Abstract
The aim of this study was to develop mannosylated liposomes as bioadhesive carriers for oral drug delivery. Two kinds of acyclovir (ACV)–entrapped mannosylated liposomes, i.e. ManN-ACV-lip and PAM-ACV-lip, were prepared by the use of mannosamine HCl (ManN) and p-aminophenyl-α-D-mannopyranoside (PAM), respectively. The mean sizes, drug entrapment efficiency, and loading capacity values of all liposomal formulations were in the ranges of 233–371 nm, 82–95%, and 42–47%, respectively. The mean size of PAM-ACV-lip was significantly smaller than those of conventional ACV liposomes and ManN-ACV-lip due to the more conical packing parameter of mannose-conjugated phospholipid. The mannosylating group grafted into bilayer membrane resulted in a decrease in drug entrapment, owing to competitive binding. The in vitro drug absorptions through everted sacs of mice ileum of both mannosylated ACV liposomes were significantly higher than those of conventional ACV liposomes or suspension.
Introduction
Membranous epithelial cells (M cells) are specialized epithelial cells overlying the subepithelial lymphoid follicles in the gastrointestinal tract, especially terminal ileum and respiratory tracts (CitationKreuter, 1991; CitationYoung & Heath, 2000). In the intestine, Peyer’s patches are the major sites of antigen and microorganism sampling. Aggregated or isolated lymphoid follicles are separated from the lumen by a specialized epithelium that contains scattered M cells (CitationPringault, 1999). These cells are specialized for antigen sampling and also exploited as a route of host invasion by many pathogens. In addition, M cells possess a high transcytotic capacity and are able to transport a broad range of materials including particulates. From these abilities of M cells, they could be used as a potential portal to deliver drugs, vaccines, and other bioactive materials (CitationErmak & Giannasca, 1998; CitationClark, Jepson, & Hirst, 2001).
One of the parameter that should be addressed is the size of the particles. It is well known that the size of the particles should be below 10 μm in order to be taken up by M cells of the Peyer’s patches in the gut (CitationErmak & Giannasca, 1998). The study on the preparation of coated nanoparticles has been carried out consistently with this approach (CitationBorges et al., 2005). Some studies suggested that M cell delivery could be facilitated by restricting delivery vehicles to the nanometer size range. Basically, particles may interact with M cells via both non-specific and specific receptor-mediated mechanisms. Non-specific mechanisms are dependent on surface charge and hydrophobicity. On the other hand, a more reliable method to enhance particle absorption would be to chemically modify the surface of the particles with ligand such as lectin so that they can interact specifically via receptor-mediated interaction with M cell apical membranes (CitationClark et al., 2002; CitationJepson, Clark, & Hirst, 2004; CitationZhang et al., 2005).
Acyclovir [9-(2-hydroxyethoxylmethyl) guanine], a synthetic purine nucleoside analog derived from guanine, is most widely used as an effective anti-viral agent in the treatment of herpes simplex virus (HSV), mainly HSV-1 and HSV-2, and varicella-zoster virus (CitationO’Brien & Campoli-Richards, 1989). However, acyclovir absorption in the gastrointestinal tract is slow, variable, and incomplete. It is estimated that merely 10–30% of an oral dose of the drug is absorbed (CitationMcEvoy, 2005). The limited bioavailability of orally administered acyclovir is likely due to poor absorption of the drug. Many attempts have been made to improve the oral bioavailability and the therapeutic effectiveness of acyclovir such as chemical modifications (CitationBeutner, 1995) or development of novel drug delivery systems of acyclovir (CitationGhosh et al., 2006).
The purpose of this investigation was to improve the absorption of acyclovir via M cells and Peyer’s patches by using a novel drug delivery system of mannosylated acyclovir liposomes, which was able to exhibit a strong bioadhesive capacity to gut mucosal tissue including Peyer’s patches after oral administration. In fact, mannose residues found on the surface of many microorganisms can allow their adhesion to the gut mucosal cells. This may be related to the interaction with mannose binding lectins (MBL) expressed on the gut mucosa and lymphoid tissue cells (CitationWagner et al., 2003). The physicochemical characterizations and in vitro absorption of the developed mannosylated acyclovir liposomes from the mucosal to the serosal surface using everted sac method of mice ileum compared with free drug were subsequently studied.
