Abstract
Fluoroquinolones are broad-spectrum antimicrobial agents that seem to reach their intracellular target site (DNA gyrase) in Escherichia coli by means of an uptake process through the outer and inner membranes. Delivery of quinolones with liposomes has many advantages than the free form of the drug. Liposomes may represent an excellent device for improving the selective transport of antibiotics in these respects. In this study, enrofloxacin-loaded multilamellar vesicles (MLVs) were prepared and the effects of formulation variables on the liposome characteristics were investigated. Liposomes were prepared by using the dry lipid film method. A number of variables, such as phospholipid (DL-α -phosphatidylcholine dipalmitoyl), cholesterol, enrofloxacin (ENF), stearylamine, and dicetyl phosphate molar ratios and α -tocopherol amounts, were studied. The liposome size, encapsulation capacity, drug release, stability, and electrophoretic mobility of ENF-loaded liposomes were determined. Using this method, spherical MLVs with high drug content could be produced. Particle size of liposomes changed between 1.63 and 3.31 μ m and liposome size was affected by all formulation variables (p < 0.05) except molar ratio of ENF. MLVs can be used as a carrier system for the controlled release of ENF. The highest encapsulation of ENF amount can be obtained using positively charged SA in the formulation and changing the formulation parameters can vary drug release patterns.
Enrofloxacin (ENF) is a member of fluoroquinolone antibiotics marketed specifically for use in veterinary medicine. It has a strong bactericidal activity against a broad spectrum of aerobic and some facultative anaerobic bacteria. Fluoroquinolone effectively penetrates into the bacterial cells but the whole content of the drug rarely re-uptakes from the cells. Therefore ENF does not concentrate effectively in the cell pathogens and this problem affects the therapeutic efficiency of free ENF. It has been pointed out that encapsulations of fluoroquinolones in liposomes could improve their effectiveness in cells (Montero et al. Citation1994; Puglisi et al. Citation1995; Cabanes et al. Citation1995; Lizondo et al. Citation1997; Baş et al. Citation2000). However, there is limited information on liposomal form of ENF (Cabanes et al. Citation1995; Baş et al. Citation2000, Citation2002; Sezer et al. Citation2002, Citation2004).
Liposomes as drug delivery systems have the potential to provide controlled release of the administered drug and to increase the stability of the labile drugs such as antibiotics (Lian and Ho Citation2001). Encapsulation of antibiotics in liposomes has a number of advantages (New Citation1995). Following different preparation methodologies and liposomes types such as large unilamellar vesicles (LUVs) and multilamellar vesicles (MLVs) show different encapsulation capacity, stability, and release properties when encapsulated antibiotics and other drugs into these vesicles (Maurer et al. Citation1998; Grabielle-Madelmont, Lesieur, and Ollivon et al. Citation2003; Castelli, Raudino, and Fresta et al. Citation2005). The size and number of lamellae of the vesicles also are important for the encapsulation of drugs in liposomes. Because MLVs contain large lipid surface and number of lamellae, they are more suitable than the other types of liposomes for the encapsulation of hydrophobic antibiotics such as ENF via interacting with bilayer and the lipid layers. For these reasons high encapsulation capacity of hydrophobic antibiotics into liposomes can be obtained through MLVs (Bedrad and Bryan Citation1989; New Citation1995; Maurer et al. Citation1998). In the previous studies to provide the cell uptake and minimize the drug escapes from cell, LUVs were prepared and evaluated for the liposome characteristics (Baş et al. Citation2000, Citation2002; Sezer et al. Citation2002, Citation2004).
The aim of our study was to prepare enrofloxacin-loaded MLVs and determine the influence of liposomal composition on in vitro release characteristics, physical stability, and encapsulation of ENF into MLVs. The effects of formulation variables on the liposome characteristics were investigated to develop an optimal formulation.
MATERIALS AND METHODS
DL-α -phosphatidylcholine dipalmitoyl (DPPC), stearylamine (SA), dicetyl phosphate (DCP), and α -tocopherol were purchased from Sigma Chemical Co. (USA). Cholesterol (96.0%) was from Merck Chemical Co. (Darmstadt, Germany) and ENF (purity 99.7%) was obtained from Bayer (Germany). All other chemicals were of analytical grade.
