Abstract
The aim of this study was to develop chitosan-based materials in drug delivery systems possessing covalent attachment of thiol moieties. Thiol-containing chitosan (TCS), found to be soluble in water, was synthesized by graft copolymerization technique. The TCS beads were prepared by using tripolyphoshate, at pH 4.0. The morphology of TCS beads was examined by scanning electron microscopy. The in vitro drug release behavior was studied in phosphate buffer solution at various pH, using indomethacin as a model drug at two different concentrations (0.3 and 0.6% w/w). The release amounts of indomethacin from TCS beads were higher increasing pHs in the dissolution medium. The release rate of indomethacin at pH 7.4 was higher than the release rate at pH 1.4 due to ionization of thiol groups and high solubility of indomethacin in an alkaline medium. These results indicated that the TCS beads may become a delivery system for the controlled release of different drugs wherever pH sensitive mechanics might be useful. This is especially applicable in cases when it is important to minimize drug release in acidic sites, such as in the stomach.
Chitosan (CS) is obtained by alkaline deacetylation of chitin, which is one of the most abundant polysaccharides in nature. Shell wastes of shrimp, lobster, and crab are the main industrial sources of chitin (Muzzarelli Citation1997; Sorlier et al. Citation2001). CS is a polysaccharide consisting of copolymers of glucosamine and N-acetylglucosamine. The primary amino group accounts for the possibility of relatively easy chemical modification of chitosan and salt formation with acids. At acidic pH the amino groups are protonated, which promotes solubility, whereas chitosan is insoluble at alkaline and neutral pH (Fini and Orienti Citation2003). Because of its favorable properties, such as enzymatic biodegradability, nontoxicity, and biocompatibility (Muzzarelli Citation1997; Sorlier et al. Citation2001; Fini and Orienti Citation2003), chitosan has received considerable attention as a novel carrier in drug delivery systems and has been included in the European Pharmacopoeia since 2002. So far, chitosan has been utilized in various fields of pharmaceutical dosage forms, such as tablets, gels, and microspheres, and as mucoadhesive and/or permeation-enhancing excipient for oral, nasal, ocular, and buccal drug delivery (Takeuchi, Yamamoto, and Kawashima Citation2001; Felt et al. Citation1999; Senel et al. Citation2000), and in nonviral gene delivery (Borchard Citation2001; Liu and Yao Citation2002).
Interest is a growing in the chemical modification of CS to improve its solubility and widen its applications (Sugimoto, Morimoto, and Sashiwa Citation1998; Sashiwa and Shigemasa Citation1999; Heras, Rodriguez, and Ramos Citation2001). Among various methods, graft copolymerization is most attractive because it is a useful technique for modifying the chemical and physical properties of natural polymers. CS bears two types of reactive groups that can be grafted. First, the free amino groups on deacetylated units and second, the hydroxyl groups on the C3 and C6 carbons deacetylated units. Grafting of CS allows the formation of functional derivatives by covalent binding of a molecule, the graft, onto the CS backbone. Recently different researchers showed that after primary deviation followed by graft modification, CS would obtain much improved water solubility and bioactivities such as antibacterial and antioxidant properties (Xie et al. Citation2001, Citation2002; Jayakumar et al. Citation2005). Grafting CS is a common way to improve chitosan properties such as increasing chelating (Yang and Yuan Citation2001) or complexation properties (Chen and Wang Citation2001), bacteriostatic effect (Jung et al. Citation1999), or enhancing adsorption properties (Kotze et al. Citation1997; Thanou, Verhoef, and Junginger Citation2001). Although the grafting of CS modifies its properties, it is possible to maintain some interesting characteristics such as mucoadhesivity (Hoffman et al. Citation1997), biocompatibility (Tasker et al. Citation1998; Ono et al. Citation2000), and biodegradability (Singh and Ray Citation1998).
When compared with other modified-chitosan materials, thiolated chitosans have numerous advantageous features, such as significantly improved mucoadhesive and permeation enhancing properties (Schnurch and Hornof Citation2001; Kast and Schnurch Citation2001; Hornof, Kast, and Schnurch Citation2003; Schnurch, Brandt, and Clausen Citation1999, Citation2003; Clausen, Kast, and Schnurch Citation2002). Moreover, solutions of thiolated chitosans display in situ gelling properties at physiological pH values (Hornof et al. Citation2003). The strong cohesive properties of thiolated chitosans make them highly suitable carriers for controlled drug release applications (Schnurch et al. Citation1999; Kast et al. Citation2002).
