Abstract
The oral administration of nonsteroidal anti-inflammatory drugs (NSAIDs) is often associated with upper gastrointestinal tract side effects. To reduce these effects and improve the therapeutic efficacy, NSAIDs are often formulated as controlled release systems. We have prepared a new formulation consisting of dextran hydrogels containing NSAIDs as pendant agents, through ultraviolet irradiation of solutions of dextran functionalized with methacrylic groups in the presence of the drug derivatized in the same way. Release studies of different drugs from this system, carried out in media simulating the gastrointestinal tract, have demonstrated that the amount of released drug is strictly related to the concentration of the polymer in the solution submitted to irradiation as well as to its derivatization degree. Our obtained data confirm that the system is able to realize a colon-specific drug delivery.
INTRODUCTION
Nonsteroidal anti-inflammatory drugs (NSAIDs) are among the most prescribed medications in the world for their efficacy as anti-inflammatory, antithrombotic, anti pyretic, and analgesic agents. Numerous adverse effects, especially toward the gastrointestinal tract and including a high incidence of gastric and duodenal ulceration, have been reported as a consequence of their use (Langman Citation2003). To reduce gastrointestinal symptoms and prolong drug activity, NSAIDs are often formulated as modified delivery systems, such as enteric-coating or sustained-release methods. The research of systems more efficient than those already present on the market continuously increases, and this trend is confirmed by the relevant number of patents and papers reported in the literature in the past year (Arica et al.; Freitas and Marchetti; Landgraf, Li, and Benson Citation2005).
Different biodegradable polymers have been used as polymeric carriers as well as starting materials for the preparation of hydrogels used for modified release formulations (Kamath and Park Citation1993; Peppas et al. Citation2000). A possible approach for a colon drug release is yielded by pH-sensitive systems, having carboxylic groups in side chain (Mandracchia et al. Citation2004; Giannuzzo et al. Citation2006). These systems, as a consequence of the presence of ionizable groups, show a swelling degree lower at acid pH than in neutral one. For this reason, they are able to retain a high percentage of drug in acid solution simulating the conditions of the gastric tract, releasing it in a prolonged way in a medium simulating the intestinal fluid. However, even with such “intelligent hydrogels,” a not-negligible amount of drug is released during the transit in the stomach. To obtain a more efficient system for the controlled release of NSAIDs we have planned to synthesize hydrogels having the active molecules bound covalently to the polymeric matrix. This link has been obtained in one step during the network formation through a photo-cross-linking reaction, involving both the polymer and the drug.
Dextran was chosen as polymer because it is degradated by dextranases, enzymes present in the colon. The functionalization of dextran (DEX) with glycidyl methacrylate leads to the formation of a derivative (DEX-MA) that generates hydrogels for ultraviolet (UV) irradiation (Pitarresi et al. Citation2003). 2-phenylpropionic acid, chosen as model of the NSAIDs, was derivatized by reaction with 2-hydroxyethyl methacrylate. UV irradiation of solutions containing DEX-MA and the functionalized drug allowed the preparation of hydrogels containing the drug in the side chain. The release profile in conditions simulating the gastrointestinal tract showed that the drug was completely retained in the stomach, whereas it was slowly released in the colon, as a consequence of the action of dextranases and esterases. The amount of released drug was strictly related to the derivatization degree of the polymer as well as to its concentration in the solution to be irradiated. The same strategy was then applied successfully to 2-(4-isobutylphenyl)propionic acid (ibuprofen) and O-acetylsalicylic acid, two of the most used NSAIDs.
MATERIALS AND METHODS
All the reagents used were of analytical grade. Dextran (DEX) from Leuconostoc ssp. (Mr 40000), dimethylsulfoxide (DMSO), N,N-dimethylformamide (DMF), 4-dimethylaminopyridine (DMPA), 2-phenylpropionic acid, salicylic acid, O-acetylsalicylic acid, 2-(4-isobutylphenyl)propionic acid (ibuprofen), and N-(3-dimethylaminopropyl)-N′-ethyl carbodiimide hydrochloride were purchased from Fluka. Glycidyl methacrylate (GMA), 2-hydroxyethyl methacrylate, esterases (from porcine liver, 41 U/mg), and dextranases (from Pennicilium sp., 400/800 U/mg protein) were purchased from Sigma. Silica gel (70-230 mesh) was provided by Merck; 2H2O and 2HCCl3 came from Aldrich.
