Abstract
Two novel cholesterol-based cytofectins containing primary amino head groups, glycylcholesteryl formylhydrazide (MS10) and β-alanylcholesterylformylhydrazide (MS11), have been prepared and incorporated into unilamellar cationic liposomes in equimolar amounts with dioleoylphosphatidylethanolamine (DOPE) as colipid. Stable lipoplexes were formed with pGL3 DNA which afforded protection to the DNA from serum nuclease digestion. Packing of the DNA was shown by ethidium displacement to be more effective in MS11 lipoplexes. High transfection levels in three human transformed epithelial cell lines HeLa (cervical), SNO (esophageal), HepG2 (hepatocyte-derived), and the murine fibroblast line NIH-3T3 were achieved by both lipoplexes at liposome:DNA ratios of 6:1 and 7:1 (w/w) corresponding to +/− charge ratios of 1.6:1 and 1.9:1. MS11 lipoplexes, in particular, afforded high transfection activities in the presence of fetal bovine serum.
As more and more disease conditions are being ascribed to genetic malfunctions so too more gene-based modalities are emerging for their treatment. Thus the insertion of corrective DNA into genetically compromised cells of whole organisms and its subsequent controlled expression therein is an attractive prospect. Since the first gene therapy trial for the treatment of adenosine deaminase (ADA) deficiency (Blaese, Culver, and Anderson Citation1990) in a growing number of conditions this has been demonstrated to be a viable approach. While viral DNA delivery systems are being actively developed, attendant safety and production difficulties have impacted negatively on their application (Yi and Lee Citation2005). However, a number of nonviral gene delivery systems suitable for in vitro and in vivo application have been proposed.
Cohesion between the DNA cargo and the nonviral vehicles is provided initially by the attraction between cationic (basic) centers on the vehicles and nucleic acid phosphate residues. Among these, the two main groups comprise first, polymer-based delivery vehicles, which include polyethyleneimine (Bousiff et al. Citation1995), poly-L-lysine (Wu and Wu Citation1987), polybrene (Mumper et al. Citation1996), and secondly, chitosan (Corsi et al. Citation2003) and cationic liposomes. In this latter group, the cationic head group is linked via a spacer to membrane bilayer-embedded anchors. These components frequently comprise two hydrophobic long chain unsaturated acyl groups such as are found in DOTAP (Felgner et al. Citation1987) and DOSPER (Buchberger et al. Citation1996). But a separate but sizable class of cytofectins relies on the cholesteryl moiety to secure cationic head groups to liposomal membranes as exemplified by the widely adopted DC-Chol (Gao and Huang Citation1991). Cationic head groups secured to this anchor are believed to be more active than those linked to diacyl chains (Marshall et al. Citation1999). Here, the cationic centers are most commonly tertiary (Gao and Huang Citation1991; Takeuchi et al. Citation1996) and quaternary amines (Kisoon, Ariatti and Moodley Citation2002; Reynier et al. Citation2004).
However tertiary amines are frequently less basic than their primary amine counterparts as seen with methylamines and ethylamines (Sykes Citation1970). Primary amine-containing cytofectins therefore may exhibit significant DNA binding activity. Whereas this is true of polycations such as poly-L-lysine and poly-L-ornithine with ε and δ primary amino groups (Wu and Wu Citation1988; Dong, Skoultchi, and Pollard Citation1993, respectively), the same has not been firmly established for monocationic amphiphiles. To explore this possibility we decided to synthesize two cytofectins embodying a cholesteryl hydrophobic anchor, a polar spacer arm, and primary amino cationic center: glycylcholesterylformylhydrazide (MS10) and β -alanylcholesterylformylhydrazide (MS11) (). These derivatives were formulated into stable unilamellar liposomes with equimolar amounts of dioleoylphosphatidylethanolamine (DOPE) as colipid.
FIG. 1 Synthesis of glycylcholesterylformylhydrazide (MS10) (n = 1) and β -alanylcholesterylformylhydrazide (MS11) (n = 2) from cholesterylformylhydrazide (a). i = NHS esters of N-trityl amino acids; ii = AcOH/H2O.
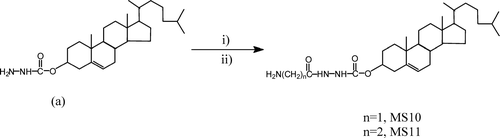
Lipoplexes formed with plasmid DNA pGL3 at positive/negative charge ratios of 1.6:1 and 1.9:1 exhibited strong resistance to serum nucleases and low toxicity in a selection of transformed human epithelial cell lines (SNO, HepG2, HeLa) and the murine fibroblast cell line NIH3T3. Transfection levels were uniformly high for both lipoplexes in the four cell lines suggesting that MS10 and MS11, when formulated into liposomes, may indeed be versatile transfecting agents with broad applicability.