However, to our knowledge no work has been published on thiolated-chitosan particles or beads and its applications in the delivery of drugs and other substances. In this work, thiolactic acid was grafted into chitosan by using 1-ethyl-3-(3-dimethylaminopropyl) carbodiimide by means of coupling reaction. The grafted chitosan was used for the preparation of beads using tripolyphosphate to improve using this controlled release system in contact with a gastric fluid, for instance. The morphological features of the system, swelling properties, and the in vitro drug release profiles of the prepared beads have been evaluated.
MATERIALS AND METHODS
Chitosan (deacetylation degree 75–85%), tripolyphosphate (TPP), and thiolactic acid were received from Aldrich (Milwaokee, WI, USA). 1-ethyl-3-(3-dimethylaminopropyl) carbodiimide (EDC) was received from Alfa Aesar Company (Ward Hill, MA, USA). Indomethacin drug was purchased from Fluka (Buchs, Switzerland). All other materials used were analytical grade.
Synthesis of Thiol-Containing Chitosan (TCS)
CS (0.1650 g) was dissolved in 1% w/w acetic acid solution. Then thiolactic acid (0.1055 g) was dissolved in water and then added dropwise to the CS solution. Then, EDC (0.1552 g) was added to the above reaction mixture. The pH of the solution was maintained at 5.0. The reaction was conducted at 50°C for 7 hr. Then the reaction mixture was poured into acetone solution (200 mL) for precipitation. The precipitate was washed repeatedly with ethanol and acetone. The product was dried in vacuum at room temperature. The synthesis of TCS was shown in Diagram 1.
Preparation of TCS Beads
Thiol-containing chitosan TCS beads were prepared by using ionotropic gelation process with counter polyanion TPP. TCS (0.7006 g) was dissolved in 10 ml of a 2% w/w acetic acid solution and stirred for 1 day to obtain a transparent and homogeneous solution. The TCS solution with or without drug was dropped through a syringe needle (0.5 mm in diameter) into the 10% TPP aqueous phase. Solidified white beads were formed immediately and allowed to stand for 1 hr in the solution with occasional agitation at room temperature. The pH of the TPP solution was adjusted to pH 4.0 with a 1M HCl solution. The gel beads were filtered, washed with distilled water repeatedly, and dried under vacuum at room temperature for 2 days. For the preparation of drug-loaded TCS beads two different amounts of indomethacin (IM) (0.3 and 0.6% w/w with respect to TCS solution) were dispersed in 10 g of TCS solution for 1 day. IM is a nonsteroid anti-inflammatory drug (NSAID) used to treat arthrthitis (Mi et al. Citation2002a). The advantages of such controlled release formulations containing NSAID over the conventional dosage forms were reported earlier (Kulkarni et al. Citation1999, Citation2001). Such formulations help to minimize the serious gastric irritation side effects of the conventional-dosage NSAID formulations. CS beads with or without IM were prepared from the same procedure.
Swelling Tests
Dried CS and TCS beads were carefully weighed and immersed in phosphate buffer media (50 mL) with pH values at 1.4 and 7.4 at 37°C. At predetermined time intervals, swollen beads were taken out, and the excess water was blotted with filter paper from the surface, and then weighed on a sensitive balance. The following equation was used to determine the swelling degree.
Here Xd and Xw represent the mass of dry and swollen beads, respectively.
Determination of Drug-Loading Efficiency
During the bead preparation process, the aqueous phase was collected, and the drug content in the aqueous phase was determined by ultraviolet spectrophotometer at 320 nm. Then the loading efficiency during bead preparation process was calculated by the following formula:
Where m, c, and v represent the initial drug (IM) mass in beads (TCS) droplet, the IM concentrations in TPP solution, and the volume of TPP solution, respectively.