Synthesis of DEX-MA
Dextran methacrylate (DEX-MA) was synthesized as already reported (Pitarresi et al. Citation2003). To a solution of dextran (5.0 g) in DMSO (40 ml), DMAP (1.0 g) and GMA (0.73 g, 1 mol/6 mol of repetitive unit) were added. The solution was maintained under stirring at room temperature for 24 hr. EtOH (200 ml) was added dropwise; the precipitated solid was recovered by filtration, dissolved in water (15 ml), and the solution, adjusted to pH 8 with 1.0 M HCl, was submitted to exhaustive dialysis. After freeze-drying, the polymer was characterized by FT-IR, 13C-, and 1H-NMR. FT-IR spectra were recorded with a Perkin Elmer Paragon 1000 spectrophotometer in the range 4000–400 cm−1 using KBr pellets (resolution of 1 cm−1). 13C-and 1H-NMR spectra were obtained with a Bruker AC-400 instrument. The degree of derivatization (amount of methacryloyl groups for 100 dextran glucopyranose residues DD), calculated on the basis of the 1H-NMR spectrum as reported in the literature (Van Dijk-Wolthuis et al. Citation1997), was 20 ± 1. Employing different amounts of GMA (0.51 and 0.30 g, respectively), DEX-MA with DD = 14 ± 1 and 8 ± 1 also were synthesized.
Synthesis of DEX-MA Hydrogels in Different Solvents
UV irradiation was performed with a photochemical multirays reactor equipped with ten 12-watt medium pressure mercury lamps (λ maximum 313 nm). The photo-cross-linking reactions were carried out under nitrogen, on solutions of the polymer (7% w/v, DD = 14) in water, DMF, 50% water in DMF and DMSO, contained in quartz tubes of 1 cm diameter, with an irradiation time of 60 min. The gels were washed with water, submitted to exhaustive dialysis, and freeze-dried. All the experiments were carried out in triplicate. The yields of the hydrogels with the SD are reported in .
TABLE 1 Yield (%) of the hydrogels obtained after 60 min of irradiation (λmax = 313 nm) of 7% (w/v) solutions of dextran-methacrylate (derivatization degree = 14) in different solvents
Derivatization of Drugs with 2-Hydroxyethyl Methacrylate
To a solution of 2-phenylpropionic acid (1.32 g, 8.8 mmol) in DMF (45 ml), DMAP (0.12 g, 1 mmol) and N-(3-dimethyl-aminopropyl)-N′-ethyl carbodiimide hydrochloride (1.70 g, 8.8 mmol) were added successively. After complete dissolution, the mixture was cooled in an ice bath and 2-hydroxyethyl methacrylate (1.15 g, 8.8 mmol) was added. The solution was maintained under stirring at room temperature for 24 hr; DMF was distilled at 40°C under reduced pressure and the residue was recovered with CHCl3 (50 ml). The organic phase was washed with a saturated solution of NaHCO3 (2 × 20 ml), with H2O (2 × 20 ml) and dried on Na2SO4. The solvent was removed under reduced pressure; column chromatography of the residue (60 g of silica gel for 1 g of raw product) using CHCl3-AcOEt 9:1 as eluant gave methacryloyloxyethyl 2-phenylpropionate.
Applying the same procedure to ibuprofen and O-acetylsalicylic acid, the relative functionalized drugs were obtained. For these two derivatives column chromatography was carried out as described before, using a mixture of petroleum ether/acetone 4:1 as eluant. All the products were characterized by FT-IR, 1H-, and 13C-NMR.
Methacryloyloxyethyl 2-phenylpropionate. FT-IR (nujol): 1731, 1723, 1638 cm−1, 1H-NMR (2HCCl3) δ 1.54 (3H, d, J = 6.8, CH3), 1.92 (3H, s, CH3C═), 3.78 (1H, q, J = 6.8 Hz, CH), 4.3–4.4 (4H, m, OCH2CH2O), 5.57 (1H, d, J = 1.1 Hz, CH2═), 6.05 (1H, d, J = 1.1 Hz) and 7.30 ppm (5H, s, arom). 13C-NMR (2HCCl3). δ 18.4, (CH3C═), 18.5 (CH3), 45.6 (CH); 62.4–62.5 (CH2─CH2); 126.2 (C4); 127.3 (CH2═), 127.6 (C2 e C6), 127.8 (C3 e C5), 136.0 (C═CH2), 140.4 (C1), 167.2 (O═CC═) and 174.4 ppm (C═O).
