Abstract
Biodegradable implantable matrices containing bovine serum albumin were prepared from oligoesters by melting, and subsequently tested on in vitro albumin release. The linear poly (DL-lactic acid) and the branched terpolymer of DL-lactic acid, glycolic acid, and mannitol were synthesized. Products were of similar molecular weight and possessed different thermal and swelling characteristics. Oligoesters were loaded with 4% albumin and plasticized by 30% triacetin. Other additives added into the matrices as albumin stabilizers were divalent stearates and magnesium oxide. The influences of oligomer molecules constitution, divalent ion stearates or magnesium oxide addition, and triacetin concentration on the albumin release were quantified. SDS-PAGE revealed protein hydrolysis during the dissolution tests.
Proteins play an indispensable role in all biological processes, functioning, for example, as enzymes, signal ligands, receptors, or highly specific and efficient antibodies. These macromolecules have been among the targets of genetic engineering research and focused on the production of potential proteins for therapeutic purposes (Putney Citation1998). A large number of protein drugs have thus become commercially available for clinical use. Protein-based drug use; however, has been limited due to short biological halftime and stability. Because the use of proteins is directed toward the treatment of chronical disorders, the therapy of which may take months or even years, the development of implantable systems with a prolonged release and capable of maintaining the required drug level in the organism over a long period of time is of particular importance. In this regard, a several month-long liberation at a constant rate following zero-order kinetics appears most advantageous (Weidner Citation2001). An alternative administration of aqueous solutions in the form of injections or infusions that would maintain the drug level in effective concentrations is difficult and costly and reduces patient compliance (Kang and Singh Citation2001).
Biodegradable polymer preparations, mainly in the form of injectable microparticles, are being developed especially with because of their ability to release therapeutically important proteins in a controlled fashion (Weert, Hennink, and Jiskoot Citation2000). As drug carriers, polymers have the capacity to protect proteins from proteolysis, prolong the period of their release, and hence increase their therapeutic efficacy. However, there is no “universal” biodegradable polymer on the market at the present time that would ensure an optimal microenvironment for all proteins (Jiang et al. Citation2002). As compared with low molecular weight drugs, macromolecular proteins possess a more complex multilayer structure and substantially lower stability (Diwan and Park Citation2001). Their molecules are easily apt to denaturate, aggregate (Castellanos et al. Citation2002; Kwon et al. Citation2001), and degradate by the action of moisture, of acidic components of the degrading polymer (Jiang and Schwendeman Citation2001; Youan et al. Citation2001), or of protein-polymer interactions (Kang and Schwendeman Citation2002). For these reasons, the possibilities of modulating protein release kinetics in relationship to the optimization of homogenization techniques or encapsulation techniques during production, composition, and properties of the carriers and also in relationship to the modifications of the incorporated protein are being investigated. To obtain trustworthy results that could serve in the prediction of in vivo behavior, the selection of the parameters of the medium for in vitro release evaluation also is important (Soriano, Llabres, and Evora Citation1995).
Thus, the preparation of the dosage form capable of a controlled long-term release without the loss of the biological activity of the protein is extremely complicated. With a number of proteins, a complete release from a polymer-based biodegradable preparation is practically impossible to achieve. The mechanism of protein release is generally directed by diffusion through the pores, polymer erosion, and osmotic phenomena (swelling), with the fundamental rule that the more water a polymer absorbs, the faster the protein release is. However, protein stability that is influenced by water content is another important factor, since water induces its degradation and is one of the main reasons for its incomplete liberation. Similarly, polymer microclimate also is modified by the water present (Kang and Schwendeman Citation2002).
Biodegradable copolymers of lactic and glycolic acids (PLGA) appear attractive carriers for the development of systems for prolonged and directed protein release (Singh et al. Citation2001). PLGAs are biocompatible, degrade to toxicologically acceptable acids (Fu et al. Citation1999), and are approved by the FDA for the formulation of systems with controlled drug release (Jain et al. Citation2000). Given many possibilities of the modification of their chemical or physical properties, wide flexibility is the main advantage of their use.
The goal of our work was to prepare biodegradable plasticized polymer matrices with albumin via melting an oligoester polymer carrier. Protein in high concentration can be built in these matrices; in fact, their protein encapsulation efficiency is, in principle, 100%, which is remarkably different from the amount of protein that can be microencapsulated into microparticle systems. Protein release from these matrices was tested with the aim of evaluating the possibilities of its stabilization during the process of prolonged release.