In Vitro Drug Release Studies
The in vitro release tests were carried out on all formulations of drug-loaded CS beads, and TCS beads were suspended in 100 mL of phosphate buffer at various pH at 37°C and placed in an incubated shaker at 120 rpm. At predetermined time intervals, 1 mL aliquot of sample was withdrawn and after suitable dilution, the concentration of drug released was monitored by ultraviolet spectrophotometer at 320 nm. The dissolution medium was replaced with fresh buffer to maintain the total volume. The drug release percent can be determined by the following equation:
Where L and Rt represent the initial amount of drug loaded and the cumulative amount of drug released at time t, respectively.
Instruments
The surface morphology of samples was analyzed by scanning electron microscopy (Leica Cambridge S360 microscope, UK). The drug release was monitored by ultraviolet spectrophotometer (Shimadzu UV-1601, Japan). The infrared spectrum was recorded with a double-beam Perkin-Elmer 1600 FTIR spectrophotometer.
RESULTS AND DISCUSSION
Synthesis of TCS
Thiolactic acid was attached covalently to the primary amino group of chitosan under the formation of amide bonds as shown in Diagram 1. The carboxylic acid moieties of thiolactic acid were activated by EDC forming as O-acylurea derivative as intermediate product that reacts with the primary amino groups of chitosan. The TCS was found to be soluble in both water and acetic acid. The yield of the TCS was 86%. The FTIR spectrum of TCS and CS is shown in . The FTIR spectrum of CS () showed a broad –OH stretching absorption band between 3450 and 3100 cm− 1 and the aliphatic C-H stretching between 2990 and 2850 cm− 1. As the –OH stretching band and the aliphatic C-H stretching band were appeared between 3450 and 2850 cm− 1 in the spectrum, Another major absorption band between 1220 and 1020 cm− 1 represented the free primary amino group (–NH2) at C2 position. The peak at 1647 cm− 1 represented acetylated amino group of chitin, which indicated that the sample is not fully deacetylated. The peak at 1384 cm− 1 represents the –C–O stretching of primary alcoholic group (–CH2–OH). The adsorption peaks at 3434 cm− 1 is due to –NH stretching. The peaks at 1646 and 1578 cm− 1 are due to the formation of amide linkage (–NHCO linkage). The peaks at 1254, 1154, and 896 cm− 1 are due to thiol groups. The above peaks confirmed the structure of TCS.
SEM of Prepared TCS Beads
shows SEM images of drug-free CS and TCS beads. The liquid-gel transition of TCS in TPP aqueous solution can be explained by the electrostatic interaction between positively charged free amino groups with negatively charged counteranion TPP (Mi et al. Citation1999). The CS beads have a regular spherical shape, smooth surface, and homogeneous polymer matrix. This may be because cross-linking is more efficient in the CS beads. The TCS beads have some cracks on the surface that may be due to the grafting of thiol-containing monomer. The insufficient cross-linking of TCS beads as the amino groups of CS were substituted by thiol compound has lead to the formation of rough and nonuniform surface structure. The size of the CS and TCS beads was found to vary in between 1.5–1.75 mm.
Swelling Behaviors of Beads
The swelling behavior of the CS and TCS beads in solutions of pH 1.4 and 7.4 at 37°C are shown in and . The TCS beads at pH 1.4 were found to disintegrate within 3 days and then dissolve. The CS beads slowly swell and were found to be dissolved after 4 days. The results could be associated with differences in cross-linking density (Lee et al. Citation2001). The lower cross-linked TCS beads swelled quickly and gradually dissolved within 3 days, whereas the higher cross-linked beads did not dissolve within the same time. This is expected behavior, attributed to the high amount of interchain linkages in the well cross-linked chitosan-TPP network. The swelling percentage was much higher at pH 1.4 than pH 7.4. Moreover, the swelling reached a stable equilibrium much more rapidly in pH 7.4 than in pH 1.4. This is due to the lack of protonation of amino groups at pH 7.4, which prevents swelling in alkaline medium (Gupta and Ravi Kumar Citation2000).
Entrapment of Drug in TCS Beads
IM drug was efficiently entrapped into TCS beads during ionotropic process (see ). The relatively high efficiency of loading could be explained by the low water solubility of IM as well as the ionic binding between the carboxyl group of IM and the thiol group in TCS beads. The carboxyl groups in IM and the thiol group in TCS are ionized at pH 4. In this work, the drug was not chemically attached to the polymer. Thus, there will be only both electrostatic and hydrophobic interactions as well as the effect within the polymer matrix. Therefore, the drug is expected in a biologically active form and can exert its therapeutically effect upon the body as soon as it is released from the polymeric matrix.