MATERIALS AND METHODS
Cholesteryl chloroformate, N-trityl glycine, β -alanine, N-hydroxysuccinimide (NHS), trityl chloride, dicyclohexylcarbodiimide (DCC), dioleoylphosphatidylethanolamine (DOPE), and 2-[-(2-hydroxyethyl)-piperazinyl]-ethanesulfonic acid (HEPES) came from Sigma-Aldrich (St Louis, MO, USA). Solvents, silica gel 60F254 chromatography plates, glutaraldehyde, OsO4, propylene oxide, and lead citrate were purchased from Merck (Darmstadt, Germany). Ultrapure DNA-grade agarose was supplied by BioRad (Richmond, CA, USA). The components of Spurr's resin (GRL4206, DER736, NSA, S-1) were purchased from TAAB Laboratories (UK). pBR322 DNA was from Roche (Mannheim, Germany) and pGL3 control vector and luciferase assay kit were from Promega Corporation (Madison, WI, USA). Trypsin-EDTA and penicillin-streptomycin mixtures were supplied by Whittaker Bioproducts (Walkerville, MD, USA). Minimum essential medium (MEM) with Earle's salts was bought from GIBCO BRL (Inchinnan, Scotland).
Plasticware was obtained from Bibby-Sterilin (Staffordshire, England). N-trityl β -alanine and the NHS esters of N-trityl glycine and N-trityl β -alanine were prepared by standard procedures described elsewhere (Ariatti and Hawtrey Citation1975). Cholesterylformylhydrazide was synthesized from cholesteryl chloroformate and hydrazine as described previously (Singh and Ariatti Citation2006). Ultrapure water (Milli-Q50) was used throughout this study.
Spectral Data
Infrared (IR) spectra were recorded with a Nicolet Impact 420 Fourier transform spectrophotometer using the KBr disc technique (0.5% dilution). 1H NMR spectra were obtained at 300 MHz on a Varian Gemini 300 instrument. Chemical shifts (δ) were recorded relative to CDCl3 (7.24 ppm). Electrospray mass spectra were recorded on a Waters APIQ-TOF Ultima instrument (ES-TOF).
Preparation of N-Tritylglycylcholesterylformylhydrazide
Cholesterylformylhydrazide (44.2 mg, 0.1 mmol) and N-tritylglycine NHS ester (41.4 mg, 0.1 mmol) were dissolved in a mixture of DMF : pyridine (1:1 v/v), in a total volume of 800 μ L. The reaction mixture was kept at room temperature overnight. The reaction was monitored by TLC. The product was extracted using a CHCl3 : H20 (1:1 v/v) mixture because the NHS dissolves in water and will be eliminated in the H2O layer. The CHCl3 extract was evaporated under reduced pressure and any excess pyridine co-evaporated with ethanol. The final product was crystallized from ethanol. The product was monitored using TLC and spraying with 33% sulphuric acid.
Product produced a single spot by TLC (Rf 0.96, chloroform:methanol, 95:5, v/v). Mp: 108–110°C (yield: 63.7 mg, 86%). IR (film): 3436 (b, N-H), 2929 (st, C─H), 1449 (m, C═C), 1654 (m, C═O), 1378 (w, C─N), 1510 (v, aromatic rings) cm− 1. 1H NMR (300 MHz, CDCl3) : δ 0.68 (s, 3H, C─CH3), 0.86 (d, 6H, CH─CH3), 0.89 (d, 3H, CH─CH3), 0.99 (s, 3H, C─CH3), 3.1 (bs, 1H, NH), 4.54 (m, 1H, Chol-H3α), 5.37 (d, 1H, Chol-H6), 7.2–7.4 (15H, trityl group) ppm. MS, m/z, ES-TOF: 744.55 [M + H+], 767.21 [M + Na+].
Preparation of N-Trityl-β -Alanylcholesterylformylhydrazide
Cholesterylformylhydrazide (44.2 mg, 0.1 mmol) and N-trityl-β -alanine NHS ester (42.8 mg, 0.1 mmol) were dissolved in a mixture of DMF: pyridine (1:1 v/v) in a total volume of 800 μ L and the resulting solution kept at room temperature overnight. The reaction was monitored by TLC. A mixture of CHCl3: H2O (1:1 v/v) was added to the reaction mixture to extract the product. The free NHS was eliminated in the water layer and the chloroform extract was evaporated under reduced pressure. The excess pyridine was co-evaporated with ethanol.
The product was crystallized from methanol and dried under reduced pressure and afforded a single spot by TLC (Rf 0.97, chloroform:methanol, 95:5, v/v). Mp: 110–112°C (yield: 54.9 mg, 72%). IR (film): 3426 (b, N─H), 2929 (st, C─H), 1649 (m, C═O), 1460 (m, C═C), 1383 (w, C─N), 1523 (v, aromatic rings) cm− 1. 1H NMR (300 MHz, CDCl3) : δ 0.65 (s, 3H, C─CH3), 0.86 (d, 6H, CH─CH3), 0.89 (d, 3H, CH─CH3), 0.98 (s, 3H, C─CH3), 4.45 (m, 1H, Chol-H3α), 5.34 (bs, 1H, Chol-H6) 7.18–7.52 (15H, trityl group) ppm. MS, m/z, ES-TOF: 758.5532 [M + H+], 781.55 [M + Na+].
