Abstract
The effects of pressure-sensitive adhesives and vehicles on the in vitro permeation of ketorolac and in vivo pharmacokinetics were studied. Duro-Tak 87-2196® showed the highest in vitro permeation profiles, and propylene glycol monolaurate-diethylene glycol monoethyl ether (DGME) (60:40, v/v) and propylene glycol monocaprylate-DGME (60:40, v/v) revealed the most favorable in vitro and in vivo results. The decreased Cmax and prolonged Tmax and half-life were obtained with the ketorolac transdermal systems compared with oral administration, indicating that the ketorolac transdermal systems may have a prolonged effect with reduced toxic event. There was an excellent relationship found between in vitro permeation flux and in vivo AUC0−∞.
INTRODUCTION
Ketorolac is a nonsteroidal anti-inflammatory drug with potent analgesic and moderate anti-inflammatory activities by inhibiting prostaglandin synthesis (Rooks et al. Citation1985; Buckley and Brogden Citation1990). Ketorolac has been reported to have similar efficacy to narcotic analgesics, whereas it does not pose potential addiction problems and respiratory depression (Yee et al. Citation1986; O'Hara et al. Citation1987; Forbes et al. Citation1990; Stanski et al. Citation1990). Therefore, ketorolac is a relatively more favorable therapeutic agent for the management of moderate to severe pain (Tiwari and Udupa Citation2003). Although oral bioavailability of ketorolac was reported to be 90% with very low first-pass metabolism, its short biological half-life (4–6 hr) and many adverse effects, such as upper abdominal pain and gastrointestinal ulceration, restrict its oral use. (Buckley and Brogden Citation1990; Reinhart Citation2000).
To avoid invasive drug therapy such as injections and to eliminate frequent dosing regimen with oral administration, a transdermal drug delivery system has been considered as an alternative dosage form. We previously performed a study using solution formulations to examine the feasibility of ketorolac transdermal system. In that study (Cho and Gwak Citation2004), the binary cosolvent system composed of propylene glycol monolaurate (PGML)-diethylene glycol monoethyl ether (DGME) or propylene glycol monocaprylate (PGMC)-DGME at the ratio of 60:40 showed high permeation rate. Also, such saturated fatty acids as caprylic acid, capric acid, or lauric acid resulted in high permeation flux when they were added to propylene glycol (PG).
Several vehicles were selected based on the results from the study using solution formulations, and then pressure-sensitive adhesive (PSA) ketorolac transdermal systems were formulated. In the present study, we investigated the effects of PSAs and vehicles on the permeation of ketorolac from formulated ketorolac transdermal systems using a flow-through diffusion cell system. Also, the in vivo pharmacokinetic study was carried out using rats based on the in vitro permeation results.
MATERIALS AND METHODS
Ketorolac tromethamine, ketoprofen (internal standard, IS), caprylic acid, capric acid, lauric acid, oleic acid, and linoleic acid were purchased from Sigma Chemical Co. (St. Louis, MO, USA). PGMC (Capryol® 90), PGML (Lauroglycol® 90), and DGME (Transcutol® P) (Gattefossé, Gennevilliers Cedex, France) were used. Acetonitrile and methanol used were of HPLC grade. Acrylic PSA solutions in organic solvents were obtained from National Starch and Chemical Company (Bridgewater, NJ, USA). Other reagents were of analytical grade.
Analysis
Samples from permeation, stability, and pharmacokinetic studies were analyzed by high-performance liquid chromatography (HPLC) system (Shimadzu Scientific Instruments, Tokyo, Japan), consisting of a pump (LC-10AD), an automatic injector (SIL-10A), and an ultraviolet detector (SPD-10A) set at 300 nm. An ODS column (μBondapak C18, 3.9 × 300 mm, 10 μm, Waters, Milford, MA, USA) was used. The mobile phase was composed of acetonitrile, methanol, water, and triethylamine (45:10:45:0.1, v/v), whose pH was adjusted to 3.2 by phosphoric acid, and delivered at a flow rate of 1.0 mL/min.