TABLE 1 Loading efficiencies for IM-loaded CS and TCS Beads
Im In Vitro Release Profiles
shows the release profiles of 0.3% of IM-TCS beads at various pH, at 37°C. Within 3 hr, 27% of IM was released from TCS beads at pH 1.4 and 44% at pH 7.4. These results showed that the drug release profiles of TCS are in fact pH sensitive. Moreover, the more noticeable changes occur within a narrow pH range between 5.8 and 7.4. The following factors are to be considered in the noticeably higher release rate of IM at pH 7.4 than that at pH 1.4. The first factor is the higher solubility of IM in alkaline medium. The second factor is an electrostatic and hydrophobic repulsion between the carboxyl group of IM and the methyl group of modified TCS in pH 7.4 media (Hadgraft and Valenta Citation2000; Mi et al. Citation2002). This study shows that the percentages of drug release increase with an increasing pH in dissolution medium. The similar drug release profile also was observed for the CS beads. However, the release percentage of IM at pH 1.4 was found to be less than that of TCS beads. The reason may be the formation of complexes between carboxyl group of IM and amino group of CS and poor solubility of drug at pH 1.4 (Mi et al. Citation2002). The velocity of diffusion of drug molecules is higher in the alkaline medium, effectively contributing to the controlled drug release. The polymer matrix probably combines two types of mechanisms for drug release: controlled diffusion and swelling.
Thiolated chitosans also display, besides their strong mucoadhesive and permeation-enhancing properties, excellent cohesive properties. The reducing thiol functions on the chitosan backbone enable the glycol proteins to also form inter- as well as intramolecular disulfide bonds. Such a cross-linking of the polymeric chains results in a high stability of drug carrier systems based on thiolated chitosans (Schnurch, Hornof, and Guggi Citation2004). shows the influence of the initial drug loading on the release percent of IM drug from TCS beads at pH 1.4 and 7.4. There is no significant change between the release trends of 0.3 and 0.6% IM-loaded TCS beads. The amount of drug released from high drug-loaded beads is higher than that from low drug-loaded beads. Increasing the initial drug content provides the larger equilibrium amount of drug released in each pH.
FIG. 6 Drug release from 0.3 and 0.6% of IM-loaded TCS beads in simulated gastric fluid, pH 1.4, and simulated intestinal fluid, pH 7.4.
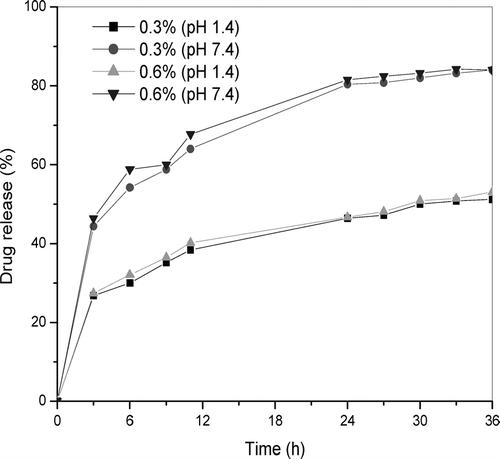
shows the in vitro release profiles of 0.6% IM-loaded CS and TCS beads investigated at pH 1.4 and 7.4. At pH 1.4, 25% of IM was released from CS beads within 3 hr while for TCS beads the amount of IM released was higher (27%). Fully 38% of IM was released from CS beads at pH 7.4 within 3 hr while this value is increased to 44% of IM for TCS beads. In the case of CS beads, the complex is formed between the amino group of CS and carboxyl group of IM. In the TCS beads there is a hydrophobic interaction between the carboxyl group of IM and the methyl group of thiol-containing chitosan (Mi et al. Citation2002). TCS beads released the IM drug much faster than that of CS beads. When thinking of drug delivery applications, these improved mucoadhesive properties along with a controlled release out of the polymer matrix should render TCS beads very promising tools. Their positive result is due to the expected increase of drug bioavailability by adhering to mucosal tissues. Because of these properties, TCS beads should be further investigated.