Materials and methods
Materials
Acyclovir micronized (ACV) was obtained from Zhejiang Wuyi Pharmaceutical Factory (China). Phosphatidylcholine (PC) (Phospholipon® 90G) was a gift from Rhône Poulenc Rorer (Köln, Germany). Cholesterol (Chol) and sodium hydroxide were from Merck (Darmstadt, Germany). D-mannosamine HCl (ManN) was obtained from Fluka Chemie (Buchs, Switzerland). Dicetylphosphate (DCP) and p-aminophenyl-α-D-mannopyranoside (PAM) were purchased from Sigma (St. Louis, MI). Sodium lauryl sulfate, potassium dihydrogen orthophosphate, sodium chloride, potassium chloride, and calcium chloride were from Ajax Finechem (Seven Hills, Australia). Sodium hydrogen carbonate and magnesium sulfate were from Carlo Erba Reagenti (Milano, Italy). Chloroform and methanol were from Labscan (Ireland).
Preparation of mannosylated ACV liposomes
ManN and PAM were used to prepare two kinds of mannosylated ACV liposomes, i.e. ManN-ACV-lip and PAM-ACV-lip, respectively. In the case of ManN-ACV-lip, DCP was employed to introduce negative charge on the surface of liposomes. Total lipid of 200 mg at PC:Chol molar ratio of 7:3 was dissolved in 10 ml chloroform. ACV 120 mg and 10 mg ManN or 10 mg PAM were dissolved in 240 ml methanol, which was subsequently added to the lipid mixture solution. The solvent was evaporated out at 41°C for 1 h (Eyela® A-3S, Tokyo Rikakikai, Japan) until a dry thin film was obtained, which was hydrated by phosphate buffer pH 7.0 at 60°C for 1 min thereafter. The liposomal dispersion was then completely hydrated at 54°C for 2 h and subsequently sonicated at room temperature for 15 min (Crest® 950D, Crest Ultrasonic Corp., Trenton, NJ). The Mannosylated ACV liposomes were finally purified by ultracentrifugation at 93 000 g for 1 h at 4°C (Beckman® L-70, Beckman, Fullerton, CA) and pellet washing until the ACV concentration in the supernatant was less than 6 μg/ml. The liposomes were finally filled and sealed under N2 gas, and kept at 4°C prior to use.
Physicochemical characterization of mannosylated ACV liposomes
The morphology of ManN-ACV-lip and PAM-ACV-lip were examined using a scanning electron microscope (SEM) (Jeol® JSM-5410LV, Jeol, Tokyo, Japan) and a transmission electron microscope (TEM) (Jeol® JEM-2100, Jeol, Tokyo, Japan). Their particle sizes and zeta potentials were measured by using a submicron particle analyzer (Coulter® N4MD, Coulter Corporate Communication, Hialeah, FL) and a zeta potential analyzer (Zetasizer® Nanoseries, Malvern Instruments Ltd., Worcestershire, UK), respectively. The entrapment efficiency (% EE) and loading capacity (% LC) of ManN-ACV-lip and PAM-ACV-lip according to equations (1) and (2), respectively, were determined spectrophotometrically at 255 nm (Beckman® DU 650i, Beckman, Fullerton, CA) after diluting 500-fold with 2% sodium lauryl sulfate solution.
In vitro absorption study in mice ileum sac
Animal care
Male Swiss albino mice weighing 35–45 g were supplied by the National Laboratory Animal Centre, Mahidol University, Salaya Campus (Nakornpathom, Thailand). Animal procedures were ethically approved by the Institutional Animal Care and Use Committee, Faculty of Pharmacy, Mahidol University (Bangkok, Thailand). The approved number was No 1/2550. Five mice were housed in one stainless steel box case (24 × 34 × 12 cm) in an air-conditioned room (25°C) and subject to a 12 h light/dark cycle at the Experimental Animal Unit of Faculty of Pharmacy, Mahidol University (Bangkok) for at least 1 week prior to the experiment. Food and water were fed ad libitum.