Preparation of Pressure-Sensitive Transdermal Systems
Acrylic adhesive solutions were prepared by mixing 1 mL of ketorolac solutions in various vehicles with 3 g of acrylic solution in mixed solvents (). PSA transdermal systems were prepared by casting the above solutions on polyester release liner coated with silicone using a casting knife; the thickness spread was 300 μm. They were set at room temperature for 10 min to evaporate the solvents and then were oven-dried at 90°C for ∼20 min to remove the residual organic solvents. The dried film was transferred onto a backing film.
TABLE 1 Formulation compositions for the preparation of ketorolac transdermal systems
Stability of Pressure-Sensitive Adhesive Transdermal Systems
The prepared PSA transdermal systems were stored at room temperature. Formulated ketorolac transdermal systems were cut into 2 cm × 2.5 cm size and dissolved in 50 mL of 50% methanol immediately after preparation and at 15 and 30 days by sonicating for 2 hr. The solutions were assayed by HPLC.
Procedure for In Vitro Skin Permeation
Male hairless mice aged 6–8 weeks were used. After sacrificing with ether, the skin of each hairless mouse was excised. Then it was mounted on a flow-through diffusion cell system consisting of a multichannel peristaltic pump (205S, Watson Marlow, North Wilminton, MA, USA), a fraction collector (Retriever IV, ISCO, Lincoln, NE, USA), a circulating water bath (RB-10, Jeo Tech, Kimpo, Korea), and flow-through diffusion cells. The surface area of the receiver cell opening was 2 cm2, and the cell volume was 5.5 mL. The receiver cells were filled with pH 7.4 isotonic phosphate buffer solution, and the media were stirred by a Teflon-coated magnetic bar to keep them well mixed. The skin permeation studies were performed at 37°C.
Pharmacokinetic Studies
Male Sprague-Dawley rats weighing 280–320 g were obtained from Samtako Bio Co. (Osan, Korea). The rats were anesthetized with 1 mL/kg of ketamine hydrochloride (50 mg/mL, Yuhan Corp., Seoul, Korea) and the right femoral artery was cannulated using polyethylene tubes (0.58 mm i.d. × 0.96 mm o.d.; Naume Corp., Tokyo, Japan). After surgery, each animal was housed individually in cage. The animals fasted overnight until the end of the experiment but were allowed water ad libitum. Rats were then divided into 5 groups, comprising 6 rats each. Groups 1–5 were administered oral dosage form and transdermal delivery system (TDS) 1, 2, 3, and 4, respectively. The oral dose was 2487 μ g/kg, and the compositions of TDS 1, 2, 3, and 4 were FN 8, FN 10, FN 16, and FN 24, respectively, (). For the administration of TDS, the hair of left side between back and abdomen was shaved carefully so that the stratum corneum remained intact. The size of TDS applied to the shaved site of rat was 2 cm × 2.5 cm. Serum samples (0.1 mL) were collected before and 0.25, 0.5, 1, 2, 4, 6, 8, 12, and 24 hr after drug administration and analyzed by HPLC. The pharmacokinetic studies of ketorolac transdermal delivery systems were carried out according to the Principles for Biomedical Research Involving Animals developed by the Council for International Organizations of Medical Sciences and approved by the Ethical Review Committee at the Ewha Womans University.
Procedure for Serum Sample Preparation
The serum sample (0.1 mL) was mixed with 50 μ L of the working IS solution (30 μg/mL) and acidified with 30 μL of 1N hydrochloric acid. The mixture was added to 6 mL of n-hexane:ether (70:30, v/v), vortex-mixed for 10 min, and centrifuged for 5 min. Then 5 mL of organic phase were evaporated and the residue was reconstituted with 120 μL of mobile phase. And then 40 μL was injected directly into the HPLC system.
Statistical Analysis
The pharmacokinetic variables from the 5 groups were compared with a one-way ANOVA, followed by a posterior testing with an unpaired t-test using the Bonferroni correction. A p value of less than 0.05 was considered significant.