CONCLUSION
Novel TCS beads have been synthesized for use as a controlled drug delivery system. The beads were prepared under mild conditions at room temperature at pH 4.0, and the loading efficiency of a model drug, indomethacin, was over 85%. The TCS beads were found to be pH sensitive. The water solubility of the drug, the swelling degree of beads, and the ionization of thiol groups influenced the release profiles of the drug at various pHs. The release rate in simulated intestinal fluid (pH 7.4) is higher than that in simulated gastric fluid (pH 1.4), enabling the drug delivery or release to take place preferentially in the intestine, avoiding drug leakage in the stomach. According to our results, TCS beads represent a promising tool as successful drug carriers for controlled drug delivery systems.
R. Jayakumar thanks the Portuguese Foundation for Science and Technology for providing him with a Post-Doctoral Research Fellowship (SFRH/BPD/14670/2003). This work was partially supported by the FCT Foundation for Science and Technology, through funds from the POCTI and/or FEDER programs, and by the European Union-funded STREP Project HIPPOCRATES (NMP3-CT-2003-505758).
REFERENCES
- Borchard G. Chitosans for gene delivery. Adv. Drug Del. Rev. 2001; 52: 145–150
- Chen S., Wang Y. Study on β-cyclodextrin grafting with chitosan and slow release of its inclusion complex with radioactive iodine. J. Appl. Polymer Sci. 2001; 82: 2414–2421
- Clausen A. E., Kast C. E., Schnurch A. B. The role of glutathione in the permeation enhancing effect of thiolated polymers. Pharm. Res. 2002; 19: 602–608
- Felt O., Furrer P., Mayer J. M., et al. Topical use of chitosan in ophthalmology: tolerance assessment and evaluation of precorneal retention. Int. J. Pharm. 1999; 180: 185–193
- Fini A., Orienti I. The role of chitosan in drug delivery: current and potential applications. Am. J. Drug Del. 2003; 1: 43–59
- Gupta K. C., Ravi, Kumar M. N. V. Drug release behavior of beads and microgranules of chitosan. Biomaterials 2000; 21: 1115–1119
- Hadgraft J., Valenta C. pH, pKa and dermal delivery. Int. J. Pharm. 2000; 200: 243–247
- Heras A., Rodriguez N. M., Ramos V. M. N-methylene phosphonic chitosan: a novel soluble derivative. Carbohydrate Polymers 2001; 44: 1–8
- Hoffman A. S., Chen G., Wu X., et al. Graft copolymers of PEO-PPO-PEO triblock polyethers on bioadhesive polymer backbones: synthesis and properties. Polymer Preprints 1997; 38: 524–525
- Hornof M., Kast C. E., Schnurch A. B. In vitro evaluation of the viscoelastic behavior chitosan-thioglycolic acid conjugates. Eur. J. Pharm. Biopharm. 2003; 55: 185–190
- Jayakumar R., Prabaharan M., Reis R. L., Mano J. F. Graft copolymerized chitosan: present status and applications. Carbohydrate Polymers 2005; 62: 142–158
- Jung B. O., Kim C. H., Choi K. S., et al. Preparation of amphiphilic chitosan and their antimicrobial activities. J. Appl. Polymer Sci. 1999; 72: 1713–1719
- Kast C. E., Schnurch A. B. Thiolated polymers-thiomers: development and in vitro evaluation of chitosan-thioglycolic acid conjugates. Biomaterials 2001; 22: 2345–2352
- Kast C. E., Valenta C., Leopold M., Schnurch A. B. Design and in vitro evaluation of a novel bioadhesive vaginal drug delivery system for clotrimazole. J. Control. Rel. 2002; 81: 347–354
- Kotze A. F., Luessen H. L., Leeuw B. J. D., et al. N-Trimethyl chitosan chloride as a potential absorption enhancer across mucosal surfaces: in vitro evaluation in intestinal epithelial cells (CaCo-2). Pharm. Res. 1997; 14: 1197–1202
- Kulkarni A. R., Soppimath K. S., Aminabhavi T. M. Controlled release of diclofenac sodium from sodium alginate beads crosslinked with glutaraldehyde. Pharma. Acta Helv. 1999; 74: 29–36