MATERIALS AND METHODS
Chemicals
Bovine serum albumin (BSA), fraction V, 99% (Sigma), triacetin 99% (Aldrich), magnesium oxide (Lachema a.s., Neratovice, Czech Rep.), zinc stearate and magnesium stearate (Aldrich), calcium stearate (Fluka), and Bradford reagent for protein determination (Sigma) were purchased. Polymers were prepared in our laboratory. All other chemicals were of pharmacopoeial or analytical grade.
Oligoester Preparation
Oligoesters were synthesized by the polycondenzation method described earlier (Dittrich and Melichar Citation1996). The parameters of the oligoesters are described in .
TABLE 1 Characteristics of oligoesters
Albumin Particle Size Reduction
Lyophilized albumin was comminuted in the mortar with the pestle in the presence of hexane. The size and shape of albumin particles were evaluated under a light microscope. Particles smaller than 50 μ m were obtained in this way.
Preparation of Biodegradable Matrices and In Vitro Release Study
The matrices with the protein were prepared via melting the polymer carrier. The required amount of an oligoester, triacetin (TA) as a plasticizer, and an additive (where applicable) were mixed at a temperature below 70°C. Subsequently, albumin was added to the mixture at a temperature below 50°C. The temperature setting was determined with a view to prevent heat-induced albumin degradation. The resultant mixture with albumin was then homogenized by mixing.
The matrices for the determination of albumin liberation were prepared by placing 150 mg samples of the homogeneous mixture to the bottom of scintillation vials and layering 7.0 g of a medium over the samples. A 1/15 mol.l-1 phosphate buffer solution (PBS) of pH 7.4 was chosen as the medium. Each matrix of equal composition was prepared in two or four parallel samples that were incubated at 37°C.
At given time intervals, the aqueous medium was completely withdrawn with a syringe, BSA was detected, and the same amount of fresh PBS (7.0 g) was again layered over the sample. The Bradford reagent, mixed in a 1:1 ratio with the medium withdrawn, was used for quantification of the amount of BSA released. Following the addition of the reagent to the medium sample, the intensity of blue coloration, proportional to the protein amount, was determined spectrophotometrically at 595 nm in the 10–60 min interval. Absorbance was measured in polystyrene cells against a blank sample, containing 1/15 mol.l-1 phosphate buffer of pH 7.4 and the Bradford reagent in 1:1 ratio (Bradford Citation1976).
Sodium Dodecyl Sulfate-Polyacrylamide Gel Electrophoresis (SDS-PAGE)
The presence of covalent aggregates and structural integrity of the in vitro released BSA was evaluated by the SDS-PAGE method (Igartua et al. Citation1998; Pokorová et al. Citation2002). The BSA released during the in vitro assays was compared with native BSA and reference markers as low molecular weight standards. The protein samples were diluted with a Tris-HCl buffer of pH 8.8 with 0.1% SDS, glycerol, and bromophenolic blue. Approximately 5 μ l of the BSA solution were loaded per gel lane. Electrophoresis of the samples was performed at a constant voltage of 100 V in a Tris/glycine/SDS buffer using a Bio-Rad Laboratories electrophoresis system for 1.5 hr. After migration, the gel was stained with silver to visualize the protein, destained, and dried.
RESULTS AND DISCUSSION
Matrices with BSA as a highly hydrophilic amphoteric macromolecular model substance were prepared. BSA was added into the mixture as the last component so that its structure could be modified by the preparation process and additives to a minimum degree; the compound was heated to a maximum temperature of 50°C. Upon exposure to heat, BSA undergoes two structural change stages, the first stage being reversible. Even though the second one is irreversible, it does not necessarily result in a complete destruction of the ordered structure. The first stage takes place on heating up to 65°C, and the second one commences with increasing the temperature above this point. The onset temperature of the conformation change was 58.1°C as found by differential scanning calorimetry, and the temperature of denaturation was 62°C (Friedli Citation1996).
For the carriers, a linear oligoester derived from D,L-lactic acid (PDLLA) and a branched oligoester based on D,L-lactic acid, glycolic acid, and mannitol (PMLGA) were selected. Oligoesters with branched chains are advantageous due to their continuous erosion, low degree of swelling, and low viscosity (Arvanitoyanis et al. Citation1995). As shown in , the differences in the Mw values determined for both carriers by the classic (GPC) and (GPC-MALS) can be attributed to their different branching.