Methacryloyloxyethyl 2-(4-isobutylphenyl)propionate. FT-IR (nujol): 1734, 1724, 1638 cm−1, 1H-NMR (2HCCl3) δ 0.89 (6H, d, J = 7.2 Hz, 2 × CH3), 1.45 (3H, d, J = 7.3, CH3), 1.86 (3H, s, CH3C═), 1.9–2.1 (1H, m, (CH3)2CH), 2.46 (2H, d, J = 7.2 Hz, CH2Ph), 3.77 (1H, q, J = 6.8 Hz, CH), 4.3–4.4 (4H, m, OCH2CH2O), 5.61 (1H, d, J = 1.2 Hz, CH2═), 5.99 (1H, d, J = 1.1 Hz. CH2═), 7.12 (2H, d, arom) and 7.23 ppm (2H, d, arom). 13C-NMR (2HCCl3). δ 18.4, (CH3C═), 18.5 (CH3), 22.6 (2 × CH3), 30.3 (CHCH2), 45.2 (CHPh and CH2PH), 62.4–62.5 (CH2─CH2), 126.2 (CH2═), 127.3 (C3 and C5), 129.5 (C2 and C6), 136.0 (C═CH2), 137.6 (C4), 140.7 (C1), 167.2 (O═CC═) and 174.4 ppm (C═O).
Methacryloyloxyethyl O-acetylsalicylate. FT-IR (nujol): 1745, 1722, 1638 cm−1, 1H-NMR (2HCCl3) δ 1.96 (3H, s, CH3C═), 2.34 (3H, s, CH3CO), 4.48 (2H, t, CH2O), 4.52 (2H, t, CH2O), 5.61 (1H, d, J = 1.2 Hz, CH2═), 6.15 (1H, d, J = 1.1 Hz. CH2═), 7.12 (1H, d, arom), 7.33 (1H, t, arom), 7.58 (1H, t, arom) and 8.04 ppm (1H, d, arom). 13C-NMR (2HCCl3). δ 18.4, (CH3C═), 21.1 (CH3CO), 62.5–60.0 (CH2─CH2); 123.0 (C3), 124.1 (C1), 126.2 (C5), 126.4 (CH2═), 132.0 (C6), 134.3 (C4), 136.0 (C═CH2), 140.4 (C2), 164.27 (C═O), 167.3 (O═CC═) and 169.8 ppm (CH3C═O).
Synthesis of Hydrogels with Drug in Side Chain and Entrapped in Network
To a solution (5, 7, 9% w/v) in DMF of DEX-MA having DD values of 8, 14, and 20, respectively, methacryloyloxyethyl 2-phenylpropionate (2.5% w/v) was added and the mixture was irradiated as already described. The irradiation time was 1 hr for the 9% and 7% w/v solutions and 3 hr for the 5% solution. The gels were purified through exhaustive dialysis and then freeze-dried. The yields of the gels with the respective SDs are reported in . The formation of the gel with the drug bound in side chain was confirmed by FT-IR spectra. Hydrogels having ibuprofen and O-acetylsalicylic acid in side chain were prepared starting from a solution at 2.5% w/v of the respective functionalized drug and 5% w/v of DEX-MA with DD = 8 in 5 ml of H2O/DMF (1:1). 2-Phenylpropionic acid (2.5% w/v) was added to aqueous solution of DEX-MA (5% w/v). The solution was irradiated for 3 hr and submitted to freeze-drying.