Preparation of Liposomes
MLVs were prepared using modified dry film method (Vays, Goswami and Singh Citation1995). DPPC, cholesterol, and ENF with or without SA or DCP were dissolved in chloroform in a 50 ml round-bottom flask and dried in a rotary evaporator (Heidolph WB 2000, Germany) under reduced pressure at 50°C from a thin lipid film on the flask. A jet of dry nitrogen removed traces of chloroform and the film formed was hydrated with phosphate buffered saline (PBS) (pH 7.4). The flask was shaken during hydration with the help of a wrist-action shaker (Janke & Kunkel type VX 10, Germany) and multilamellar liposomes were formed by constant vortexing (IKA, KMS1, USA). A number of variables were investigated for the optimization of liposome properties () (n=3).
TABLE 1 Formulations, codes, size, drug encapsulation capacity, and zeta potential values of MLVs
Purification of Liposomes
Free and liposomal ENF were separated by gel filtration on Sephadex G-50 column at 25°C (1.0 cm diameter; a gel bed height of 20 cm, Sigma Chemical Co., USA) and PBS buffer (pH 7.4) was used as medium (Pons, Foradada, and Esteirich Citation1993).
Encapsulation Efficiency
First, 0.1 mL (%1 v/v) Triton X-100 solution was added to the 1 mL of freshly prepared liposomal suspension and vortexing. Then 0.1 ml sample was taken and adjusted to 10 mL with PBS (pH 7.4). The amount of drug present in this solution was estimated spectrophotometrically at 270 nm (BioSpec, Shimadzu, Japan) (n=3).
Determination of Liposome Size
Measurements were performed at 25°C, using a Helos submicron particle-size analyzer (Sympatec GmbH, Clausthal-Zellerfeld, Germany) with a suspension closed-loop cell (type Sucell 12CL) and Helos and QX software. The dilution medium was bidistilled water.
Zeta Potential
The vesicles were suspended in fresh PBS (pH 7.4) as dilution medium (20 sec at 25°C) and the zeta potential values determined with Zetasizer 3000 HSA Malvern Ltd. (Malvern, UK) (n=3).
In Vitro Dissolution Study
In vitro drug release from liposomal systems was determined using dialysis sacks (The sacks retain proteins with molecular weight greater than 12.000, Sigma, 250-7U USA). The dialysis sack was washed and one end of the sack was tied and 5 mL of liposomal suspension filled into the sack and the other end of the sack was tied. The dialysis sacks containing liposomal preparations were suspended vertically into a beaker containing 200 mL of PBS (pH 7.4) and contents of the beaker were stirred using a magnetic stirrer at 37 ± 1°C. Then, samples were withdrawn periodically and replaced with fresh PBS and the amount of drug was determined spectrophotometrically at 270 nm (n=3).
Stability Study
ENF-encapsulated MLVs were stored at 4 ± 1°C for 60 days and drug loss was monitored. Then 0.5 mL of samples were taken on the 15th, 30th, and 60th days of the study and Sephadex G-50 column was applied. All the fractions were pooled after 0.1 mL of a 1% v/v aqueous solution of Triton X-100 was added to the suspension, and the volume was adjusted. The amount of drug was determined spectrophotometrically as mentioned above (n=3).
Statistical Analysis
Statistical data analysis was performed using the one-way analysis of variance with p < 0.05 as minimum level of significance.
RESULTS AND DISCUSSION
Characterization of ENF Liposomes
Dry lipid film method was used for the encapsulation of ENF in MLV liposomes (Vays et al. Citation1995). ENF-loaded MLV liposomes had higher drug encapsulation as seen in . Encapsulation capacity of liposomes changed between 51.0% and 96.7%. The DPPC, cholesterol, ENF, SA, and DCP molar ratio in the formulation affected the drug encapsulation capacity of the MLVs (p<0.05). The highest drug encapsulation capacity (96.7%) was obtained with the liposomes containing the highest molar ratio of SA (E3). The reason for the high drug encapsulation in MLVs may be the interaction between the electrostatic forces of SA and ENF (Kulkarni, Betageri, and Singh Citation1995). As summarized in , the percentage of drug encapsulation into MLVs increased with the increase in SA content of the formulation (E1-E3) (p<0.05). On the contrary, using the increasing amount of DCP decreased the encapsulation capacity (F1-F3) (p<0.05). The addition of even the smallest amount of DCP into the formulation was enough to significantly reduce the encapsulation capacity of the drug.