Synthesis of Glycylcholesterylformylhydrazide (MS10)
N-tritylglycylcholesterylformylhydrazide (30 mg, 0.04 mmol) was dissolved in CHCl3 (300 μ L) and to this was added glacial acetic (600 μ L) acid and H2O (60 μ L). The reaction mixture was maintained at room temperature for 3 hr. The product was monitored by TLC in CHCl3:MeOH (95:5, v/v). TLC plates were first sprayed with ninhydrin to detect the presence of the amino groups and then with 33% H2SO4 to detect the cholesteryl moiety. The reaction mixture was evaporated to dryness under reduced pressure and sufficient petroleum ether (100–120°C) was added to the sample to remove any tritanol present in the reaction mixture. This mixture was then kept at room temperature overnight. The ether was finally removed and the mixture rinsed with petroleum ether before drying in a Rotavapor-R rotary evaporator to produce a powder.
The product gave a single spot by TLC (Rf 0.10, CHCl3:MeOH, 95:5, v/v). Mp: 203–204°C (yield : 19.1 mg, 96%). IR (film) : 3441 (b, N-H), 2934 (m, C─H), 1649 (m, C═O), 1260 (w, C─N), 1432 (m, C═C) cm− 1. 1H NMR (300 MHz, CDCl3) : δ 0.69 (s, 3H, C─CH3), 0.84 (d, 6H, CH─CH3), 0.89 (d, 3H, CH-CH3), 0.99 (s, 3H, C─CH3), 3.49 (2H, NH2), 4.53 (m, 1H, Chol-H3α), 5.36 (bs, 1H, Chol-H6) ppm. MS, m/z, ES-TOF: 502,4764 [M + H+], 524.4638 [M + Na+].
Synthesis of β -alanylcholesterylformylhydrazide (MS11)
N-trityl-β -alanylcholesterylformylhydrazide (49.9 mg, 0.066 mmol) was dissolved in CHCl3 (500 μ L). Approximately 100 μ L of 90% acetic acid was added to the reaction mixture that was then incubated at 37°C, and the product was monitored by TLC as described for MS01 over a 5-hr period until the reaction had reached completion. The reaction mixture was evaporated thereafter to dryness to form a white film in the flask. Sufficient petroleum ether (100–120°C) was added to fully immerse the product that was kept at room temperature overnight. The ether was removed and the product was further rinsed in petroleum ether before drying in a rotary evaporator to produce a white powder.
The product was then recrystallized from ethanol and dried in the Xerotec freeze drier. The product (33.5 mg, 98%) afforded a single spot by TLC (Rf 0.08). IR (film): 3431 (b, N─H), 2934 (st, C─H), 1664 (m, C═O), 1250 (m, C─N), 1408 (m, C═C) cm−1. MS, m/z, ES-TOF: 516.5375 [M + H+], 538.5215 [M + Na+].
Liposome Formulation
The preparation of liposomes was adapted from the method described by Gao and Huang (Citation1991). Briefly, MS10 or MS11 (2 μ mol) was mixed in a 1:1 mol ratio with DOPE as helper lipid in CHCl3 (1 mL). The lipid was deposited as a thin film on the inner wall of a 10 ml flask by evaporation of solvent in vacuo at 20°C (Büchii Rotavapor-R rotary evaporator). The thin film was rehydrated overnight (20°C) in 20 mM HEPES, 150 mM NaCl (pH 7.5) and the suspension was vortexed (5 min) and finally sonicated for 5 min at 20°C (Transsonic bath-type sonicator). Liposome suspensions were stored at 4°C for several weeks. Transmission electron microscopy was carried out to determine lamellarity and size distribution of liposomes. The procedure adopted entailed the glutaraldehyde-induced cross-linking of a liposome-containing BSA solution followed by staining of sections in uranyl acetate and lead citrate and has been described elsewhere (Kisoon et al. Citation2002). Samples were deposited on formvar G-200 coated grids and viewed in a Jeol 1010 electron microscope at 60 kV.
Lipoplex Characterization
Transmission Electron Microscopy
A 50 μ L solution of HEPES buffered saline (HBS) containing 6 or 7 μ g liposome lipid and 1 μ g pGL3 DNA was matured for 20 min, mixed with 0.5 % (w/v) uranyl acetate and placed on parafilm. After 3 min this was transferred to a formvar coated grid that was air dried overnight and viewed on a Jeol 1010 transmission electron microscope at 60 kV.
