et al. Determination of dynamic thiol/disulphide homeostasis in children with tetralogy of fallot and ventricular septal defect. Cardiol Young. 2019;29(4):499–504. doi: 10.1017/S104795111900012X.
- Le GZ, Dong XY, Shen Y, et al. Erythrocyte oxidative stress in children with left to right shunt congenital heart disease. ]. Zhongguo Dang Dai Er Ke Za Zhi. 2010;12(6):440–443.
- Khalid S, Yamazaki H, Socorro M, et al. Reactive oxygen species (ROS) generation as an underlying mechanism of inorganic phosphate (P(i))-induced mineralization of osteogenic cells. Free Radic Biol Med. 2020;153:103–111. doi: 10.1016/j.freeradbiomed.2020.04.008.
- Ighodaro OM, Akinloye OA. First line defence antioxidants-superoxide dismutase (SOD), catalase (CAT) and glutathione peroxidase (GPX): their fundamental role in the entire antioxidant defence grid. Alexandria J Med. 2018;54(4):287–293. doi: 10.1016/j.ajme.2017.09.001.
- Sharifi-Rad M, Anil Kumar NV, Zucca P, et al. Lifestyle, oxidative stress, and antioxidants: back and forth in the pathophysiology of chronic diseases. Front Physiol. 2020;11:694. doi: 10.3389/fphys.2020.00694.
- Arlouskaya Y, Sawicka A, Głowala M, et al. Asymmetric dimethylarginine (ADMA) and symmetric dimethylarginine (SDMA) concentrations in patients with obesity and the risk of obstructive sleep apnea (OSA). J Clin Med. 2019;8(6).
- Sanli C, Oguz D, Olgunturk R, et al. Elevated homocysteine and asymmetric dimethyl arginine levels in pulmonary hypertension associated with congenital heart disease. Pediatr Cardiol. 2012;33(8):1323–1331. doi: 10.1007/s00246-012-0321-9.
- Campbell K, Vowinckel J, Keller MA, et al. Methionine metabolism alters oxidative stress resistance via the pentose phosphate pathway. Antioxid Redox Signal. 2016;24(10):543–547. doi: 10.1089/ars.2015.6516.
- Michel M, Dubowy KO, Entenmann A, et al. Targeted metabolomic analysis of serum amino acids in the adult fontan patient with a dominant left ventricle. Sci Rep. 2020;10(1):8930. doi: 10.1038/s41598-020-65852-x.
- Guerra A, Rego C, Coelho C, et al. Polymorphism C242T of the gene of the p22phox subunit for nicotinamide adenine dinucleotide phosphate oxidase, and erythrocytic antioxidant enzymes, in patients with tetralogy of fallot. Cardiol Young. 2007;17(3):295–300. doi: 10.1017/S1047951107000534.
- Pavlova E, Ra M, Vm S, et al. Oxidative stress in children undergoing surgical corrections of congenital heart malformations. Pediatric Oncall. 2009;6:53–54.
- Drapisz S, Goralczyk T, Jamka-Miszalski T, et al. Nonstenotic bicuspid aortic valve is associated with elevated plasma asymmetric dimethylarginine. J Cardiovasc Med. 2013;14(6):446–452. doi: 10.2459/JCM.0b013e3283588dfa.
- Pektaş A, Koca H, Pektas M, et al. The oxidative status of children with acyanotic congenital heart diseases: a randomized controlled study. Gazi Med J . 2016;
- Coskun FY, Taysı S, Kayıkçıoğlu M. Can serum 8-hydroxy-2'-deoxyguanosine levels reflect the severity of pulmonary arterial hypertension? Rev Assoc Med Bras. 2021;67(10):1437–1442. doi: 10.1590/1806-9282.20210640.
- Srujana K, Begum SS, Rao KN, et al. Application of the comet assay for assessment of oxidative DNA damage in circulating lymphocytes of tetralogy of fallot patients. Mutat Res. 2010;688(1–2):62–65. doi: 10.1016/j.mrfmmm.2010.03.009.
- Li RK, Mickle DA, Weisel RD, et al. Effect of oxygen tension on the anti-oxidant enzyme activities of tetralogy of fallot ventricular myocytes. J Mol Cell Cardiol. 1989;21(6):567–575. doi: 10.1016/0022-2828(89)90822-5.
- Vassalle C, Maltinti M, Sabatino L. Targeting oxidative stress for disease prevention and therapy: where do we stand, and where do we go from here. Molecules. 2020;25(11):2653. doi: 10.3390/molecules25112653.
- Takeuchi M, Takahashi T, Taga N, et al. Right ventricle of patients undergoing congenital cardiac surgery differentially expresses haem oxygenase-1 and heat shock protein 70 genes. J Int Med Res. 2003;31(5):413–417. doi: 10.1177/147323000303100509.
- Teoh KH, Mickle DA, Weisel RD, et al. Effect of oxygen tension and cardiovascular operations on the myocardial antioxidant enzyme activities in patients with tetralogy of fallot and aorta-coronary bypass. J Thorac Cardiovasc Surg. 1992;104(1):159–164. doi: 10.1016/S0022-5223(19)34848-2.
- Fudulu D, Angelini G. Oxidative stress after surgery on the immature heart. Oxid Med Cell Longev. 2016;2016:1971452–1971410. doi: 10.1155/2016/1971452.
- Forman HJ, Zhang H. Targeting oxidative stress in disease: promise and limitations of antioxidant therapy. Nat Rev Drug Discov. 2021;20(9):689–709. doi: 10.1038/s41573-021-00233-1.
- Tan Y, Wang S, Ren X, Zhang C, Xu F. The prognostic implications of perioperative endogenous hydrogen sulfide and nitric oxide levels in children with congenital heart disease complicated by pulmonary arterial hypertension. Eur J Pediatr. 2021;180(6):1915–1922.