The encapsulation capacity of ENF increased with increase in DPPC, cholesterol, and drug molar ratios in MLV formulations (p<0.05). Increasing the molar ratio of DDPC in liposomes increased the drug encapsulation capacity of MLVs. Because the thickness of the lipid surface of liposome changed with the amount of phospholipid in the MLV formulations, higher encapsulation capacity of drugs in liposome was obtained with increases in the lipid surface and bilayer of MLVs (Montero, Carrera, and Hernandez Citation1994; Kulkarni et al. Citation1995; Castelli et al. Citation1999; Belsito et al. Citation2005). Because of the large lipid surface of MLVs, quinolone solubilization within the phospholipid bilayer of liposomes increased and this could lead to high drug encapsulation capacity into the vesicles (Montero et al. Citation1994; New Citation1995).
The other factor affecting the encapsulation capacity of liposome was the cholesterol content of vesicles. Cholesterol was added to the liposome formulation because it had three recognized effects: increasing the fluidity or microviscosity of the bilayer; reducing the permeability of the membrane; and stabilizing the membrane in the presence of biological fluids (Plessis et al. Citation1996). The MLVs formulation containing the highest cholesterol content encapsulated 93.1% of drug (B3) and this value was higher than the LUVs formulations previously reported (Sezer et al. Citation2002, Citation2004). The encapsulation capacity of this formulation increased with the cholesterol amount of MLVs. It might be because of the higher encapsulation capacity of ENF in MLVs than in LUVs, more forming cholesterol into the MLVs lamellarity, and reduced permeability of the membranes of the vesicle. Changing the amount of α -tocopherol (D1 and D2) did not affect the encapsulation capacity of liposome. Therefore the data were given in (p>0.05).
Zeta potential values of MLVs are given in . Zeta potential of liposome changed between −91.3 and +58.8 mV. The effects of molar ratios of ENF, SA, and DCP on liposome encapsulation led to electrophoretic mobility. At pH < 7.0, fluoroquinolones are positively charged due to the C-7 position of the piperazinyl group and as a result, they become relatively soluble (Montero et al. Citation1994). Above this pH, ENF is negatively charged and shows hydrophilic properties. Nearly all quinolones show hydrophobic properties that depend on structure and pH (Lizondo et al. Citation1997). ENF has encapsulated negative charge in MLVs suspension (pH∼ 7.5), and the addition of DCP (negatively charged) into liposome formulation increased this negativity and decreased the encapsulation capacity to 57.9% as seen in . Adding the SA (positively charged) in MLVs, liposomes loaded positive charge, and we saw that the addition of SA into the liposome encapsulation capacity was increased to 96.7% (E3) () (p<0.05). This difference might be attributed to interlamellar repulsion and ion pair formation between the ENF and the charged components (Bedrad and Bryan Citation1989; New Citation1995; Lizondo et al. Citation1997).
FIG. 2 ENF-loaded MLVs vesicle size distribution of E3 formulation (the molar ratios of DPPC: cholesterol: ENF: SA is 7: 26: 1.5: 3).
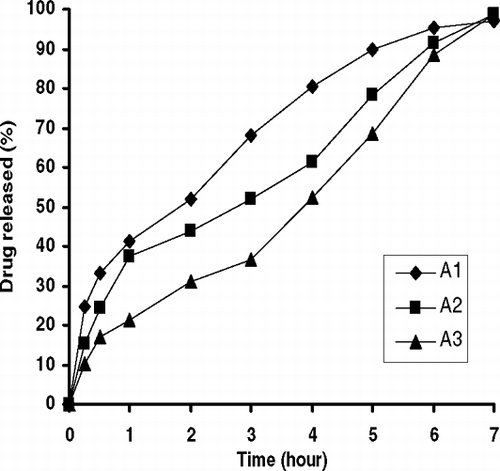
Incorporation of SA into the liposomes increased the zeta value of liposome from +12.0 to +58.8 mV (p<0.05) and average liposome size from 1.63 to 2.67 μ m (). In contrast, the addition of DCP reduced the electrophoretic mobility of MLVs from −51.3 to −91.3 mV (p<0.05). Except the charge value of the formulations containing SA or DCP and lower molar ratios of ENF, the zeta potential of MLVs did not affect the liposome size (p>0.05). As seen in , the size of the vesicles changed between 1.63 and 3.31 μ m. The DPPC, cholesterol, SA, and DCP molar ratios in the formulation affected the liposome size (p<0.05). We observed that the size distribution of all vesicles is sharp and the best homogeny pellet size was obtained by formulation E3 ().