Band Shift Assay
Liposome:DNA mixtures in HBS (15 μ L) were incubated at 20°C for 30 min. Gel loading buffer (3 μ L; 50% glycerol, 0.5% bromophenol blue, 0.5% xylene cyanol, 72 mM Tris-HCl, 60 mM NaH2PO4, 20 mM EDTA at pH 7.5) was added and samples were applied to 1% agarose gels in a Mini-Sub® apparatus (BioRad). Electrophoresis was conducted at 50V for 90 min in 36 mM Tris-HCl, 30 mM NaH2PO4, 10 mM EDTA, pH 7.5). Gels were stained with ethidium bromide (1 μ g/mL) for 30 min and viewed under transillumination at 300 nm using a UVP gel documentation system (Upland, CA, USA).
Nuclease Digestion Assay
Liposome:DNA complexes in HBS (15 μ L) were stored for 20 min after initial mixing of liposome and DNA solutions, whereupon fetal bovine serum was added to a final concentration of 10% (v/v). After incubation at 37°C for 4 hr, mixtures were treated with EDTA and SDS to a final concentration of 10 mM and 0.5% (w/v), respectively, and subjected to agarose gel electophoresis and staining as described above.
Dye Displacement Assay
This assay was adapted from that described by Tros de Ilarduya et al. (Citation2002). Briefly, a mixture of ethidium bromide (1 μ g) in HBS (500 μ L) was used to provide a baseline reading (0%) for fluorescence adopting an excitation wavelength of 520 nm and an emission wavelength of 600 nm with the instrument (Shimadzu RF-551 spectrofluorometric detector) set at high sensitivity. Thereafter, 6 μ g of pBR322 DNA was added and the new reading set at 100% fluorescence. Aliquots of cationic liposome (5 μ g) were added stepwise to the solution until a total of 70 μ g of liposome lipid had been added. Solutions were mixed thoroughly after each addition before fluorescence measurements were taken.
Growth and Maintenance of Cell Lines
The three human cell lines (HepG2, HeLa, SNO) and murine line NIH-3T3 employed in this study were obtained from Highveld Biological (Pty), (Kelvin, South Africa). All lines were propagated in 25 cm2 flasks at 37°C in MEM (5 mL) containing 10% (v/v) fetal bovine serum, 20 mM HEPES, 10 mM NaHCO3, 100 U/ml penicillin G, and 100 μ g/ml streptomycin sulphate at pH 7.5. Cells were divided 1:3 (1:2 HepG2 cells) every 3–4 days and stored in a biofreezer (−80°C) in complete medium containing 10% DMSO.
Growth Inhibition Assays
Cells were trypsinized and seeded into 24-well plates at the following densities: 2.6 × 104, 2.2 × 104, 2.3 × 104, and 2.0 × 104 cells/well for HeLa, SNO, NIH-3T3, and HepG2 cells, respectively. Plates were incubated for 24 to 36 hr to permit attachment and growth of cells to semiconfluency. The liposome:DNA complexes in HBS (15 μ L) were incubated at 20°C prior to addition to wells. Cells were prepared by first removing the complete growth medium and replacing it with 0.5 mL of serum-free medium. The reaction complexes were then added to the wells and the cells were then incubated at 37°C for 4 hr. Thereafter, the medium was replaced with complete medium (MEM + 10% FCS + antibiotics). Cells were incubated for a further 48 hr.
Adherent cells were then quantified by the method of Schellekens and Stitz (Citation1980). Briefly, cells were washed twice with PBS and stained with 200 μ L crystal violet solution (0.5% W/V crystal violet, 0.8% W/Vsodium chloride, 5% V/V formaldehyde, 50% V/Vethanol) for 20 min. The stain was then removed and the cells washed extensively with water. The multiwell plates were then air dried for 24 hr, and the stain extracted with 2-methoxyethanol (0.5 mL) over a period of 36 hr, with gentle rocking (20 rev / minute) on a Stuart Scientific STR 6 platform shaker. Absorbance values for the samples were then measured in a Novospec spectrophotometer (Biochrom, Cambridge, UK), at a wavelength of 550 nm.
Transfection Studies
Assays were conducted in 24-well plates. HeLa, NIH-3T3, SNO, and HepG2 cells were seeded into wells at 2.6 × 104, 2.3 × 104, 2.2 × 104, and 2.0 × 104 cells/well, respectively, and grown to semiconfluency (36–48 hr). Complete medium was removed and cells were washed with PBS (0.5 mL) before addition of serum-free medium (MEM + antibiotics). Transfection complexes were added and cells incubated for 4 hr before removal of medium and addition of complete medium. Luciferase expression assays were conducted on cell lysates after 48 hr (Promega luciferase assay system) and results were expressed as relative light units/mg soluble cell protein. Transfections also were conducted in the presence of 10% fetal bovine serum.