A typical course of BSA release from the matrices formed from the linear and branched oligoester plasticized by 30% triacetin is shown in . According to GPC-MALS, both carriers had close Mw values. The influence of the PMLGA carrier branching on a faster BSA liberation is related to its higher hydrophilicity, since it contains terminal groups at a higher concentration.
FIG. 1 Albumin (BSA) release from biodegradable matrices—influence of oligoester constitution. Ingredients in weight percent: oligoester 60, BSA 4, triacetin 30, magnesium oxide 4, and zinc stearate 2. ♦ = PMLGA, ▴ = PDLLA.
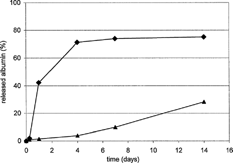
The extent of an nonpolar albumin sorption onto short hydrophilic branches is very limited. The BSA release also is related to the degree of matrix swelling. The swelling ratio values, expressed in percentage, and calculated as an increase in the weight of polymer corpuscules compared with that of dry polymer, are summarized in . This parameter has a quite different time evolution course for both types of the carriers. In the BSA release, an opening of the diffusion pathways in the matrix enabled by swelling at the beginning of the process apparently is the principal mechanism.
TABLE 2 Time evolution of swelling ratio values (in percent) of branched PMLGA and linear PDLLA oligoesters
Upon degradation of oligoester carriers, acids that lower the pH values in the matrices are formed. Stearates of bivalent cations react with these acids, which results in the formation of water soluble salts. The cations of these salts can influence carrier swelling by linking the terminal carboxylic groups of the carrier molecules with ionic bridges. The course of PMLGA swelling with 2% zinc stearate and without this additive is shown in . Apparently, PMLGA itself has relatively stable values of the swelling ratio, while they are gradually increased on the addition of 2% zinc stearate. A very hydrophilic network structure with an increased relaxation ability is probably formed. Since the zinc cations interact only with some carboxyls, microgel aggregates of molecules or a network structure with a low density of crosslinks seem a likely result. Following the plasticization of the oligoesters with 30% triacetin, similar relationships were observed. The plasticizer increases the swelling ratios and the use of zinc stearate, similar to a nonplasticized matrix, leads to a remarkable network-forming effect.
TABLE 3 Swelling ratio (in percent) time evolution of oligoester PMLGA, effect of 30% triacetin (TA) plasticization, and 2% zinc stearate (ZnSt) addition
In the matrices, the zinc cations can react with the BSA fraction in solution as well. Researchers have demonstrated that the formation of reversible, insoluble protein complexes with metal cations is an effective way toward increasing the stability of proteins (Putney Citation1998; Yamagata et al. Citation2003). Stearic acid, released upon the reaction of stearates with hydroxy acids in the matrix, has been described in a number of reports as a substance that binds to human serum albumin (Oncley Citation2003; Woolf Citation1998).
Calcium and magnesium stearates at 2% concentration also were incorporated into PMLGA matrices plasticized with 30% triacetine as an alternative to zinc cations. The influence of these additives was apparent from both the rate and extent of albumin liberation (). Almost all BSA was released upon the addition of the calcium salt, with the total liberation time at 4 days. Throughout the same time, albumin was being released from both the matrices with and without magnesium stearate. Hence, the magnesium salt had no influence on BSA liberation. In these matrices, a significant aggregation occurred, and approximately one-third of BSA was converted into buffer-insoluble products. The liberation over a longer period was achieved with the matrices containing zinc stearate as a consequence of a stronger interaction of the zinc ions (as compared with others) not only with the carrier, but also probably with the protein as well. The protein was being released into the medium for a period longer than 1 week. In this context, it is worth mentioning that modulation of the liberation of the recombinant human growth protein from the PLGA matrices by zinc oxide is based on the same principle (Tracy et al. Citation1999; Takada et al. Citation2003).
FIG. 2 Albumin (BSA) release from M-DLLA-GA oligoester matrices plasticized with triacetin—influence of divalent metal stearates. Ingredients in weight percent: BSA 4, triacetin 30, stearates 2, and oligoester M-DLLA-GA to 100. ♦ = Without stearates, – = zinc Stearate, ▴ = calcium stearate, ▪ = magnesium stearate.