RESULTS AND DISCUSSION
Effect of Pressure-Sensitive Adhesives
To evaluate the effects of PSAs, four kinds of acrylic adhesives were employed; Duro-Tak®87-2196 (FN 4), 87-2100 (FN 1), 87-2510 (FN 3), and 87-2097 (FN 2) (). Fully 100 mg of ketorolac tromethamine were dissolved in 1 mL of methanol, and mixed with 3 g of PSA. As depicted in , the highest penetration rate was obtained with Duro-Tak®87-2196; the permeation rate of Duro-Tak®87-2196, 87-2100, 87-2097, and 87-2510 was 3.25 ± 1.69, 1.17 ± 0.07, 0.47 ± 0.15, and 0.32 ± 0.05 μ g/cm2/hr, respectively. The lag time was not quite different among samples, which was ∼8 hr. The steady-state flux (Js) and lag time (TL) are expressed as follows (Barry Citation1983): Js = DKC/h and TL = h2/6D (Js = the steady-state flux, D = diffusion coefficient, C = the solubility of drug in PSA, K = partition coefficient between skin and the PSA, h = the thickness of skin). Based on the results that the lag time was almost the same, the diffusion coefficient was estimated to be constant among PSAs used. Therefore, the difference of permeation flux was thought to be due to the KC, which is the solubility of ketorolac in the skin.
FIG. 1 Effect of PSA on the permeation of ketorolac through excised hairless mouse skin. Data were expressed as the mean ± S.D. (n = 3). • = 87–2100; ˆ = 87–2510; ▴ = 87–2196; ▵ = 87–2097.
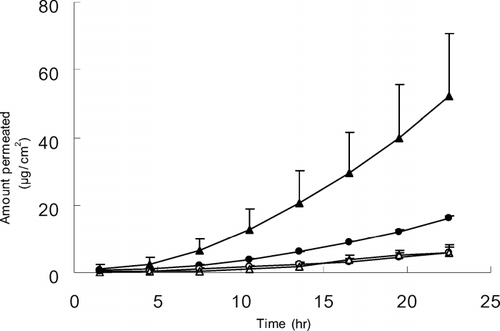
Although the underlying mechanism was not directly investigated in this study, the difference of functional group in PSAs may be involved in the solubility difference. The PSAs with carboxyl functional group (Duro-Tak®87-2196 and 87-2100) showed the higher permeation rate, followed by one without a functional group (Duro-Tak®87-2097). The acrylic adhesive with a hydroxyl functional group (Duro-Tak®87-2510) provided the lowest permeation rate. The copolymer type also affected the permeation flux, in which acrylate-vinylacetate (Duro-Tak®87-2196) produced better permeability than acrylate (Duro-Tak®87-2100). Thus, it was speculated that the permeation rate of a drug compound can be varied by the chemical nature and copolymer of PSAs. In the case of ketorolac, the highest permeation rate was achieved when PSA with carboxylic functional group and acrylate-vinylacetate copolymer was used.
The effects of drug loading doses were examined using methanol and Duro-Tak®87-2196 as a vehicle and PSA, respectively. The drug concentrations employed were 2 (FN 7), 5 (FN 6), 7 (FN 5), and 10% (FN 4) in methanol as described in . The permeation flux and lag time at 2, 5, 7, and 10% were 0.34 ± 0.14 μg/cm2/hr and 9.2 ± 2.8 hr; 0.55 ± 0.19 μg/cm2/hr and 8.8 ± 1.4 hr; 0.98 ± 0.21 μ g/cm2/hr and 12.7 ± 0.8 hr; and 3.25 ± 1.69 μ g/cm2/hr and 8.7 ± 1.0 hr, respectively. From the results, in which the drug loading in PSA transdermal system increased, the permeation flux also increased, indicating that high drug loading is required to achieve appropriate permeation rate.
Effect of Vehicles on the Permeation of Ketorolac
In designing a PSA transdermal drug delivery system, it is essential to find an appropriate vehicle that solubilizes a drug, mixes well with PSA, and/or enhances the permeation rate. From our previous study using solution formulations of ketorolac (Cho and Gwak Citation2004), PGML showed very high permeation rate, and the permeation rate was increased by the addition of DGME. Also, DGME-PGMC and PG-fatty acids cosolvents showed high permeation fluxes. These vehicles were used for the fabrication of ketorolac PSA transdermal systems to identify the optimum penetration enhancers, and they were satisfactory in meeting the conditions for PSA transdermal system. To examine the effects of vehicles, 50 mg of ketorolac tromethamine were dissolved in 1 mL of various solvents, and mixed with 3 g of Duro-Tak®87-2196 (FN 8–38).