RESULTS AND DISCUSSION
Cytofectins and Liposomes
Both MS10 and MS11 have a cholesterylformylhydrazide entity in common (). Attachment of the glycyl and β -alanyl moieties entailed primary amino group protection on the amino acids and carboxyl group activation with DCC and NHS followed by N-detritylation after coupling. Equimolar mixtures of the new cytofectins with DOPE, which has a strong tendency to adopt an inverted hexagonal structure (H11 phase) and which has the added property of reducing the cytotoxicity of cationic surfactants (Sternberg, Sorgi, and Huang Citation1994), facilitated the formation of unilamellar liposomes. MS10 and MS11 liposomes showed some size dispersity (100–200 nm and 50–200 nm, respectively) ( and ).
Lipoplex Ultrastructure
The enhancement of transfection efficiencies using nonviral methods such as cationic liposomes requires a full understanding of the supramolecular structures of cationic liposome:DNA complexes and their interaction with the cell membrane (Safinya Citation2001). Lipoplexes (200–350 nm) appeared as groups or clusters of spherical or oblong vesicles of ∼ 50 nm diameter. Lipoplexes formed at a liposome:DNA +/− charge ratio of 1.6:1 (6:1, w/w) ( and ) appeared similar to those prepared at a +/− charge ratio of 1.9:1 (7:1, w/w) ( and 3D). A +/− charge ratio greater than 1 is expected to give a positive zeta potential (net surface charge), which is thought to facilitate interaction of complexes with the anionic cell-surface proteoglycans (Zabner et al. Citation1995). Similar clusters have been described by others (Percot et al. Citation2004; Cao et al. Citation2000). The shape adopted by lipoplexes is influenced by the size of plasmid DNA, the type of liposome, and the conditions and mechanisms adopted for lipoplex formation (Sun et al. Citation2004).
Analysis of Liposome-DNA Interactions
Results presented in demonstrate the formation of electrostatic complexes between MS10 and MS11 liposomes and pGL3 plasmid DNA. All DNA is liposome-associated at a liposome:DNA ratio of 6:1 (w/w) or +/− ratio of 1.6:1 ( and , lane 6). Assuming that all the vesicles observed in lipoplex clusters are unilamellar, effectively half the positive charges, those on the outer leaflet of the membrane bilayer, will be available for interaction with the DNA. The effective +/− charge ratio therefore would be 0.8:1 (almost 1:1). It is understood that primary amino groups on cytofectins MS10 and MS11 are protonated at pH 7.5, as the pKb values for such groups are about 3.5. This, in large measure, explains the apparent excess positive charge observed in such complexes when full DNA retardation is observed in band shift assays (Cao et al. Citation2000; Kisoon et al. Citation2002).
FIG. 4 Band shift assay of interaction between MS10 (A) and MS11 (B) cationic liposomes and pGL3 DNA. Incubation mixtures (15 μ L) in HBS (pH 7.5) contained varying amounts of cationic liposomes. Lanes 1–8 (0, 1.0, 2.0, 3.0, 4.0, 5.0, 6.0, and 7.0 μ g). The plasmid DNA was kept constant at 1 μ g.
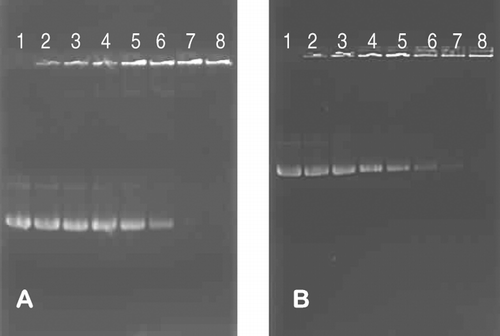
Cationic liposome-DNA interactions also were interrogated in ethidium displacement experiments. On intercalation of the planar fluorophore into double-stranded DNA a marked enhancement of fluorescence is observed (Even-Chen and Barenholz Citation2000). Introduction of liposomes, however, displaces the ethidium cation (Xu et al. Citation1999) in a rapid process with attendant reduction in fluorescence and compaction of the DNA. In (A and B) we see that the gradual increase in liposome concentration is marked by a decrease in fluorescence until a plateau is reached at a liposome:DNA ratio of 6:1 (w/w). Continued introduction of liposomes up to a ratio of 10:1 resulted in no further loss of fluorescence indicating no further DNA condensation. MS11 liposomes appeared to condense plasmid DNA to a greater extent than MS10 liposomes (40% and 30% displacement, respectively).
Results presented in (A and B) confirm that MS10 and MS11 lipoplexes afford protection to the plasmid DNA from assault by serum nucleases over a wide range of liposome:DNA ratios (1–7:1, w/w). Under the same experimental conditions naked DNA was extensively degraded ( and , lanes 2). If the liposome:DNA association is weak, nucleases may access and degrade DNA or alternatively destabilize the lipoplexes (Yang and Huang Citation1997). The inhibitory effect of serum on transfection efficiency of lipoplexes is a major obstacle to the application of gene delivery vectors both in vitro and in vivo (Ross and Hui Citation1999).