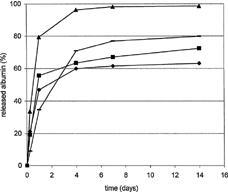
An increase in zinc stearate concentration from 2% to 8% also had an effect on albumin release (). A more pronounced crosslinking of the matrix structure by the zinc cations and, probably, its hydrophobicization by 8% hydrophobic salt as a precursor of a fatty acid lead to a lower amount of liberated albumin (). The process of zinc stearate incorporation, usually added to a plasticized carrier in the melt, was shown as an important factor. Alternatively, the salt was added to the melted carrier following its dissolution in triacetin. The change in the order of ingredient addition leads to a different reactivity of the stearate in the matrix and gives rise to a burst and consequently to release of the total amount of albumin.
FIG. 3 Albumin (BSA) release from M-DLLA-GA oligoester matrices plasticized with triacetin (TA). Influence of zinc stearate concentration and its incorporation mode. Ingredients in weight percent: BSA 4, TA 30, zinc stearate (ZnSt), and M-DLLA-GA to 100. ♦ = ZnSt 2 incorporated as powder, – = ZnSt 8 incorporated as powder, ▴ = ZnSt 2 incorporated as TA solution, ▪ = ZnSt 8 incorporated as TA solution.
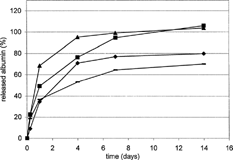
The influence of zinc stearate presence on the integrity of BSA released is depicted on . SDS-PAGE shows BSA released from the samples at different time intervals. While the protein maintains its structural integrity up to a 5-hr-incubation, BSA hydrolysis resulting in the generation of small molecular weight fragments is apparent after 3- and 5-day incubation periods. It is evident from the intensities of SDS-PAGE lines that a mixture of several degradation products in amounts increasing with time was released from the matrices. Whereas the existence of a fraction of molecular weight lower than 5000 was shown, it was not possible to analyze this fraction by the Bradford method. Hence, this low molecular weight fraction together with another one that arose from the aggregation (polymerization) of albumin molecules were the components of a protein amount that was not further specified. As the degradation of the carrier results in a higher concentration of terminal carboxylic groups, the pH level in the microclimate of the matrix drops down. The acidic pH initiates polymerization of albumin and consequently the amount of the protein that can be released from the matrices decreases (Lai and Topp Citation1999).
FIG. 4 SDS-PAGE of albumin (BSA) stability incorporated in matrices from oligoester PMLGA plasticized with triacetin (TA)—influence of zinc stearate addition. Lane 1 = LMW standard; lanes 2–4-PMLGA 66%; TA 30, BSA 4; lane 2, 5 hr; lane 3, 3 days; lane 4, 7 days; lanes 5–7, PMLGA 64, TA 30, ZnSt 2, BSA 4; lane 5, 5 hr, lane 6, 3 days; lane 7, 7 days.
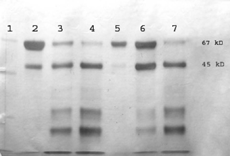
Magnesium oxide (MgO) used in our experiments ( and ) as well as magnesium hydroxide (Kang and Schwendeman Citation2002) are anacid additives, which increase both microclimate pH and polymer water uptake. MgO has been shown to prevent acid-induced instability of proteins, encapsulated in PMLGA and PDLLA matrices. There is ample evidence that a significant degradation of native albumin takes place before its dissolution. Magnesium oxide/hydroxide are additives, the use of which has been shown to increase pH values in the microclimate of the polymer matrices and retard hydrolysis of peptidic bonds as well as of the non-covalent aggregation of protein molecules (Zhu and Schwendeman Citation2000). The stabilizing effect of magnesium hydroxide and alkaline zinc carbonate on BSA stability in solid implants has been reported (Zhu and Schwendeman Citation2000). Matrix without MgO () displayed a fast initial burst in the range of 10–30% of BSA amount after 6 hr of liberation in all cases. On the other hand, matrices containing MgO ( and ) released a maximum of 7% of the total amount of BSA over the first 6 hr.
FIG. 5 Albumin (BSA) release from M-DLLA-GA oligoester matrices—influence of magnesium oxide and triacetin. Ingredients in weight percent: BSA 4, triacetin (TA) 30, magnesium oxide (MgO) 4, magnesium stearate 2, M-DL-LA-GA to 100. ♦ = TA 0%, MgO; = TA 10%, MgO; ▴ = TA 20%, MgO; ▪ = TA 30%, MgO.
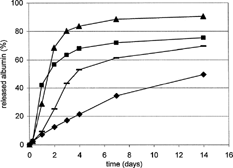
Matrix plasticization is advantageous because of the simple application of a dosage via an injection needle or in a spray. Thus, triacetin, which proved to be efficient before, was used as the plasticizer (Kladníčková, Klein, and Dittrich Citation2004). The influence of an increasing concentration of triacetin is depicted in .