Release of ENF from MLV Liposome
Release behaviors of ENF-loaded MLVs are given in , , , , . As seen in , the release of ENF from MLVs changed with phospholipid amount (A1–A3). Drug release delayed as molar ratio of DPPC increased in the formulation (p<0.05). This might be due to the interaction of ENF with the large surface of lipid bilayer membrane of MLVs and DPPC lipid layer thickness and fluidity of membrane as reported previously (Kulkarni et al. Citation1995; Castelli et al. Citation1999; Belsito et al. Citation2005). shows the effect of molar ratio of cholesterol on drug release from liposomes. The release of ENF from MLVs was not completed during the test and the amount of released drug decreased from 92.0% to 66.8% with the increase of cholesterol content in the liposomes (B1–B3) (p<0.05). ENF release from B3 formulation, which contained the highest molar ratio of cholesterol, was the slowest among all other formulations. High membrane permeability and the stabilizing effect of cholesterol on membrane structure may be the reason of as reported previously (Puglisi et al. Citation1995; Kulkarni et al. Citation1995; Mohammeda et al. Citation2004; Sezer et al. Citation2004). Similar results were obtained with an increase of molar ratio of ENF in the formulation () (p<0.05).
FIG. 3 Effect of lipid compositions (cholesterol) [the molar ratios of DPPC: cholesterol: ENF are 4.7: 8.7: 1 (B1), 4.7: 13: 1 (B2), and 4.7: 17.3: 1 (B3)] on drug release from MLVs.
![FIG. 3 Effect of lipid compositions (cholesterol) [the molar ratios of DPPC: cholesterol: ENF are 4.7: 8.7: 1 (B1), 4.7: 13: 1 (B2), and 4.7: 17.3: 1 (B3)] on drug release from MLVs.](/cms/asset/0b54fc88-c089-49ff-9eb9-31b26b762e7b/idrd_a_163993_uf0003_b.gif)
FIG. 5 Effect of SA molar ratios [the molar ratios of DPPC: cholesterol: ENF: SA are 7: 26: 1.5: 1 (E1), 7: 26: 1.5: 2 (E2), and 7: 26: 1.5: 3 (E3)] on drug release from MLVs.
![FIG. 5 Effect of SA molar ratios [the molar ratios of DPPC: cholesterol: ENF: SA are 7: 26: 1.5: 1 (E1), 7: 26: 1.5: 2 (E2), and 7: 26: 1.5: 3 (E3)] on drug release from MLVs.](/cms/asset/186ac3e2-68b6-474d-a0af-02bb1d24d178/idrd_a_163993_uf0005_b.gif)
FIG. 6 Effect of DCP molar ratios [the molar ratios of DPPC: cholesterol: ENF: DCP are 7: 26: 1.5: 1 (F1), 7: 26: 1.5: 2 (F2), and 7: 26: 1.5: 3 (F3)] on drug release from MLVs.