FIG. 5 Ethidium intercalation assay for MS10 (A) and MS11 (B) cationic liposomes at varying liposome:DNA (w/w) ratios. Incubation mixtures contained 6 μ g pBR322 DNA.
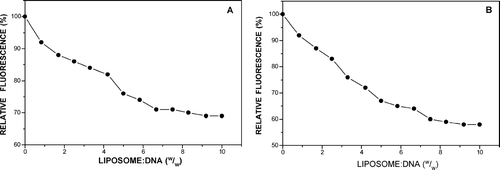
FIG. 6 Serum nuclease protection assays for MS10 (A) and MS11 (B) lipoplexes. Lane 1: undigested pBR322 DNA (1 μ g); Lane 2: unprotected plasmid DNA (1 μ g) digested by serum nucleases; Lanes 2–8: varying amounts of cationic liposomes (0, 2, 3, 4, 5, 6, and 7 μ g) with plasmid DNA (1 μ g) and serum (10%, v/v).
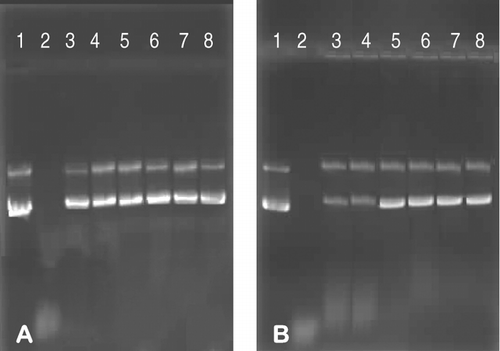
Growth Inhibition Studies
The toxicity of lipoplexes to cells was determined under experimental conditions adopted for transfection studies. Toxicities, in general, were found to increase with exposure to greater lipolex concentrations. At the highest lipoplex concentration tested (7 μ g liposome lipid/well) growth inhibitions by MS10 (MS11) lipoplexes were 28 (26), 20 (17), 31 (29), and 29 (26)% in HeLa, NIH-3T3, SNO, and HepG2 cells, respectively ( and ). Complexes therefore were well tolerated with MS11 exhibiting marginally lower levels of toxicity. There appears to be a direct relationship between toxicity and efficiency of transfection in cells (Wiethoff and Middaugh Citation2003).
FIG. 7 Growth inhibition studies of MS10 (A) and MS11 (B) liposome:DNA complexes in three human transformed epithelial cell lines (HeLa, SNO, HepG2) and the murine fibroblast line NIH-3T3. Cells in 0.5 mL MEM were treated with lipoplexes containing 1 μ g DNA and up to 7 μ g of liposomes. Data presented as means ± S.D. (n = 4).
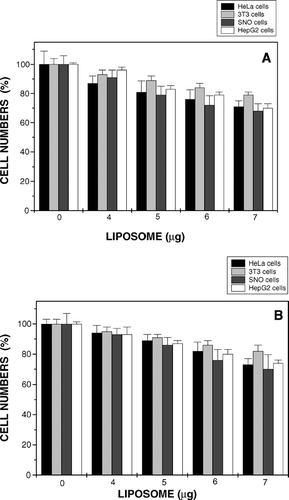
Transfection Activities of MS10 and MS11 Lipoplexes
Among the factors affecting transfection efficiencies of lipoplexes, perhaps the most important are size and zeta potential. Thus, in related lipoplexes size increases are associated with lower levels of transfection in CHO, COS-1, and HepG2 cells (Singh and Chaudhuri Citation2004). Moreover lipoplexes containing cholesterol-based cytofectins with high positive zeta potentials lead to elevated transfection activities in NIH-3T3, HeLa, and COS-7 cells (Takeuchi et al. Citation1996). In the present study, MS10 and MS11 lipoplexes prepared at 6:1 and 7:1 (w/w) liposome: DNA ratios showed no significant size difference and lay in the 200–350 nm range. Both cytofectins achieved highest transfection levels in HeLa cells, whereas luciferase activity levels in SNO, HepG2, and NIH-3T3 cells were similar at both liposome:DNA ratios for both MS10 and MS11 ( A–H).
FIG. 8 Transfection of HeLa (A, E), SNO (B, F), HepG2 (C, G), and NIH-3T3 (D, H) cells by MS10 (A–D) and MS11 (E–H) lipoplexes. Semiconfluent cells in 24-well dishes were exposed to 1 μ g of pGL3 DNA in complex with liposomes (3–7 μ g lipid). Control 1 represents endogenous luminescence of cells while control 2 reflects luciferase activity of cells treated with pGL3 DNA alone (1 μ g). Data presented as means ± S.D. (n = 4).