TABLE 2 Yields (%) of the hydrogels obtained in N,N-dimethylformamide from irradiation (λmax = 313 nm) of solutions of dextran-methacrylate at different derivatization degree and concentration (w/v%) in the presence of methacryloyloxyethyl 2-phenylpropionate (2.5% w/v)
Release Studies
First, 10 mg of freeze-dried gel were dispersed in 1 ml of 0.1 N HCl and kept at 37.0 ± 0.1°C under stirring (100 rpm). Second, 2 hr the dispersion was neutralized with 1 ml of 0.1 N NaOH and a solution of dextranases (250 μ l) at pH 6 was added (final enzyme concentration 12 U/ml). For the following 6 hr, at regular intervals of time, 250 μ l of esterases solutions (0.5 mg/ml concentration; pH 6) were added to the dispersion. At regular intervals of time, 20 μ l of the solution were withdrawn and analyzed by HPLC. HPLC apparatus consisted of a Perkin Elmer Series 200 LC pump, equipped with a 235 diode array. The analyses were carried out using a Merck Hibar LiChrocart (250-4, 5 μm) RP-18 column, with a flow of 1 ml/min, monitoring the released drug at λ = 220 nm for 2-phenylpropionic acid and O-acetylsalicylic acid and at λ = 215 nm for ibuprofen. A linear gradient from CH3CN/H3PO4 10− 2 M mixture (1:1) to pure CH3CN in 15 min was used as eluant for the analyses of 2-phenylpropionic acid and O-acetylsalicylic acid.
In the case of ibuprofen, a linear gradient from CH3CN/H3PO4 10−2 M mixture (7:3) to pure CH3CN always in 15 min was employed. After 24 hr of contact with the esterases, the pH was adjusted to a value of 12 with 0.1 N NaOH to obtain the complete destruction of the gel and calculate the real amount of drug present in the sample. All the experiments were carried out in triplicate and the results were in agreement within ±3% standard error.
RESULTS AND DISCUSSION
Previous studies have showed that dextran can be easily functionalized with glycidyl methacrylate to obtain a derivative (DEX-MA) able to give chemical hydrogels through UV irradiation (λ maximum 313 nm). The methacrylic groups (λmax for DEX-MA in water = 288 nm) are reactive enough to give rise, without initiators, to the network formation in aqueous solutions of the polymer at an opportune concentration (Pitarresi et al. Citation2001, Citation2003). Compared with other methods, this procedure allows the use of the hydrogels without purification processes that are necessary to remove solvents, catalysts, or initiators usually employed in the other syntheses. Furthermore, when the drug is added to the solution to be irradiated, the purification of the hydrogel cannot be carried out because it could remove the drug physically entrapped into the network. As a consequence, with slightly water-soluble drugs, only ethanol can be used to increase the drug solubility, and it is usually added in small amount to the aqueous solution to avoid the polymer precipitation (Giannuzzo et al. Citation2006).
We have planned to bind the drugs during the hydrogel formation through a reaction with their methacryloyl derivatives. These molecules are completely insoluble in water; DMF, DMSO, and their mixtures with water are able to solubilize both DEX-MA and drugs derivatives, but they are never used for this type of synthesis. For this reason, we started our investigation with a set of experiences of photo-cross-linking reactions carried out on 7% solutions of DEX-MA with DD = 14 in these solvents to verify if the hydrogel formation occurred. These conditions of concentration and DD value, already used in other studies (Pitarresi et al. Citation2003), assured a good yield for reactions carried out in water. The yields of the hydrogels, after exhaustive dialysis and freeze-drying, are reported in .
The comparison of the hydrogels yields shows that there are no significant differences so it is possible to carry out the photo-cross-linking in every one of these organic solvents. DMF or its mixtures with water were chosen for the successive syntheses. Of course the employment of such solvents needs a successive purification by dialysis, that is possible because the drug is chemically bound to the matrix.
Then we decided to verify the possibility to link the functionalized drug to the matrix during the photochemical reticulation. Maintaining a constant drug amount, different concentrations (5, 7, 9% w/v) of DEX-MA with DD = 14 were employed in the solution to be irradiated. The time of irradiation was 1 hr for the more concentrated solutions and 3 hr for the most dilute one. In solution at concentrations less than 5%, even prolonging the irradiation time, the formation of the gel did not take place. The yields of the gel, obtained from all the samples after dialysis and freeze-drying, are reported in .
It is evident that the yields (calculated with respect to both reagents) are high enough to assure the occurred formation of the covalent bond drug-polymer. It is likely that the reaction runs according to the mechanism schematized in . A confirmation that the formation of the bond has taken place is given by the comparison among the FT-IR spectra of DEX-MA, of the functionalized drug, and of the gel, reported in .
