Abstract
We aimed to prepare and investigate microparticles with the varying contents of calcium gelling ion, loaded with phenytoin, a standard antiepileptic agent, in its acidic form. Two different methods of alginate-based microparticles preparation were used: with and without treatment with chitosan. Furthermore, two standard procedures, the one-stage and the two-stage, were applied. Microparticle size of 12 one-stage formulations ranged from 466 to 636 μm. Both types of formulations, chitosan-treated and nontreated, appeared to be highly loaded with the model drug (91–96%). The chitosan-coated alginate-based microparticles prepared by the one-stage procedure exhibited kinetics of phenytoin liberation comparable to a similar sustained release system that had been tested at pH 6.8, as published earlier. As the gel erosion of alginate-based microparticles should be potentiated by the higher pH (used in the present study at pH 7.4), the most favorable of 12 formulations, with the liberation half-time of about 2 hr, seemed to be eligible for further modifications. Counterintuitively, the applied two-stage procedure did not appear to beneficially affect the dissolution behavior of phenytoin when tested in two formulations, which makes further modifications necessary.
The alginate-based microparticles have been widely investigated in a large array of applications, such as enzyme immobilization, immunoisolation in cell transplantation, and drug release systems (Gaserod, Sannes, and Skjak-Braek Citation1999). Such versatility is based on natural source, easy handling, and biocompatibility of alginates (Buthe, Hartmeier, and Ansorge-Schumacher Citation2004). Alginate is a family of polysaccharides composed of α -L-guluronic acid (G) and β -D-mannuronic acid (M) residues, arranged in homopolymeric (MM, GG) as well as heteropolymeric blocks (MG). It has been well established that the monomer composition affects drug release from the different formulation systems (Takka and Acarturk Citation1999; McDowell Citation1986). Alginates with high mannuronic acid content are appropriate for thickening applications, whereas alginates with a high level of guluronic acid are the best for forming gels (Takka and Acarturk Citation1999).
Concerning the sustained release systems, gel erosion of alginate-based microparticles due to presence of chelators such as phosphate, lactate, or citrate or nongelling cations like sodium or magnesium, imposes a significant problem, since it accelerates the release of a drug. Therefore, there is need for a stabilizing agent that can be a membrane forming polycation bound to the alginate gel by electrostatic interactions. One such agent is chitosan, a natural linear biopolyaminosaccharide, obtained by alkaline deacetylation of chitin (Roberts Citation1992). Chitin is a straight homopolymer composed of β -(1,4)-linked N-acetyl-glucosamine units, whereas chitosan comprises a series of copolymers of glucosamine and N-acetyl-glucosamine (Kas Citation1997; Singla and Chawla Citation2001; Kato Citation2003). Chitosan has one primary amino and two free hydroxyl groups for each C6 building unit. Due to the easy availability of free amino groups in chitosan, it carries a positive charge and thus reacts with different negatively charged surfaces and polymers (Sinha et al. Citation2004).
There are numerous variations in procedures and materials applied during the production of microparticles with a complex coacervate alginate-chitosan membrane and a solid alginate gel core. However, the two principal modes are one-stage and two-stage procedures. In the one-stage procedure, a complex coacervate membrane is formed at the interface between the alginate and chitosan solutions when the alginate solution is dropped directly into the solution of chitosan. This yields particles with a complex alginate-chitosan membrane surrounding a liquid alginate core. Gelling of the core is achieved by having calcium chloride in chitosan solution either during the formation of the particles (Huguet, Neufeld, and Dellacherie Citation1996; Huguet et al. Citation1994; Lee, Park, and Ha Citation1997; Overgaard et al. Citation1991) or by treating the liquid core particles with the salt after the membrane has been formed (Huguet et al. Citation1994).
The two-stage procedure comprises formation of calcium alginate beads, followed by a membrane forming stage when the beads are suspended in a solution of polycation (Lim and Sun Citation1980). The amount of chitosan bound to the alginate beads depends on several factors (Gasserod, Smidsrod, and Skjak-Braek Citation1998). First, the choice of producing method clearly limits the amount of bound chitosan. If the capsules are made by a one-stage procedure, only low amounts of chitosan could be bound. This is probably because chitosan binds to the surface only and creates a membrane with such small pores they prevent further diffusion of chitosan into the beads, and subsequent binding onto the gel network is restricted. In the two-stage procedure, more chitosan can be bound both onto the surface of the bead and in the interior of the gel network while suspending calcium alginate beads in a solution of chitosan. Hence, the two-stage procedure may result in binding 100 times more chitosan than with the one-stage procedure. These high chitosan contents are only obtained for the most porous gels with the chitosan of average molecular weight less than 20,000.