The effects of cosolvents containing DGME-PGML (FN 8–13) and DGME-PGMC (FN 13–18) on the ketorolac permeation from PSA transdermal system were investigated. shows the fluxes of ketorolac at the various ratios of DGME-PGML and DGME-PGMC cosolvents. Both of the two cosolvents showed the highest fluxes at 40% of DGME, which were 8.2 ± 2.7 and 6.3 ± 1.0 μg/cm2/hr, respectively. This is consistent with the results from the study using solution formulations (Cho and Gwak Citation2004), even though the enhancement factors by the addition of DGME to PGMC or PGML in the PSA transdermal systems were much lower than those in solution formulations. The enhancing effect of the addition of DGME may be because DGME itself may not have a profound effect on the structural integrity of the skin. It just eases the partition of a compound by increasing the solubility of the compound in the skin. The DGME alone revealed low permeation rate (2.8 ± 0.8 μg/cm2/hr).
FIG. 2 Effect of PGML-DGME and PGMC-DGME cosolvents on the steady-state flux of ketorolac through excised hairless mouse skin. Data were expressed as the mean ± S.D. (n = 3). • = DGME-PGMC; ▴ = DGME-PGML.
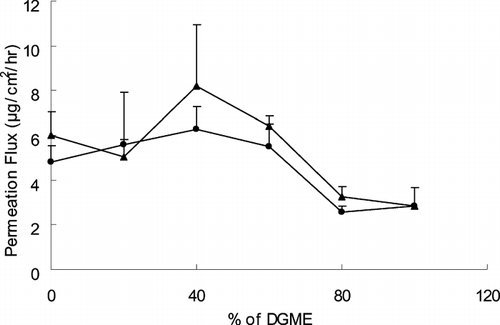
Fatty acids also are known to be enhancers with lipophilic properties, and many studies have shown that the skin permeability enhancing effects of fatty acids are greatest with PG vehicles (Cooper Citation1984; Cooper, Merritt, and Smith Citation1985; Aungst, Rogers, and Shefter Citation1986; Yamada, Uda, and Tanigawara Citation1987). The binary system was considered to disorganize the multilaminate hydrophilic-lipophilic layers located intercellularly in the stratum corneum, consequently promoting percutaneous absorption of drugs (Nomura et al. Citation1990). In this study, various concentrations of fatty acids were used with PG as vehicles shown in (FN 19–38).
In our earlier study using solution formulations (Cho and Gwak Citation2004), the highest permeation flux was attained with 10% caprylic acid in PG. As depicted in , the permeation flux from PSA transdermal system containing caprylic acid in PG, however, was low compared with systems containing other fatty acids. This result indicated that the effects of vehicles in the solution formulations may not be extrapolated to predict their effects in PSA transdermal systems. The overall permeation fluxes from ketorolac PSA transdermal systems containing fatty acids in PG ranged from 1.0 to 4.5 μg/cm2/hr, which was considerably low compared with solution formulations (1.3–113.6 μg/cm2/hr) from our previous study (Cho and Gwak Citation2004).
FIG. 3 The effects of fatty acids in PG on the permeation flux (A) and lag time (B) of ketorolac from ketorolac PSA transdermal systems. Data were expressed as the mean ± S.D. (n = 3). ▪ = caprylic acid; □ = capric acid;


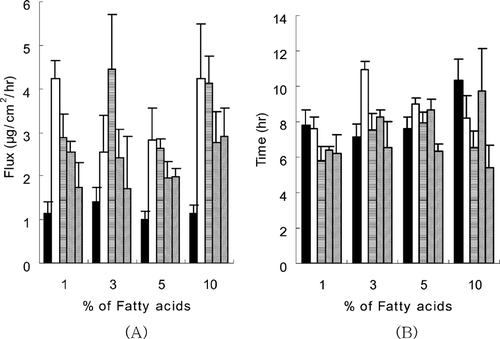
The overall low permeation rate was attributable to the lower loading dose (264–298 μg) applied to the 2 cm2 receiver cells (), compared with that of solution formulations (1500 μ g). In addition, the low permeation flux of ketorolac from PSA ketorolac transdermal systems was partly attributed to the low thermodynamic activity due to its high solubility in the acrylic adhesive matrix. The overall lag times of PSA transdermal delivery systems containing fatty acids in PG ranged between 5–11 hr. Among them, the lag time of PSA transdermal system containing linoleic acid in PG was relatively short at all concentrations used (). The longest lag time (11.0 hr) was observed at the formulation of 3% capric acid in PG.