Preparation of mice ieum sac
The mice ileum was isolated immediately from the animal body right after the cervical dislocation. The technique in preparation of everted sac is as follows. First, a glass stirring rod was carefully inserted along the lumen and tied up at one end with the stitching silk. The ileum was then everted by the use of stirring rod so that the mucosal surface became outside and serosal surface inside. The ileum was subsequently tied at one side with stitching silk and filled with Kreb-Henseleit solution. The other side of the ileum was then tied so that the ileum became separated into sacs with the length of 3 cm. The sacs were finally incubated at 37°C in Kreb-Henseleit solution and bubbled with 5% CO2 + 95% O2 until use.
In vitro absorption study
The prepared everted sacs were immersed in 10 ml of ACV suspension, conventional ACV liposomes (ACV-lip), ManN-ACV-lip, and PAM-ACV-lip in Kreb-Henseleit solution. Afterward, the solution was aerated with 5% CO2 + 95% O2 and continuously shaken at 37°C for 1 h in a shaking water bath (HETOMIX® TBVS, Heto, Allerod, Denmark). After incubation, the sacs were cut open to collect the internal serosal medium. Mucus in the sample collected from the ileum sac was removed by centrifugation at 11 000 g for 5 min (Hettich® Universal 30F, Hettich GmbH, Tuttlingen, Germany) and its volume was measured. The % ACV absorbed through mice ileum was determined by high-performance liquid chromatography (HPLC). The experiments were done in 10 replications. The absorption rate of ACV through mice ileum was expressed in terms of % drug being absorbed within 1 h.
HPLC analysis of ACV
The ACV concentrations in all samples were determined by HPLC (Shimadzu® C-10AT VP equipped with SPD-10A VP UV-VIS detector and SCL-10A VP system controller, Shimadzu, Kyoto, Japan). A C18 reverse-phase column (Mightysil® RP-C18 GP Aqua column, 5 μm, 4.6 × 250 mm, Kanto Chemical, Tokyo, Japan) was used at ambient temperature. The mobile phase was 0.1% v/v glacial acetic acid solution at a flow rate of 1.4 ml/min. The sample injection volume was 50 μL and the detection wavelength was 254 nm. The retention time was 14.7 min. A very high degree of linearity with the correlation coefficient (r2) of 0.9999 was achieved across the specified range of 1–50 μg/ml. The accuracy of HPLC analytical method in terms of mean values (n = 3) of % recovery of ACV at 1, 10, and 50 μg/ml were 101.29 ± 0.77, 99.22 ± 0.70, and 99.21 ± 0.34%, respectively. The precision of the assay procedure expressed as % CV’s (n = 3) at 1, 10, and 50 μg/ml were 0.96, 0.12, and 0.08% for intra-day, and 1.13, 0.40, and 0.09% for inter-day, respectively.
Results and discussion
Physicochemical characterization of mannosylated ACV liposomes
Microscopic appearance
The SEM and TEM images of ACV-lip, ManN-ACV-lip, and PAM-ACV-lip are illustrated in . It can be seen that their morphology was almost spherical in nature, although particle sizes were apparently different according to their compositions and surface charges. Their sizes were obviously shown to be in nanometer range, which were the same order of magnitude as those measured by the light scattering method. The existence of the phospholipid bilayers could be evidenced by the multilamellar structure shown in the TEM images, which are the typical characteristics of vesicles prepared by chloroform film method.