High porosity is achieved by selecting an alginate with a high ratio of α -L-guluronic acid to β -D-mannuronic acid residues, by including gelling calcium ions in the chitosan solution, and/or by making the beads homogeneous (Gasserod, Smidsrod, and Skjak-Braek Citation1998). Homogeneity here refers to the alginate concentration gradient through the cross-section of the bead. In an inhomogeneous bead, the alginate concentration at the bead surface may be 10 times higher than in the center (Skjak-Braek, Grasdalen, and Smidsrod Citation1989).
The objective of the study was to prepare and evaluate microparticles with varying contents of calcium gelling ion and chitosan, loaded with phenytoin in its acidic form, to improve drug release. The model substance phenytoin has been well established as a standard antiepileptic agent. The extended-release formulations of antiepileptics decrease daily fluctuations and simplify treatment of this chronic condition. Such commercial formulations with phenytoin sodium appear to be well designed, with one possible shortcoming. That concern deals with the potential for irregular absorption that appears to occur particularly in the case of elderly patients (Pellock et al. Citation2004). Besides the wanted well-controlled release, an additional benefit of the present investigation might be manufacturing in environmentally friendly conditions.
MATERIALS AND METHODS
Manugel GHB, medium viscosity sodium alginate with high guluronic acid (“high G”) content was kindly donated by ISP (International Specialty Products, NJ, USA). Phenytoin was provided by Fluka (Neu-Ulm, Germany). Chitosan, high and low molecular weight (degree of deacetylation, > 75% and 75–85%, respectively), was purchased from Aldrich (Milwaukee, WI, USA). Calcium chloride was obtained from Merck KGaA (Darmstadt, Germany).
Microparticles Preparation
Microparticles were prepared according to the one-stage procedure using a custom made air-jet device. Approximately 7 ml of 2% phenytoin suspension (bubble free) in 2% aqueous solution of alginate (drug:polymer ratio = 1:1) was extruded through a 20-gauge needle at a rate of 120 ml/hr into 70 ml of a gelling medium that consisted of aqueous solution of different concentrations of calcium chloride and chitosan. pH value of gelling medium was adjusted to 5.5 with acetate buffer. The laminar air flow in coaxial cylinder, pointed to the tip of the needle producing small droplets, was constant (1200 l/hr). The prepared microparticles were allowed to harden for 1 hr, after which they were collected, washed, and dried at room temperature for 72 hr. The 12 different formulations (F1–F12) of inhomogeneous microparticles were made according to this procedure ().
TABLE 1 Composition of model formulations of inhomogeneous microparticles prepared according to the one-stage procedure
In case of the two-stage procedure, after 30 min of hardening of the microparticles in gelling medium with different concentrations of calcium chloride, they were transferred without washing to a solution of 0.2% (w/w) chitosan (pH adjusted to pH 5.5 with acetate buffer). Chitosan solutions also contained 0.3M of calcium chloride. According to this procedure, two additional formulations, a homogeneous one with low molecular weight chitosan (F7a) and an inhomogeneous one with high molecular weight chitosan (F7b), were prepared. Homogeneity of the microparticles was achieved by including 200 mM of NaCl in the gelling medium (Skjak-Braek et al. Citation1989).
Microparticles Size Determination
The particle sizes of 50 microparticles were measured with Motic digital microscope DMB3-223ASC and Motic Images Plus v.2.0 software for each formulation and the mean particle size was determined.
Drug Assay (Drug Loading Determination)
Determination of phenytoin in microparticles was performed using RP-HPLC method after microparticles were broken with the aid of ultrasonication in 0,1 M NaOH for 15 min, with subsequent centrifugation at 4000 rpm for 5 min. Phenobarbitone was used as an internal standard. The column used was Zorbax SB-C18, 250 × 4, 6 mm, 5 μ m. Composition of mobile phase was ethanol:water:0,5% triethanolamine in ratio 40:59,5:0,5 (v/v/v), respectively. pH of mobile phase was adjusted to 3.0 with 85% ortophosphoric acid. Chromatography was conducted at ambient temperature at a flow rate of 1.0 ml/min. The absorbance of each sample was determined spectrophotometrically at 228 nm using Agilent 1100 Series variable wavelength ultraviolet detector. All determination tests were performed in triplicate.