TABLE 2 Stability of ketorolac transdermal delivery systems (TDS) containing various permeation enhancers
Physicochemical Stability
The stability of formulated ketorolac transdermal systems was evaluated. As shown in , the initial concentration remaining after 30 day storage was 90–100 %, which was not degraded significantly, regardless of the formulations. The appearance or adhesive property after 30-day storage at room temperature did not change.
Pharmacokinetics in Rats
Based on the in vitro results from permeation of ketorolac from PSA transdermal systems, PGML, PGML-DGME (60:40, v/v), PGMC-DGME (60:40, v/v), and 3% capric acid in PG were employed as vehicles for the in vivo pharmacokinetic study of ketorolac transdermal systems. The initial drug doses from transdermal delivery systems after drying were 661–746 μg (), whereas the oral dose was 746 μg for the mean rat weight of 300 g used in this study.
We found statistically significant differences in the Cmax (p < 0.01) and Tmax (p < 0.05) between groups as shown in and . The statistically significantly lower Cmaxwere obtained from TDS 1, 3, and 4 compared with oral delivery (p < 0.05). Also, TDS 3 revealed more than 10 times increased Tmax (p < 0.05) and TDS 4 showed more than two times t1/2 (p < 0.05), compared with oral administration. The overall decreased Cmax and increased Tmaxand t1/2 were observed in transdermal systems compared with oral administration even though all are not statistically significant. The Tmax and half-life of ketorolac by oral administration were 0.3 ± 0.05 and 3.6 ± 0.5 hr, respectively, which were similar to those investigated in other studies (Grandos-Soto et al. Citation1995; Pasloske et al. Citation1999). The high AUC0−∞ and AUC0−24 values were attained with TDS 2 and TDS 3, which used PGML-DGME (60:40) and PGMC-DGME (60:40) as a vehicle, respectively, and the values were similar to those of oral administration.
FIG. 4 Ketorolac serum concentrations after administration by oral and transdermal delivery systems containing various permeation enhancers. Data were expressed as the mean ± S.E. (n = 3). • = oral; ˆ = PGML; ▴ = PGML-DGME (60:40); ▵ = PGMC-DGME (60:40); ▪ = 3% capric acid in PG.
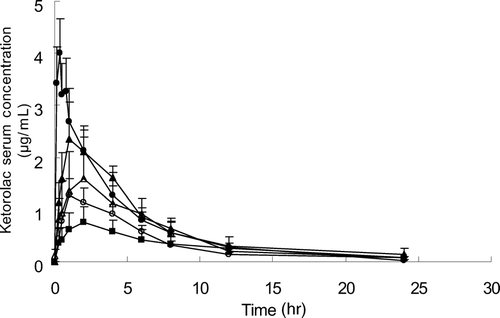
TABLE 3 Pharmacokinetic parameters of kerorolac from oral and transdermal delivery system (TDS) administration
The relationship between in vitro permeation flux through excised hairless mouse skin and in vivo AUC0−∞ in rats is depicted in . A good relationship (r = 0.9576) between Js and AUC0−∞ was obtained. We thought that the in vivo plasma level of ketorolac can be predicted from the in vitro permeation profiles.
CONCLUSION
Ketorolac transdermal systems containing PGMC or PGML in combination with DGME at the ratio of 60:40 resulted in relatively high permeation rate from the in vitro study. When the ketorolac transdermal systems containing PGML-DGME (60:40) or PGMC-DGME (60:40) were applied to rat skins, high values of AUC0−∞ were attained, which were similar to that of oral administration. We found an excellent relationship between in vitro Js and in vivo AUC0−∞. From the results of the reduced Cmax and prolonged Tmax and half-life in PSA transdermal systems, we speculated that the ketorolac transdermal systems may have a prolonged effect with reduced toxic event, compared with oral delivery.