Zeta potential and particle size
The zeta potential of ACV-lip was found to be slightly negative (−4.52 mV), as shown in . The pKa’s of ACV at 2.27 and 9.25 (CitationMcEvoy, 2005) suggested that the pure drug powder would be in the ionized form with negative charge at pH 7.0. This was confirmed by the experimental value of zeta potential at −14.03 ± 4.04 mV. It should be noted that the net negative charge of ACV-lip was far below that of the pure drug powder, which may be due to the fact that the ionized ACV could almost completely be hidden inside the bilayer membrane. It was also shown that DCP did introduce negative surface charge (–26.73 mV) on ManN-ACV-lip via the ionization of acidic –HPO4 group. Since the –NH2 group of ManN was in the protonated form with positive charge in buffer pH 7.0, it would likely interact with the oppositely negative charge on surface of liposomes by electrostatic attraction. The zeta potential of PAM-ACV-lip appeared to be slightly positive (0.54 mV), which may be due to protonation at –NH2 group of PAM.
Table 1. Zeta potential, particle size, entrapment efficiency (% EE), and loading capacity (% LC) of ACV-lip, ManN-ACV-lip, and PAM-ACV-lip.
The mean sizes of ACV-lip, ManN-ACV-lip, and PAM-ACV-lip () were in the range of 233–371 nm, which were all significantly different (p < 0.05, ANOVA, LSD). The size of negatively charged vesicles was much larger than that of the other two types of vesicles. Upon hydration with buffer pH 7.0, negative charge was immediately developed on the charge-introducing lipid molecule followed by the orientation of phospholipid molecules into bilayer membrane. This bilayer membrane would subsequently curve and split up to form small closed vesicles in order to reduce its free energy. The type of charge so developed within the bilayer membrane just before the formation of vesicles may likely modify not only the rigidity of the membrane but also the rate of curving, splitting, and vesicle forming, which in turn determined the size of ManN-ACV-lip. In contrast, the smallest size of PAM-ACV-lip may be attributed to the more conical packing parameter of mannose-conjugated phospholipid (due to the large carbohydrate head group) compared with the more cylindrical packing parameter of the PC phospholipid. As mannose-conjugated phospholipid was incorporated into the liposome bilayer, the angle of curvature of liposomal membrane was increased, resulting in progressively smaller particles (CitationWhite et al., 2006). Although the size of PAM-ACV-lip was significantly less than ACV-lip according to this reason, ManN-ACV-lip was conversely larger than ACV-lip. This was probably due to the prevailing effect of surface charge over the dimensional orientation of PAM in bilayer membrane.
Entrapment efficiency and loading capacity
It is shown in that ACV could appreciably be entrapped into all three ACV liposomal formulations studied, the entrapment efficiency (% EE) and loading capacity (% LC) of which ranged from 82–95% and 42–47%, respectively. Both % EE and % LC of ACV-lip were significantly higher than that of PAM-ACV-lip, which in turn was higher than that of ManN-ACV-lip (p < 0.05, ANOVA and LSD). Such differences could be attributed to the combined effects of surface charge and mannosylating group on the surface of liposomes. The lowest entrapment of ManN-ACV-lip would be due to the electrostatic repulsion of the same negative charge of ionized ACV and liposomes during hydration. On the other hand, drug entrapments of neutral PAM-ACV-lip as well as slightly negative ACV-lip were certainly unaffected by electrostatic interaction but the hydrophobic force instead. However, the competitive binding inside the same hydrophobic region between molecules of mannosylating group and the drug in PAM-ACV-lip resulted in lower entrapment as compared to ACV-lip.
In vitro absorption study in mice ileum sac
The % ACV absorbed from the mucosal to the serosal side of everted sacs of mice ileum during 1-h incubation at 37°C of both ManN-ACV-lip (0.35 ± 0.06%) and PAM-ACV-lip (0.31 ± 0.04%), as shown in , were significantly higher than that of ACV suspension (0.22 ± 0.05%) (p < 0.05, Dunnett T3), but not significantly different from that of ACV-lip (0.26 ± 0.03%). Similarly, revealed that the apparent permeability coefficients (Papp) of ManN-ACV-lip (1.570 ± 0. 283 cm/min) and PAM-ACV-lip (1.374 ± 0. 167 cm/min), but not that of ACV-lip (1.132 ± 0. 126 cm/min), were significantly higher than that of ACV suspension (0.998 ± 0. 245 cm/min) (p < 0.05, Dunnett T3).