As regards the nonplasticized matrix, about one-half of the protein content was released in a continuous fashion. Increasing triacetin concentration to 10% and 20% resulted in a gradual increase of liberation rate as well as the total amount of released albumin. An even higher concentration of the plasticizer (30%) led to a burst increase, but the total amount of the liberated model drug decreased. The most favorable liberation course was observed in the system containing 20% triacetin. Over 80% of the total amount of BSA was released over the first 4 days, followed by another, low-rate release phase. However, 30% triacetin concentration was used in most experiments, since this concentration coupled favorable liberation course with a suitable viscosity for easy preparation and application of the polymer matrices.
CONCLUSION
We have shown that the hydrophilization of the oligoester carrier by chain branching is advantageous from the viewpoint of its diffusivity for albumin. Plasticization with triacetin resulted in an acceleration of the model compound release from the oligoester matrices. Also the release of albumin from the matrices can be modified by the salts of stearic acid with polyvalent cations and magnesium oxide as additives.
We thank Prof. Štěpán Podzimek, Synpo a.s. Pardubice, for the polymer characterization by GPC and GPC-MALS. This work was supported by FRVŠ grant 2972/2003 and grant MSM 111600001.
REFERENCES
- Arvanitoyannis I., Nakayama A., Kawasaki N., Yamamoto N. Novel star-shaped polylactide with glycerol using stannous octoate or tetraphenyl tin as catalyst: 1. Synthesis, characterization, and study of their biodegradability. Polymer 1995; 36: 2947–2956, [CSA]
- Bradford M. M. A rapid and sensitive method for the quantitation of microgram quantities of protein utilizing the principle of protein-dye binding. Anal. Biochem. 1976; 72: 248–254, [PUBMED], [INFOTRIEVE], [CROSSREF], [CSA]
- Castellanos I. J., Cruz G., Crespo R., Griebenow K. Encapsulation-induced aggregation and loss in activity of γ-chymotrypsin and their prevention. J. Control Rel. 2002; 81: 307–319, [CROSSREF], [CSA]
- Dittrich M., Melichar L. Influence of processing conditions on the release of sodium fluoride from oligomeric L-lactic acid matrices. Biomaterials 1996; 17: 1591–1597, [PUBMED], [INFOTRIEVE], [CROSSREF], [CSA]
- Diwan M., Park T. G. Pegylation enhances protein stability during encapsulation in PLGA microspheres. J. Control Rel. 2001; 73: 233–244, [CROSSREF], [CSA]
- Friedli G.-L. Interaction of deamidated soluble wheat protein (SWP) with other food proteins and metals. PhD. Thesis, University of Surrey, , UK. 1996
- Fu K., Griebenow K., Hsieh L., Klibanov A. M., Langer R. FTIR characterization of the secondary structure of proteins encapsulated within PLGA microspheres. J. Control Rel. 1999; 58: 357–366, [CROSSREF], [CSA]
- Igartua M., Hernández R. M., Esquisabel A., Gascón A. R., Calvo M. B., Pedraz J. L. Stability of BSA encapsulated into PLGA microspheres using PAGE and capillary electrophoresis. Int. J. Pharm. 1998; 169: 45–54, [CROSSREF], [CSA]
- Jain R. A., Rhodes Ch. T., Railkar A. M., Malick A. W., Shah N. H. Controlled release of drugs from injectable in situ formed biodegradable PLGA microspheres: effect of various formulation variables. Eur. J. Pharm. Biopharm. 2000; 50: 257–262, [PUBMED], [INFOTRIEVE], [CROSSREF], [CSA]
- Jiang W., Schwendeman S. P. Stabilization and controlled release of bovine serum albumin encapsulated in poly(D,L-lactide) and poly(ethylene glycol) microsphere blends. Pharm. Res. 2001; 18: 878–885, [PUBMED], [INFOTRIEVE], [CROSSREF], [CSA]