FIG. 1 Schematic representation of the formation of the hydrogel having the functionalized drug chemically bound.
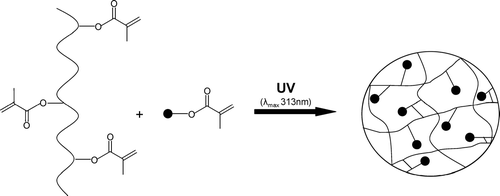
FIG. 2 FT-IR spectra of the functionalized drug (A), of DEX-MA (B), and of the corresponding gel (C).
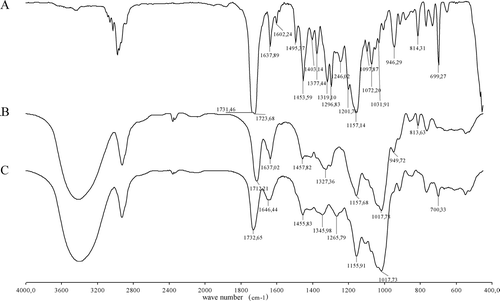
The disappearance of the peak at about 814 cm−1, characteristic of the C═C double bond stretching and present on both reagents, suggests that these groups have been involved in the formation of inter-and intrapolymeric chain bonds as well as of the polymer-drug ones. Moreover, the shift of the asymmetric stretching of the ester groups from 1712 and 1723 cm−1 (respectively in the polymer and the drug) to 1732 cm−1 (in the gel) is in agreement with the lack of conjugation due to the opening of the double bonds of the methacrylic residues of the starting molecules. Furthermore, the spectrum of the gel shows peaks that are characteristic of the two reagents. An example: the value of the ester peak in this network is at 1732 cm−1, whereas in DEX-MA hydrogels it is at 1720 cm−1 and confirms the presence of the linked drug.
Release studies of 2-phenylpropionic acid from the synthesized matrices were carried out in conditions simulating the gastrointestinal tract. A set of experiments was carried out on freeze-dried hydrogels deriving from solutions at different concentrations of DEX-MA with DD = 14. The first results showed that all the samples did not release the drug if maintained in solutions reproducing the gastric transit (pH 1.0 at 37.0 ± 0.1°C for 2 hr). In acid conditions the glycosidic bonds are stable and the networks do not undergo degradation. Furthermore the swelling degree of the matrix in acid solution is low. In fact Q = Ws/Wd (where Ws is the weight of the swelled network and Wd is the weight of the dry one) is equal to 6.2 in medium at pH 1.0 and 12.0 at pH 6.0. The poor swelling of the network protects probably the ester bonds by hydrolytic reactions, so that the drug is completely retained. The release studies had been continued in conditions simulating the intestinal tract (pH 6.0 solution in the presence of dextranases and esterases at 37.0 ± 0.1°C). The release profiles of the drug from matrices obtained from 5, 7, and 9% solutions of DEX-MA (DD = 14) are reported in .
FIG. 3 Release profiles [(Mt/M∞) × 100] of 2-phenyl propionic acid from the hydrogels obtained by irradiation of solutions at different concentrations of DEX-MA (DD = 14), maintained at 37.0 ± 0.1°C at pH 6.0 in the presence of dextranases and esterases.
![FIG. 3 Release profiles [(Mt/M∞) × 100] of 2-phenyl propionic acid from the hydrogels obtained by irradiation of solutions at different concentrations of DEX-MA (DD = 14), maintained at 37.0 ± 0.1°C at pH 6.0 in the presence of dextranases and esterases.](/cms/asset/945fd3f0-0261-415d-9200-7317543906e6/idrd_a_173963_uf0003_b.gif)
The percentage of released drug was calculated considering the total amount of bound drug (M∞), detected after basic hydrolysis of the matrix performed in solution at pH 12, for addition of an opportune amount of 1.0 M NaOH to each sample. The release profiles showed the same trend for the three hydrogels, even if the major amount of drug is released from the system obtained from the less concentrated solution.