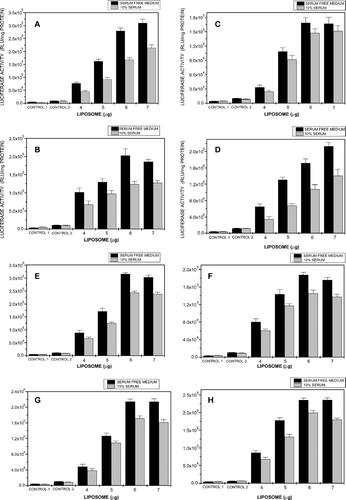
Cationic liposome-mediated DNA transfer and gene expression are often compromised by the presence of serum (Yang and Huang Citation1997). Hence transfections in this study also were carried out in the presence of 10% fetal bovine serum. Under these conditions MS10 lipoplex-mediated gene transfer at a ratio of 6:1 (w/w) was reduced to 61, 65, 57, and 85% of the activity in the absence of serum in HeLa, 3T3, SNO, and HepG2 cells, respectively ( A–D). At the same ratios MS11 lipoplexes achieved levels of 75, 85, 76, and 81% respectively ( E–H). Although MS10 and MS11 lipoplexes were approximately the same size, the DNA in MS11 complexes was more tightly packed as shown in the dye displacement assay ( and ). This may have afforded greater protection against serum nuclease digestion and challenge by negatively charged serum proteins thus ensuring delivery of more intact DNA to the cells.
CONCLUSION
The potential applicability of primary amino group-containing cholesterol-based cytofectins in transfection studies in mammalian cell lines in vitro was investigated. Thus in three human transformed epithelial cell lines—HeLa (cervical), SNO (esophageal) and HepG2 (hepatocyte derived)—and in the murine fibroblast line NIH-3T3, glycylcholesterylformylhydrazide (MS10), and β -alanylcholesterylformylhydrazide (MS11)-containing cationic liposomes in complex with pGL3 plasmid promote favorable transfection levels as measured by luciferase activity. Although the spacer length between the cationic head and the hydrophobic anchor is an important factor to be considered in the design of new cytofectins (Remy et al. Citation1995; Wheeler et al. Citation1996), this study does not reveal significant differences in transfection activities in the absence of serum between MS10 and MS11, which has extra methylene in the spacer. However, the MS11 complexes showed unusually high transfection activity in the presence of serum, perhaps due to the increased compaction of the DNA cargo in these lipoplexes.
REFERENCES
- Ariatti M., Hawtrey A. Synthesis of certain puromycin analogues and their use in studying the peptidyl synthetase enzyme of E. coli and rat liver ribosomes. S. Afr. J. Med. Sci. 1975; 40: 197–303
- Blaese R. M., Culver K. W., Anderson. The ADA human gene therapy clinical protocol. Gene Ther. 1990; 1: 331–337
- Boussif O., Lezoulach F., Zanto M. A., Mergny M. P., Scherman D., Demeniex B., Behr J. P. A versatile vector for gene and oligonucleotide delivery into cells in culture and in vivo: polyethyleneimine. Proc. Natl. Acad. Sci. USA 1995; 92: 7297–7314
- Buchberger B., Fernholz E., Bantle E., Weigert M., Borowski E., van der Eltz H., Hinzpeter M. DOSPER liposomal transfection reagent: a reagent with unique transfection properties. Biochimica 1996; 2: 7–10
- Cao A., Briane D., Coudet R., Reynier P., Bouchemal N., Lievre N., Hantz E., Salzmann J. L., Rigaut J. P., Taillandier E. Delivery and pathway in MCF7 cells of DNA vectorized by cationic liposomes derived from cholesterol. Antisense Nucl. Acid Drug Dev. 2000; 10: 369–380
- Corsi K., Chellat F., Yahia L., Fernandes J. C. Mesenchymal stem cells, MG63 and HEK293 transfection using chitosan-DNA nanoparticles. Biomaterials 2003; 24: 1255–1264
- Dong Y., Skoultchi A. I., Pollard J. W. Efficient DNA transfection of quiescent mammalian cells using poly-L-ornithine. Nucleic Acids Res. 1993; 21: 771–772
- Even-Chen S., Barenholz Y. DOTAP cationic liposomes prefer relaxed over supercoiled plasmids. Biochim. Biophys. Acta 2000; 1509: 176–188
- Felgner P. L., Gadek T. R., Holm M., Roman R., Chan H. W., Wenz M., Northrop J. P., Ringold G. M., Danielson M. Lipofection: a highly efficient lipid-mediated DNA-transfection procedure. Proc. Natl. Acad. Sci. USA 1987; 84: 7413–7417
- Gao X., Huang L. A novel cationic liposome reagent for efficient transfection of mammalian cells. Biochem. Biophys. Res. Commun. 1991; 179: 280–285
- Kisoon N., Ariatti M., Moodley T. A novel cationic cholesterol derivative, its formulation into liposomes and the efficient transfection of the transformed human cell lines HepG2 and HeLa. Drug Deliv. 2002; 9: 161–167