![FIG. 6 Effect of DCP molar ratios [the molar ratios of DPPC: cholesterol: ENF: DCP are 7: 26: 1.5: 1 (F1), 7: 26: 1.5: 2 (F2), and 7: 26: 1.5: 3 (F3)] on drug release from MLVs.](/cms/asset/00b6dea6-ba60-4d1f-94cd-bb0623004d49/idrd_a_163993_uf0006_b.gif)
As seen in , when electropositive detergent SA was added into the formulation, the electropositive charge of the MLVs increased. We observed that the drug release was slower than the negatively charge liposome with the addition of SA into the liposomal formulation (). On the other hand, there was an ionic binding between negatively charged phosphate groups of the phospholipid and the positively charged piperazine ring at the C-7 position of the ENF as reported (Montero et al. Citation1994; Puglisi et al. Citation1995; Lizondo et al. Citation1997). In contrast, the fastest release was obtained with the addition of DCP into the formulation (). The drug release from MLVs increased with increasing DCP ratios in the MLVs formulation () (p<0.05). Because the effect of α -tocopherol amounts on release of ENF from the liposomes was statistically insignificant, the figure is not given (p>0.05). The data on the release kinetics (r2) of MLVs are given in . As seen in the table, ENF release from the liposomes fit to zero-order kinetic model except A1, C1, F2, and F3 formulations.
TABLE 2 Dissolution kinetic values (r2) of MLVs
Stability Study
All formulations were stored at +4°C in PBS (pH 7.4) at 60 days and subjected to drug retention as shown in . Except for A1, A2, C1, F1, F2, and F3 formulations, all series remained stable for 60 days after production (p<0.05).
TABLE 3 Drug content of MLVs during storage time
Increasing ratios of DPPC, cholesterol, ENF, and SA had a significant effect on the drug relevtion of the MLVs () (p<0.05) for 60 days, but an increase in DCP concentration sharply reduced the drug retention of liposomes () (p<0.05). We did not see any aggregation in all formulations with microscopic observation. Increased drug retention of MLVs with increased DPPC, cholesterol, ENF, and SA content in the formulation might be due to the increased thickness of lipid bilayers, charge of MLVs, and the reduced permeability of the membrane surface with cholesterol ratio in the formulations (Lian and Ho Citation2001). The nature and density of charge on the surface of the liposomes influenced stability because ENF was positively charged at pH < 7.0 in solutions (Montero et al. Citation1994; Lizondo et al. Citation1997). When ENF was encapsulated in MLVs, the medium pH was above the pH 7.0 and ENF showed negative character.
The high loss of drug from the H formulations might be one of the causes of the higher molar ratio of the DCP (negatively charged) in liposome formulations () (p<0.05). Another important point was the effect of cholesterol ratio on MLVs stability. A fraction of cholesterol is often included in the lipid formulation to modulate the rigidity, to reduce serum-induced instability, and to increase the storage half-life of formulation (Aoki et al. Citation1995; Lian and Ho Citation2001; Mohammeda et al. Citation2004).
In general, we observed higher stability from MLVs compared with LUVs or another type of liposomes of the same lipid composition. This could be explained by the higher energy content of MLVs with the higher surface area of the water/lipid interface of the structure (Lang and Vigo-Pelfrey Citation1993).
CONCLUSION
ENF was successfully encapsulated into the MLVs by changing some formulation parameters: the molar ratios of DPPC, cholesterol, ENF, and charge components; SA and DCP in the liposome formulation have importance for the drug encapsulation, vesicle size, electrophoretic mobility, and drug release from MLVs. The highest encapsulation capacity and the slowest ENF release were obtained with the formulation E3 containing the highest amount of SA. On the other hand, B and E formulations remained stable during the 60-day storage period. From this point of view, E3 formulation is the optimum formulation for the encapsulation of ENF into the MLVs.
ACKNOWLEDGMENTS
The authors are grateful to Bio. Zeynep Bütünley (Roche) for measuring the vesicle sizes and Pharm. Aslı han Akkar for her collaboration in the charge determination of MLVs.