- Jiang G., Woo B. H., Kang F., Singh J., DeLuca P. P. Assessment of protein release kinetics, stability and protein polymer interaction of lysozyme encapsulated poly(D,L-lactide-co-glycolide) microspheres. J. Control Rel. 2002; 79: 137–145, [CROSSREF], [CSA]
- Kang F., Singh J. Effect of additives on the release of a model protein from PLGA microspheres. AAPS Pharm. Sci. Tech 2001; 2(4), article 30[CSA]
- Kang J., Schwendeman S. P. Comparison of the effects of Mg(OH)2 and sucrose on the stability of bovine serum albumin encapsulated in injectable poly(D,L-lactide-co-glycolide) implants. Biomaterials 2002; 23: 239–245, [PUBMED], [INFOTRIEVE], [CROSSREF], [CSA]
- Kladníčková I., Klein T., Dittrich M. Effect of plasticizers of the triester glycerol type on the release of albumin from biodegradable polymer matrices. Ces. Slov. Farmacie 2004; 1: 27–30, [CSA]
- Kwon Y. M., Baudys M., Knutson K., Kim S. W. In situ study of insulin aggregation induced by water-organic solvent interface. Pharm. Res. 2001; 18: 1754–1759, [PUBMED], [INFOTRIEVE], [CROSSREF], [CSA]
- Lai M. C., Topp E. M. Solid-state chemical stability of proteins and peptides. J. Pharm. Sci. 1999; 88: 489–500, [PUBMED], [INFOTRIEVE], [CROSSREF], [CSA]
- Oncley J. L. Dielectric behavior and atomic structure of serum albumin. Biophys. Chem. 2003; 100: 151–158, [PUBMED], [INFOTRIEVE], [CROSSREF], [CSA]
- Pokorová D., Reschová S., Franz J., Hampl J., Dittrich M. Antigenic and adjuvant activities of branched aliphatic oligoester (M-DL-LA) microspheres with incorporated bovine rotavirus. Drug Del. 2002; 9: 105–111, [CROSSREF], [CSA]
- Putney S. D. Encapsulation of proteins for improved delivery. Curr. Opin. Chem. Biol. 1998; 2: 548–552, [PUBMED], [INFOTRIEVE], [CROSSREF], [CSA]
- Singh M., Shirley B., Bajwa K., Samara E., Hora M, O'Hagan D. Controlled release of recombinant insulin-like growth factor from a novel formulation of polylactide-co glycolide microparticles. J. Control Rel. 2001; 70: 21–28, [CROSSREF], [CSA]
- Soriano I., Llabres M., Evora C. Release control of albumin from polylactic acid microspheres. Int. J. Pharm. 1995; 125: 223–230, [CROSSREF], [CSA]
- Takada S., Yamagata Y., Misaki M., Taira K., Kurokawa T. Sustained release of human growth hormone from microcapsules prepared by a solvent evaporation technique. J. Control Rel. 2003; 88: 229–242, [CROSSREF], [CSA]
- Tracy M. A., Ward K. L., Firouzabadian L., Wang Y., Dong N., Qian R., Zhang Y. Factors affecting the degradation rate of poly(lactide-co-glycolide) microspheres in vivo and in vitro. Biomaterials 1999; 20: 1057–1062, [PUBMED], [INFOTRIEVE], [CROSSREF], [CSA]
- Weert M., Hennink W. E., Jiskoot W. Protein instability in poly(lactic-co-glycolic) acid microparticles. Pharm. Res. 2000; 17: 1159–1167, [PUBMED], [INFOTRIEVE], [CROSSREF], [CSA]
- Weidner J. A new drug delivery system for protein drugs using silicone. Drug Delivery 2001; 6: 1129–1130, [CSA]
- Woolf T. B. Simulations of fatty acid-binding proteins suggest sites important for function. I. Stearin acid. Biophysical J 1998; 74: 681–693, [CSA]
- Yamagata Y., Misaki M., Kurokawa T., Taira K., Takada S. Preparation of a copoly (dl-lactic/glycolic acid)-zinc oxide complex and its utilization to microcapsules containing recombinant human growth hormone. Int. J. Pharm. 2003; 251: 133–141, [PUBMED], [INFOTRIEVE], [CROSSREF], [CSA]
- Youan B. B. C., Jackson T. L., Dickens L., Hernandez C., Owusu-Ababio G. Protein release profiles and morphology of biodegradable microcapsules containing an oily core. J. Control Rel. 2001; 76: 313–326, [CROSSREF], [CSA]
- Zhu G., Schwendeman S. P. Stabilization of proteins encapsulated in cylindrical poly(lactide-co-glycolide) implants: mechanism of stabilization by basic additives. Pharm. Res 2000; 17: 351–357, [PUBMED], [INFOTRIEVE], [CROSSREF], [CSA]