As a consequence, the successive experiments were carried out employing matrices deriving from the irradiation of 5% solutions of DEX-MA. To verify the influence of the derivatization degree of the polymer on the drug release, we studied systems deriving from dextran with DD = 8, 14, and 20, respectively. The corresponding release profiles are reported in . Once again the maximum amount of acid is released by the system deriving from the less compact gel obtained from DEX-MA with DD = 8. These results are in agreement with the data present in the literature affirming that hydrogels, deriving from dextran with high substitution degree, undergo the action of enzymes in poor extension. In the same conditions, native dextran is easily and completely destroyed by enzymes action (Franssen et al. Citation1997, Citation1999). The comparison between the release profile of 2-phenylpropionic acid physically entrapped in DEX-MA hydrogel and that obtained when it is bound to the hydrogel is reported in . Both systems have been maintained in pH 1.0 solution for 2 hr and for further 6 hr in pH 6.0 solution in the presence of dextranases and esterases.
FIG. 4 Release profiles [(Mt/M∞) × 100] of 2-phenyl propionic acid from the hydrogels obtained by irradiation of 5% solutions of DEX-MA with different derivatization degree, maintained at 37.0 ± 0.1°C at pH 6.0 in the presence of dextranases and esterases.
![FIG. 4 Release profiles [(Mt/M∞) × 100] of 2-phenyl propionic acid from the hydrogels obtained by irradiation of 5% solutions of DEX-MA with different derivatization degree, maintained at 37.0 ± 0.1°C at pH 6.0 in the presence of dextranases and esterases.](/cms/asset/9301a8f4-98a5-4f32-a3ae-5f71ef46e072/idrd_a_173963_uf0004_b.gif)
FIG. 5 Release profiles [(Mt/M∞) × 100] of 2-phenyl propionic acid from the hydrogels having the drug bound to the matrix (▪ and physically entrapped (♦, maintained at 37.0 ± 0.1°C in HCl solution (pH 1.0) for 2 hr and at pH 6.0 in the presence of dextranases and esterases for further 6 hr.
![FIG. 5 Release profiles [(Mt/M∞) × 100] of 2-phenyl propionic acid from the hydrogels having the drug bound to the matrix (▪ and physically entrapped (♦, maintained at 37.0 ± 0.1°C in HCl solution (pH 1.0) for 2 hr and at pH 6.0 in the presence of dextranases and esterases for further 6 hr.](/cms/asset/1d47685b-23ba-44c4-a2f6-099a99019cfa/idrd_a_173963_uf0005_b.gif)
The improvement obtained with the new system is evident: the drug is exclusively released in the medium simulating the colon one, as a consequence of the action of the two enzymes. In particular, when only dextranases are present in the solution, the gel undergoes a complete degradation but only traces of drug are detected in solution. At the same way, the presence of esterases produces the release of less than 20% of the acid, because the molecules of drug that are entrapped inside the network cannot be reached by the enzymes. It is absolutely necessary the presence of dextranases that breaking the polymer in segments allows the action of esterases. As a consequence of these data, only a polymer like dextran, degraded by specific enzymes present in the colon, can be used to realize such a system.
Another advantage of the proposed approach concerns the total amount of drug released from the matrix. In the literature several papers report the synthesis of macromolecular prodrugs obtained by polymerization of mixtures of methacrylic acid and methacryloyloxyethyl derivatives of NSAIDs (Wang, Chiang, and Wu Citation2002; Chang et al. Citation1998; Davaran and Entezami Citation1997) employed for the controlled release of the latter. These systems are able to release not more than 35% of the drug bound to the polymer. It is likely that in this case the polymer, not fully degradated by specific enzymes, cannot release the most amount of the linked drug.
Following this optimized procedure, two other NSAIDs (ibuprofen and O-acetylsalicylic acid) have been bound to the hydrogel. The release profile of ibuprofen is reported in . The drug is completely retained in acid solution and slowly released in a neutral one. Analogue behavior is showed by O-acetylsalicylic acid whose release profile is reported in . The amount of released acid is the sum of O-acetylsalicylic and salicylic acid, because in such conditions the first is quickly converted into the second. The drug, retained in the stomach, is quantitatively released from the formulation in the successive 4 hr. These last results show that the proposed approach is suitable for drug having both aliphatic and aromatic carboxylic groups. The system is able to protect the gastric mucosa by the damaging action of the NSAIDs, and being degradable by dextranases (enzymes present in the colon) it can be proposed as a specific release method for drugs active toward colon diseases.