REFERENCES
- Aoki H., Sun C., Fuji K., Miyajima K. Disposition kinetics of liposomes modified with synthetic aminoglycolipids in rats. Int. J. Pharm. 1995; 15: 183–191
- Baş A. L., Elmas M., Şimşek A., et al. Efficacies of free and liposome-encapsulated enrofloxacin (Baytril®) against Staphylococcus aureus infection in Turkish shepherd dog neutrophils in vitro. Rev. Med. Vet. 2000; 151: 415–420
- Baş A. L., Şimşek A., Çorlu M., et al. Determination of intracellular concentration of free and two types of liposomes-encapsulated enrofloxacin in anatolian shepherd dog monocytes. J. Vet. Med. B. 2002; 49: 1–5
- Bedrad J., Bryan L. E. Interaction of the fluroquinolone antimicrobial agents ciprofloxacin and enoxacin with liposomes. Antimicrob. Agents Ch. 1989; 33: 1379–1382
- Belsito S., Bartucci R., Sportelli L. Paclitaxel interaction with phospholipid bilayers: high-sensitivity differential scanning calorimetric study. Thermochim. Acta 2005; 427: 175–180
- Cabanes A., Reig F., Anton J. M. G., Arboix M. Sustained release of liposome-encapsulated enrofloxacin after intramuscular administration in rabbits. Am. J. Vet. Res. 1995; 56: 1498–1501
- Castelli F., Caruso S., Giuffrida N. Different effects of two structurally similar carotenoids, lutein and b-carotene, on the thermotropic behaviour of phosphatidylcholine liposomes. Calorimetric evidence of their hindered transport through biomembranes. Thermochim. Acta 1999; 327: 125–131
- Castelli F., Raudino A., Fresta M. A mechanistic study of the permeation kinetics through biomembrane models: Gemcitabine–phospholipid bilayer interaction. J. Colloid. Interf. Sci. 2005; 285: 110–117
- Grabielle-Madelmont C., Lesieur S., Ollivon M. Characterization of loaded liposomes by size exclusion chromatography. J. Biochem. Biophys. Meth. 2003; 56: 189–217
- Kulkarni S. B., Betageri G. V., Singh M. Factor affecting microencapsulation of drug in liposomes. J. Microencapsul. 1995; 12: 229–246
- Lang J. K., Vigo-Pelfrey C. Quality control of liposomal lipids with special emphasis on peroxidation of phospholipids and cholesterol. Chem. Phys. Lipids. 1993; 64: 19–29
- Lian T., Ho R. J. Y. Trends and developments in liposome drug delivery systems. J. Pharm. Sci. 2001; 90: 667–680
- Lizondo M., Pons M., Gallardo M., Estelrich J. Physicochemical properties of enrofloxacin. J. Pharm. Biomed. Anal. 1997; 15: 1845–1849
- Maurer N., Wong K. F., Hope M. J., Cullis P. R. Anomalous solubility behavior of the antibiotic ciprofloxacin encapsulated in liposomes: a 1H-NMR study. Biochim. Biophys. Acta 1998; 1374: 9–20
- Montero M. T., Carrera I., Hernandez-Borrell J. Encapsulation of a quinolone in liposomes. Location and effect on lipid bilayers. J. Microencapsul 1994; 11: 423–430
- Mohammeda A. R., Westonb N., Coombesa A. G. A., et al. Liposome formulation of poorly water soluble drugs: optimisation of drug loading and ESEM analysis of stability. Int. J. Pharm. 2004; 285: 23–34
- New R. R. C. Influence of liposome characteristics on their properties and fate. Liposome as Tools in Basic Research and Industry, J. R. Philippot, F. Schuber. CRC Press, Florida 1995; 4–20
- Plessis J., Ramachandran C., Weiner N., Müller D. G. The influence of lipid composition and lamellarity of liposomes on the physical stability of liposomes upon storage. Int. J. Pharm. 1996; 127: 273–278
- Pons M., Foradada M., Esteirich J. Liposomes obtained by the ethanol injection method. Int. J. Pharm. 1993; 95: 51–56
- Puglisi G., Fresta M., Mazzone G., et al. Formulation parameters of fluoroquinolone-loaded liposomes and in vitro antimicrobial activity. Int. J. Pharm. 1995; 118: 65–76
- Sezer A. D., Baş A. L., Akbuğa J. Effect of formulation variables on the characteristics of enrofloxacin liposomes. 62nd International World Congress of FIP, NiceFrance, August–5 September, 31, 2002; 28
- Sezer A. D., Baş A. L., Akbuğa J. Encapsulation of enrofloxacin in liposomes I: in vito characterization of LUV. J. Liposome Res. 2004; 14(1–2)77–86
- Vays S. P., Goswami S. K., Singh R. Liposomes based nasal delivery system of nifedipine: development and characterization. Int. J. Pharm. 1995; 118: 23–30