- Marshall J., Yew N. S., Eastman S. J., Jiang C., Scheule R. K., Cheng S. H. Cationic lipid-mediated gene delivery to the airways. Non-Viral Vectors for Gene Therapy, L. Huang, M.-C. Hung, E. Wagner. Academic Press. 1999; 39–68
- Mumper R. J., Duguid J. G., Anwer K., Barron M. K., Nitta H., Roland A. P. Polyvinyl derivatives as novel interactive polymers for controlled gene delivery to muscle. Pharm. Res. 1996; 13: 701–709
- Percot A., Briane D., Coudet R., Reynier P., Bouchemal N., Lievre N., Hantz E., Salzmann J. L., Cao A. A hydroxyethylated cholesterol-based cationic lipid for DNA delivery: effect of conditioning. Int. J. Pharm. 2004; 278: 143–163
- Remy J. S., Kichler A., Mordvinov V., Schiber F., Behr J. P. Targeted gene transfer into hepatoma cells with lipopolyamine-condensed DNA particles presenting galactose ligands: a stage toward artificial viruses. Proc. Natl. Acad. Sci. USA 1995; 92: 1744–1748
- Reynier P., Briane D., Coudert R., Fadda G., Bouchemal N., Bissieres P., Taillandier E., Cao A. Modifications in the head group and in the spacer of cholesterol-based cationic lipids promotes transfection in melanoma B16–F10 cells and tumours. J. Drug Target. 2004; 12: 25–38
- Ross P. C., Hui S. W. Lipoplex size is a major determinant in in vitro lipofection efficiency. Gene Ther. 1999; 6: 651–659
- Safinya C. R. Structures of lipid-DNA complexes: supramolecular assembly and gene delivery. Curr. Opin. Str. Biol. 2001; 11: 440–448
- Schellekens H., Stitz L. W. Simple method for measuring growth inhibition by interferon of cells in monolayer. J. Virol. Meth. 1980; 1: 197–200
- Singh M., Ariatti M. A cationic cytofectin with long spacer mediates favourable transfection in transformed human epithelial cells. Int. J. Pharm. 2006; 309: 189–198
- Singh R. S., Chaudhuri A. Single additional methylene group in the head group region imparts high gene transfer efficiency to a transfection-incompetent cationic lipid. FEBS Lett. 2004; 556: 86–90
- Sternberg B., Sorgi F. L., Huang L. New structures in complex formation between DNA and cationic liposomes visualized by freeze-fracture electron microscopy. FEBS Lett. 1994; 356: 361–366
- Sun Y., Yu X., Takaoka Y., Oh-i S., Düzgüneş N., Hirota S. Comparison of the shape parameters of DNA-cationic lipid complexes and model polyelectrolyte-lipid complexes. J. Colloid Interface Sci. 2004; 276: 317–322
- Sykes P. A Guidebook to Mechanism in Organic Chemistry. 3rd ed. Longman, London 1970
- Takeuchi K., Ishihara M., Kawaura C., Noji M., Furuno T., Nakanishi M. Effect of zeta potential of cationic liposomes containing cationic cholesterol derivatives on gene transfection. FEBS Lett. 1996; 397: 207–209
- Tros de Ilarduya C., Arangoa M. A., Moreno-Aliaga M. J., Düzgüneş N. Enhanced gene delivery in vitro and in vivo by improved transferrin lipoplexes. Biochim. Biophys. Acta 2002; 1561: 209–221
- Wheeler C. J., Sukhu L., Yang G., Tsai Y. J., Bustamenta C., Felgner P., Norman J., Manthorpe M. Converting an alcohol to an amine in a cationic lipid dramatically alters the co-lipid requirements, cellular transfection activity and the ultrastructure of DNA-cytofectin complexes. Biochim. Biophys. Acta 1996; 1280: 1–11
- Wiethoff C. M., Middaugh C. R. Barriers to non-viral gene delivery. J. Pharm. Sci. 2003; 92: 203–217
- Wu G. Y., Wu C. H. Receptor-mediated in vitro gene transformation by a soluble DNA carrier system. J. Biol. Chem. 1987; 262: 4429–4432
- Wu G. Y., Wu C. H. Receptor-mediated gene delivery and expression in vivo. J. Biol. Chem. 1988; 263: 14621–14624
- Xu Y., Hui S. -W., Frederik P., Szoka F. Physicochemical characterization and purification of cationic liposomes. Biophys. J. 1999; 77: 341–353
- Yang J. P., Huang L. Overcoming the inhibitory effect of serum on lipofection by increasing the charge ratio of cationic liposomes to DNA. Gene Ther. 1997; 6: 651–659
- Yi Y., Lee K. H. Retroviral gene therapy: safety issues and possible solutions. Curr. Gene Ther. 2005; 5: 25–35
- Zabner J., Fasbender A. J., Moninger T., Poellinger K. A., Welsh M. J. Cellular and molecular barriers to gene transfer by a cationic lipid. J. Biol. Chem. 1995; 270: 18997–19007