- Kulkarni A. R., Soppimath K. S., Aminabhavi T. M. In-vitro release kinetics of cefadroxil-loaded sodium alginate interpenetrating network beads. Eur. J. Pharm. Biopharma. 2001; 51: 127–133
- Lee S. T., Mi F. L., Shen Y. J., Shyu S. S. Equilibrium and kinetic studies of copper(II) ion uptake by chitosan-tripolyphosphate chelating resin. Polymer 2001; 42: 1879–1892
- Liu G. W., Yao K. D. Chitosan and its derivatives—a promising non-viral vector for gene transfection. J. Control. Rel. 2002; 83: 1–11
- Mi F. L., Shyu S. S., Chen C. T., Lai J. Y. Adsorption of indomethacin onto chemically modified chitosan beads. Polymer 2002a; 43: 757–765
- Mi F. L., Shyu S. S., Lee S. T., Wong T. B. Kinetic study of chitosan-tripolyphosphate complex reaction and acid-resistive properties of the chitosan-tripolyphosphate gel beads prepared by in-liquid curing method. J. Polymer Sci., Part-B. Polymer Physics 1999; 37: 1551–1564
- Mi F. L., Sung H. W., Shyu S. S. Drug release from chitosan-alginate complex beads reinforced by a naturally occurring cross-linking agent. Carbohydrate Polymers 2002b; 48: 61–72
- Muzzarelli R. A. A. Cell Molecular and Life Science 1997; 53: 131–140
- Ono K., Saito Y., Yura H., Ishikawa K. Photocrosslinkable chitosan as a biological adhesive. J. Biomed. Mat. Res. 2000; 49: 289–295
- Sashiwa H., Shigemasa Y. Chemical modification of chitin and chitosan 2: preparation and water soluble property of N-acylated or N-alkylated partially deacetylated chitins. Carbohydrate Polymers 1999; 39: 127–138
- Schnurch A. B., Brandt U. M., Clausen A. E. Synthesis and in vitro evaluation of chitosan-cysteine conjugates. Sci. Pharm. 1999; 67: 196–208
- Schnurch A. B., Hornof T. E. Synthesis and in vitro evaluation of chitosan-thioglycollic acid conjugates. Sci. Pharm. 2001; 69: 109–118
- Schnurch A. B., Hornof M., Guggi D. Thiolated chitosans. Eur. J. Pharm. Biopharm. 2004; 57: 9–17
- Schnurch A. B., Hornof M., Zoidl M. Thiolated polymers-thiomers:modification of chitosan with 2-iminothiolane. Int. J. Pharm. 2003; 260: 229–237
- Senel S., Kremer M., Kas S., et al. Enhancing effect of chitosan on peptide drug delivery across buccal mucosa. Biomaterials 2000; 21: 2067–2071
- Singh D. K., Ray A. R. Characterization of grafted chitosan films. Carbohydrate Polymers 1998; 36: 251–255
- Sorlier P., Denuziere A., Viton C., Domard A. Relation between the degree of acetylation and the electrostatic properties of chitin and chitosan. Biomacromolecules 2001; 2: 765–772
- Sugimoto M., Morimoto M., Sashiwa H. Preparation and characterization of water-soluble chitin and chitosan derivatives. Carbohydrate Polymers 1998; 36: 49–59
- Takeuchi H., Yamamoto H., Kawashima Y. Mucoadhesive nanoparticulate systems for peptide drug delivery. Adv. Drug Del. Rev. 2001; 47: 39–54
- Tasker R. A., Connel B. J., Ross S. J., Elson C. M. Development of an injectable sustained-release formulation of morphine: antinociceptive properties in rats. Lab. Animals 1998; 32: 270–275
- Thanou M., Verhoef J. C., Junginger H. E. Oral drug absorption enhancement by chitosan and its derivatives. Adv. Drug Del. Rev. 2001; 52: 117–126
- Xie W. M., Xu P. X., Wang W., Liu Q. Antioxidant activity of water-soluble chitosan derivatives. Biomat. Medi. Chemi. Lett. 2001; 13: 1699–1701
- Xie W. M., Xu P. X., Wang W., Liu Q. Preparation and antibacterial activity of a water-soluble chitosan derivative. Carbohydrate Polymers 2002; 50: 35–40
- Yang Z. K., Yuan Y. Studies on the synthesis and properties of hydroxyl azacrown ether-grafted chitosan. J. Appl. Polymer Sci. 2001; 82: 1838–1843