Drug Release Studies
The release of phenytoin from microparticles was measured quantitatively on an automatic sampling and analysis system Erweka DT 800 (Erweka GmbH, Heusenstamm, Germany) using the rotating basket method at 100 rpm with the temperature maintained at 37°C, in 900 ml phosphate buffer (pH 7,4 USP23). The obtained samples were mixed with solution of internal standard and phenytoin content was determined as explained under Drug Assay section. All release tests were performed in triplicate.
RESULTS AND DISCUSSION
Microparticles Size Determination
Recorded microparticle sizes were in the range of 466.2 ± 14.8 to 635.5 ± 6.2 μ m. Although statistical analysis (not shown) pointed to some inhomogeneity in mean diameters of microparticles, the range of obtained values does not suggest reliable influence of chitosan on microparticle size (), which is in good accord with the results described previously (Takka and Acarturk Citation1999).
However, far different from alginate-based formulations (), presence of chitosan tended to distort the spherical shape of microparticles during both the manufacturing and the drying phase (). This observation was not at all unexpected (Shu and Zhu Citation2002). In the one-stage procedure, with chitosan of high molecular weight present in the gelling medium, it has been observed that droplets of alginate are not able to break easily through the surface (Gaserod et al. Citation1999). This may explain in part the nonspherical shape of microparticles of formulations with higher chitosan concentration. Moreover, the spherical shape of alginate and alginate-chitosan microparticles (up to 0.2% of chitosan) in the wet state was usually lost after drying, especially for those prepared with low alginate concentration (Shu and Zhu Citation2002). This indicates that the integrity of alginate microparticles, as well as integrity of alginate-chitosan membrane, may be compromised in the drying process. Finally, a trend of improving the sphericity of dried microparticles with the increase of alginate concentration also has been noticed (pictures not shown).
Drug Assay
Both types of formulations, chitosan-treated and nontreated, appeared to be highly loaded with the model drug (). The drug contents of the dried microparticles were determined to be 90.63–96.34%. No clear relationship between the drug loading and concentration of calcium chloride and chitosan was found, neither was any influence of mere chitosan presence detected.
TABLE 2 Drug loading of 12 (F1–F12) studied model formulations
Furthermore, it appeared that the recorded morphological changes did not affect encapsulation efficiency. Measuring the phenytoin particles in alginate suspension showed that average particle perimeter was 44.0 μ m (). Along with its poor solubility in aqueous media, such a high perimeter probably prevented exit of phenytoin through the pores of instantaneously formed microparticles. This may be a part of explanation for such a high loading capacity.
Drug Release Studies
depicts the phenytoin release profiles from all alginate and alginate-chitosan microparticle formulations manufactured in the one-stage procedure. The times for 50% and 90% of drug to be released (t50% and t90%, respectively), as the parameters suitable for comparing the different formulations (Takka and Acarturk Citation1999; Sankalia et al. Citation2005), are shown in . We found that the influence of chitosan coating on phenytoin release cannot be clearly deduced.
TABLE 3 Times for 50% and 90% of the drug to be released, t50% and t90%, respectively
It has been reported that the alginates rich in guluronic acid form more rigid gels, which are less prone to erosion and the drug release would be slower. Murata et al. (Citation1993) found that the release of drug from M-rich alginate gels was slightly faster than that from G-rich alginate gels, despite similarity in their molecular weights. The gelation takes place by forming egg-box junctions to associate the metal ions with the GG blocks of the alginate chain (McDowell Citation1986; Tonessen and Karslen Citation2002). Being a polyelectrolyte, alginate can exhibit swelling properties that are sensitive to pH, ionic strength, and specific ionic composition of the medium (Ostberg, Lund, and Graffner Citation1994). These factors also may influence the release properties of alginate-based formulations.