REFERENCES
- Aungst B. J., Rogers N. J., Shefter E. Enhancement of naloxone penetration through human skin in vitro using fatty acids, fatty alcohols, surfactants, sulfoxides and amides. Int. J. Pharm. 1986; 33: 225–234
- Barry B. W. Dermatological Formulations. Marcel Dekker, New York 1983; 49–94
- Buckley M. M., Brogden R. N. Ketorolac. A review of its pharmacodynamic and pharmacokinetic properties, and therapeutic potential. Drugs 1990; 39: 86–109
- Cho Y. A., Gwak H. S. Transdermal delivery of ketorolac tromethamine: effects of vehicles and penetration enhancers. Drug Dev. Ind. Pharm. 2004; 30: 557–564
- Cho Y. J., Choi H. K. Enhancement of percutaneous absorption of ketoprofen: effect of vehicles and adhesive matrix. Int. J. Pharm. 1998; 169: 95–104
- Cooper E. R. Increased skin permeability for lipophilic molecules. J. Pharm. Sci. 1984; 73: 153–1156
- Cooper E. R., Merritt E. W., Smith R. L. Effect of fatty acids and alcohols on the penetration of acyclovir across human skin in vitro. J. Pharm. Sci. 1985; 74: 688–689
- Forbes J. A., Butterworth G. A., Burchfield W. H., Beaver A. T. Evaluation of ketorolac, aspirin and acetaminophen-codeine combination in postoperative oral surgery pain. Pharmacotherapy 1990; 10: 77S–93S
- Grandos-Soto V., Lopez-Munoz F. J., Hong E., Fluores-Murrieta F. J. Relationship between pharmacokinetics and the analgesic effect of ketorolac in the rat. J. Pharmacol. Exp. Ther. 1995; 272: 352–356
- Nomura H., Fusao K., Sugimoto Y., Miyashita Y., Dohi M., Kato Y. Percutaneous absorption of indomethacin from mixtures of fatty alcohol and propylene glycol (FAPG bases) through rat skin: effects of oleic acid added to FAPG base. Chem. Pharm. Bull. 1990; 38: 1421–1424
- O'Hara D. A., Fragen R. J., Kinzer M., Pemberton D. Ketorolac tromethamine as compared with morphine sulfate for treatment of postoperative pain. Clin. Pharmacol. Ther. 1987; 41: 556–561
- Pasloske K., Renaud R., Burger J., Conlon P. Pharmacokinetics of ketorolac after intravenous and oral single dose administration in dogs. J. Vet. Pharmacol. Ther. 1999; 22: 314–319
- Reinhart D. I. Minimizing the adverse effects of ketorolac. Drug Safety 2000; 22: 487–497
- Rooks W. H., Maloney P. J., Shott L. D., Schuler M. E., Sevelius H., Strosberg A. M., Tanenbaum L., et al. The analgesic and anti-inflammatory profile of ketorolac and its tromethamine salt. Drugs Exp. Clin. Res. 1985; 11: 479–492
- Stanski D. R., Cherry C., Bradley R., Sarnquist F. H., Yee J. P. Efficacy and safety of single doses of intramuscular ketorolac tromethamine compared with meperidine for postoperative pain. Pharmacotherapy 1990; 10: 40S–44S
- Tiwari S. B., Udupa N. Investigation into the potential of iontophoresis facilitated delivery of ketorolac. Int. J. Pharm. 2003; 260: 93–103
- Yamada M., Uda Y., Tanigawara Y. Mechanism of enhancement of percutaneous absorption of molsidomine by oleic acid. Chem. Pharm. Bull. 1987; 35: 3399–3406
- Yee J. P., Koshiver J. V., Allbon C., Brown C. R. Comparison of intramuscular ketorolac tromethamine and morphine sulfate for analgesia of pain after major surgery. Pharmacotherapy 1986; 6: 253–261