CONCLUSION
Hydrogels of dextran having a drug bound in side chain are suitable as colon-specific delivery systems. This target is achieved as a consequence of the high degradability of dextran, employed for the preparation of the hydrogels, in the intestine where dextranases are present. The amount of released drug is critically related to the derivatization degree of the functionalized polymer as well as to the concentration of the latter in the solution submitted to irradiation. In fact the best performance is reached using poor compact gels that are fully degraded by the action of dextranases. After the optimization of the procedure, carried out on a model drug such as 2-phenylpropionic acid, it has been extended to other two NSAIDs with very good results.
This work was carried out with the financial support of MIUR.
REFERENCES
- Arica B., Calis S., Durlu N. T., Kakar N., Hincal A. A. In vitro and in vivo studies of ibuprofen-loaded biodegradable alginate beads. J. Microencapsul. 2005; 22: 153–165
- Chang C. H., Sheu Y. M., Hu W. P., Wang L. F., Chen J. S. Synthesis and properties of copolymers from 2-hydroxyethyl methacrylate-linked non steroidal antiinflammatory agents with methacrylic acid. J. Polym. Sci. Polym. Chem. 1998; 36: 1481–1490
- Davaran S., Entezami A. A. Acrylic type polymers containing ibuprofen and indomethacin with difunctional spacer group: synthesis and hydrolysis. J. Control. Rel. 1997; 47: 41–49
- Franssen O., Van Ooijen R. D., De Boer D., Maes R. A. A., Hennink W. E. Enzymatic degradation of cross-linked dextrans. Macromolecules 1999; 32: 2896–2902
- Franssen O., Van Oojien R. D., De Boer D., Maes R. A. A., Herron J. N., Hennink W. E. Enzymatic degradation of methacrylated dextrans. Macromolecules 1997; 30: 7408–7413
- Freitas M. N., Marchetti J. M. Nimesulide PLA microspheres as a potential sustained release system for the treatment of inflammatory diseases. Int. J. Pharm. 2005; 295: 201–211
- Giannuzzo M., Feeney M., Paolicelli P., Casadei M. A. Synthesis and characterization of pH-sensitive hydrogels of dextran. J. Drug Del. Sci. Tech. 2006; 1: 49–54
- Kamath K. R., Park K. Biodegradable hydrogels in drug delivery. Adv. Drug Delivery Rev. 1993; 11: 59–84
- Landgraf W., Li N. H., Benson J. R. New polymer enables near zero-order release of drugs. Drug Del. Techn. 2005; 48: 50–55
- Langman J. S. Adverse effects of conventional non-steroidal anti-inflammatory drugs on the upper gastrointestinal tract. Fund. Clin. Pharmacol. 2003; 17: 393–403
- Mandracchia D., Pitarresi G., Palumbo F. S., Carlini B., Giammona G. pH-Sensitive hydrogel based on a novel photocross-linkable copolymer. Biopolymers 2004; 5: 1973–1982
- Peppas N. A., Bures P., Leobandung W., Ichikawa H. Hydrogels in pharmaceutical formulations. Eur. J. Pharm. Biopharm. 2000; 50: 27–46
- Pitarresi G., Palumbo F. S., Giammona G., Casadei M. A., Micheletti Moracci F. Biodegradable hydrogels obtained by photocrosslinking of dextran and polyaspartamide derivatives. Biomaterials 2003; 24: 4301–4313
- Pitarresi G., Craparo E. F., Carlisi B., Giammona G., Buscemi S. Ultraviolet-induced cross-linking of a functionalized polyaspartamide carrying ester groups. J. Bioactive Comp. Polym. 2001; 16: 98–118
- Van Dijk-Wolthuis W. N. E., Ketteness-Van Den Bosch J. J., Van Der Kerk-Van Hoof A., Hennink W. E. Reaction of dextran with glycidyl methacrylate: an unexpected transesterification. Macromolecules 1997; 30: 3411–3413
- Wang L. F., Chiang H. N., Wu P. C. Kinetics and hydrolysis mechanism of polymeric prodrugs containing ibuprofen, ketoprofen, and naproxen as pendent agents. J. Biomater. Sci. Polymer Edn. 2002; 13: 287–299