Also, the polyion complexation between chitosan and anionic polymers depends on the pH value of the media, the ionic strength, and temperature (Takahashi et al. Citation1990). Polk et al. (Citation1994) investigated the effect of the pH of gelling medium containing chitosan on microparticle strength and flexibility and reported that the strength and flexibility of the microparticle membrane were maximal as the pH of the chitosan solution increases to 5.5. Furthermore, for drugs of poor water solubility encapsulated into alginate-chitosan microparticles, molecular weight and viscosity of alginates should not affect the drug release (Takka and Acarturk Citation1999).
Taking into consideration these suggestions, 3 formulations were prepared (F1 through F3) using different concentrations of calcium chloride and alginate of medium viscosity characterized with high G content and 9 formulations (F4 through F12) with different concentrations of chitosan and calcium chloride using the same type of alginate. In addition, the pH of all gelling solutions was adjusted to pH 5.5.
In general, the addition of a given concentration of chitosan tended to delay the release of drug from the high G alginate-made microparticles (). The t50% value of F1 formulation, prepared only with 0,5% of calcium chloride present in the gelling medium, was 73 min, whereas t50% value of F7 formulation, prepared with 0,5% of calcium chloride and 0,2% of chitosan in gelling medium was 115 min.
FIG. 6 (a) Release profiles of phenytoin from alginate and alginate-chitosan microparticles. (b) Effect of chitosan concentration on release characteristics of alginate-chitosan microparticles. (c) Effect of calcium chloride concentration on release characteristics of alginate and alginate-chitosan microparticles.
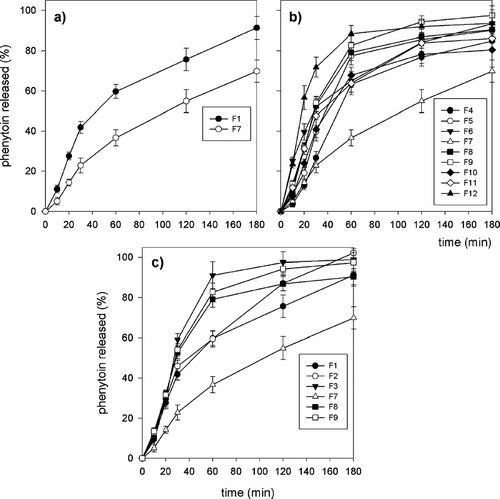
For the given concentrations of calcium chloride present in the gelling medium, phenytoin release from alginate-chitosan microparticles decreased with the addition of chitosan to the gelling medium, especially for 0.2% of chitosan (). Similar results, for small molecular weight drugs of poor solubility in aqueous media, macromolecular drugs, and small molecular weight drugs with high solubility, were reported earlier (Takka and Acarturk Citation1999; Sezer and Akbuga Citation1999a, Citation1999b).
We observed, unexpectedly, that the increase of chitosan concentration over 0.1% tended to accelerate phenytoin release, except for formulation F7, prepared with 0.5% calcium chloride and 0.2% chitosan present in the gelling medium. For this formulation, a distinct delay in release of phenytoin from microparticles, compared with all other formulations, was observed (). In some cases, chitosan coating markedly accelerated phenytoin release compared with microparticles prepared only from alginate. For example, t50% values for formulation F3 prepared with 2.0% of calcium chloride and with no chitosan present in the gelling medium was 51 min, compared with formulation F12, prepared with 2.0% of calcium chloride and 0.4% of chitosan, with t50% of 34 min.
However, the difference between t90% values of these formulations was not so distinct. The similar phenomena were reported earlier for a water soluble dye, brilliant blue, where release media was 0.9% sodium chloride and 0.1 M phosphate buffer as well, especially in the case of dried microparticles and in some cases of nondried microparticles, made in single stage procedure. The authors attributed these phenomena to destroying the integrity of chitosan-alginate complex membrane during the drying process (Shu and Zhu Citation2002).
The influence of calcium chloride concentration on phenytoin release also was examined (). The t50% and t90%values of formulations F1–F3 made with 0.5%, 1.0%, and 2.0% of calcium chloride present in gelling medium were 73, 64, 51 and 158, 138, and 125 min, respectively. They found that an increased drug release occurs while increasing calcium chloride concentration. Similar data were reported earlier (Sezer and Akbuga Citation1999a).
To further improve the liberation profile of phenytoin, two different modifications of the formulation with best kinetics of drug release, F7, were explored. Namely, we showed that integrity of microparticles in solutions against osmotic pressure and sequestrants could be significantly improved if more chitosan is bound to the alginate. This could be achieved if microparticles are made according to the two-stage procedure, with addition of calcium ions to the gelling medium along with chitosan and by making them homogeneous (Gaserod et al. Citation1999). Based on this procedure, the first modification, F7a, was made. Furthermore, it has been postulated that, on swelling in dissolution medium, the integrity of microparticles would be maintained with the pores small enough to keep the relatively large particles of phenytoin inside, thereby further slowing its release.
The second modification F7b was made having in mind that permeability of microparticles can be reduced by preparing them to be inhomogeneous, also according to the two-stage procedure, but with high molecular weight chitosan (Gaserod et al. Citation1999). The rationale for this second approach is that microparticles with improved stability and reduced permeability further slow down the release of drug in dissolution medium.
shows the release profile of phenytoin from F7a and F7b formulations compared with basic formula F7 and to F1, made only with calcium chloride and with no chitosan present in gelling medium. It can be seen that both modifications actually further increase the release of phenytoin, with increase that is more pronounced for formulation F7b. This was not totally unexpected since Shu and Zhu (Citation2002) showed that chitosan coating accelerated release of the water soluble dye brilliant blue in 0.10 M phosphate buffer from nondried microparticles, compared with those made only with alginate, especially when the single stage procedure was used. Here, in contrast with findings by Shu and Zhu (Citation2002), the microparticles prepared according to the single-stage procedure exhibited more favorable release profile in phosphate buffer compared with formulations F7a and F7b, prepared according to the two-stage procedure. At least partly, this may be seen as an unfavorable influence of the drying process on susceptibility of the microparticles prepared according to the two-stage procedure to the phosphate buffer. Alternatively, the difference in the model drug or preparation procedure used may explain in part this discordance. For instance, as a consequence of different manufacturing devices employed, mean particle sizes were 6-fold greater in Shu and Zhu (Citation2002) than in our experiment. Also, concentrations of calcium chloride in chitosan solutions were different, whereas NaCl (added to prepare homogeneous microparticles) was present only in our gelling medium.
CONCLUSION
The performed one-stage procedure yielded particles of nonspherical shape on drying process, with the sphericity improving with the increase of alginate concentration, without reliable influence of chitosan on microparticle size. All studied formulations were of very high model drug loading, with no influence of the calcium chloride or chitosan concentration on this parameter.
Increasing calcium chloride resulted in enhanced phenytoin release, whereas the influence of chitosan on the model drug release from particles made according to the one-stage procedure was complex and not so clear-cut. In some cases, chitosan coating markedly accelerated phenytoin release compared with the nontreated microparticles, which could be attributed partly to destroying the integrity of chitosan-alginate complex surface layer during the drying process. In other cases, the release of phenytoin held within the high G alginate-made chitosan-treated microparticles incubated in phosphate buffer was delayed compared with the nontreated ones, presumably due to the suppressed erosion of chitosan-reinforced calcium-induced alginate gel network. The delay was marked in the case of formulation F7, with the t50% value of 115 min, making it eligible for further modifications. However, modifications of F7 formulation according to the two-stage procedure did not appear to beneficially affect the release behavior of phenytoin, and further modifications are needed.
This work was done under the project 142063G supported by the Ministry of Science and Environmental Protection, Republic of Serbia. The authors thank Živorad Denić (DCP Hemigal) for financial support.
REFERENCES
- Buthe A., Hartmeier W., Ansorge-Schumacher M. B. Novel solvent-based method for preparation of alginate beads with improved roundness and predictable size. J. Microencapsul. 2004; 21: 865–876
- Gaserod O., Sannes A., Skjak-Braek G. Microcapsules of alginate-chitosan. II. A study of capsule stability and permeability. Biomaterials 1999; 20: 773–783
- Gasserod O., Smidsrod O., Skjak-Braek G. Microcapsules of alginate-chitosan. I. A quantitative study of the interaction between alginate and chitosan. Biomaterials 1998; 19: 1815–1825
- Huguet M. L., Neufeld R. J., Dellacherie E. Calcium-alginate beads coated with polycationic polymers: comparison of chitosan and DEAE-dextran. Process Biochem. 1996; 39: 347–353
- Huguet M. L., Groboillot A., Neufeldt R. J., Poncelet D., Dellacherie E. Haemoglobin encapsulation in chitosan/calcium alginate beads. J. Appl. Polym. Sci. 1994; 51: 1427–1432
- Kas H. S. Chitosan: properties, preparation and application to microparticulate systems. J. Microencapsul. 1997; 14: 689–711
- Kato Y., Onishi H., Machida Y. Application of chitin and chitosan derivatives in the pharmaceutical field. Curr. Pharm. Biotechn. 2003; 14: 303–309
- Lee K. Y., Park W. H., Ha W. S. Polyelectrolyte complexes of sodium alginate with chitosan or its derivatives for microcapsules. J. Appl. Polym. Sci. 1997; 63: 425–432
- Lim F., Sun A. M. Microencapsulated islets as bioartificial endocrine pancreas. Science 1980; 210: 908–910
- McDowell R. H. Properties of Alginates, 5th ed. Kelco International, London 1986
- Murata Y., Nakada K., Miyamoto E., Kawashima S., Seo S. H. Influence of erosion of calcium-induced alginate gel matrix on the release of brilliant blue. J. Control. Rel. 1993; 23: 21–26
- Ostberg T., Lund E. M., Graffner C. Calcium alginate matrices for oral multiple unit administration: IV. Release characteristics in different media. Int. J. Pharm. 1994; 112: 241–248
- Overgaard S., Scharer J. M., Moo-Young M., Bols N. C. Immobilization of hybridoma cells in chitosan alginate beads. Can. J. Chem. Eng. 1991; 69: 439–443
- Pellock J. M., Smith M. C., Cloyd J. C., Uthman B., Wilder B. J. Extended-release formulations: simplifying strategies in the management of antiepileptic drug therapy. Epilepsy Behav. 2004; 5: 301–307
- Polk A., Amsden B., De Yao K., Peng T., Goosena A. Controlled release of albumin from chitosan-alginate microcapsules. J. Pharm. Sci. 1994; 83: 178–185
- Roberts G. A. F. Introduction of chitin and chitosan. Chitin Chemistry, G. A. F. Roberts. The Macmillan Press Ltd., London 1992; 1–49
- Sankalia M. G., Mashru R. C., Sankalia J. M., Sutariya V. B. Papain entrapment in alginate beads for stability improvement and site-specific delivery: physicochemical characterization and factorial optimization using neural network, modeling. AAPS Pharm. Sci. Tech. 2005; 6: 209–222
- Sezer A. D., Akbuga J. Release characteristics of chitosan treated alginate beads: I. Sustained release of a macromolecular drug from chitosan treated alginate beads. J. Microencapsul 1999a; 16: 195–203
- Sezer A. D., Akbuga J. Release characteristics of chitosan treated alginate beads: II. Sustained release of a low molecular drug from chitosan treated alginate beads. J. Microencapsul 1999b; 16: 687–696
- Singla A. K., Chawla M. Chitosan: some pharmaceutical and biological aspects—an update. J. Pharm. Pharmacol. 2001; 53: 1047–1067
- Sinha V. R., Singla A. K., Wadhawan S., Kaushik R., Kumria R., Bansal K., Dhawan S. Chitosan microspheres as a potential carrier for drugs. Int. J. Pharm. 2004; 274: 1–33
- Shu X. Z., Zhu K. J. The release behavior of brilliant blue from calcium-alginate gel beads coated by chitosan: the preparation method effect. Eur. J. Pharm. Biopharm. 2002; 53: 193–201
- Skjak-Braek G., Grasdalen H., Smidsrod O. Inhomogeneous polysaccharide ionic gels. Carbohydr. Polym. 1989; 10: 31–54
- Takahashi T., Takayama K., Machida Y., Nagai T. Characterisics of polyion complexes of chitosan with sodium alginate and sodium polyacrylate. Int. J. Pharm. 1990; 61: 35–41
- Takka S., Acarturk F. Calcium alginate microparticles for oral aministration: I Effect of sodium alginate type on drug release and drug entrapment efficiency. J. Microencapsul. 1999; 16: 275–290
- Tonessen H. H., Karlsen J. Alginate in drug delivery systems. Drug Dev. Ind. Pharm. 2002; 28: 621–630