Figure 2. Percentage drug absorbed through mice ileum of ACV suspension, ACV-lip, ManN-ACV-lip, and PAM-ACV-lip after incubated at 37°C for 1 h. Data are the average of 10 different experiments and the error bars are standard deviations.
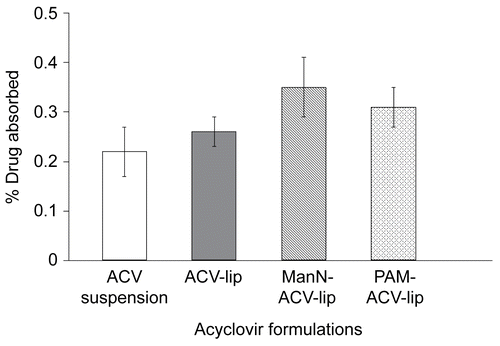
Figure 3. Permeability coefficient through mice ileum of acyclovir suspension, ACV-lip, ManN-ACV-lip, and PAM-ACV-lip after incubated at 37°C for 1 h. Data are the average of 10 different experiments and the error bars are standard deviations.
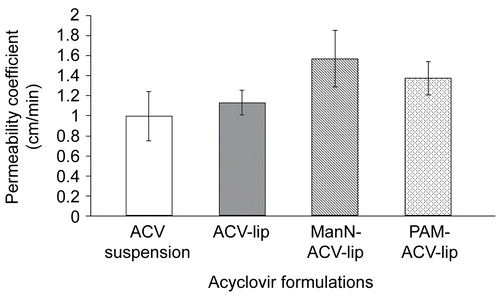
It is clearly demonstrated from the results above that both % drug absorbed and Papp values of mannosylated ACV liposomal formulations (ManN-ACV-lip and PAM-ACV-lip) were significantly higher than those of conventional ACV liposomes (ACV-lip) and ACV suspension. Such findings suggested that the strong adhesion to the gut mucosal wall of mannosylated ACV liposomes via the interaction with mannose binding lectins (MBL) expressed on the gut mucosa and lymphoid tissue cells could likely promote substantial drug transport through the gi-tract. It was also reported that the uptake of liposomes was greater in rat’s Peyer’s patch than in non-patch tissues due to the absorption of particulates predominantly taking place at gut associated lymphoid tissue (GALT) involving isolated lymphoid follicles and Peyer’s patches (CitationAramaki et al., 1993). Although lipid delivery vehicles could generally enhance particulates uptake across M cells, the uptake of ACV from conventional liposomes (ACV-lip) in this study was inadequate to bring about significant improvement compared to ACV suspension. There are several perceptible advantages for bioadhesive drug delivery systems: (i) as a result of the adhesion, the formulation stays longer at the delivery site and this improves the bioavailability of the drug; (ii) by using specific bioadhesive molecules it will be possible to target to a particular site or tissue, for example, in the gi-tract; and (iii) increased residence time (CitationWoodley, 2001). The results in this study have demonstrated the potential of mannosylated liposomal systems for increasing the bioavailability of poorly absorbable drugs.
Conclusions
Mannosylated ACV liposomes were developed by means of grafting and electrostatic binding of mannosylating groups on the surface of liposomes, the in vitro absorption of which were significantly higher than those of ACV suspension and conventional liposomes. It was suggested that the strong adhesion to the gut mucosal wall of mannosylated ACV liposomes via the interaction with mannose binding lectins (MBL) expressed on the gut mucosa and lymphoid tissue cells could likely promote substantial drug transport through the gi-tract.
Acknowledgements
Declaration of interest: The authors report no conflicts of interest. The authors alone are responsible for the content and writing of the paper.
References
- Aramaki, Y., Tomizawa, H., Hara, T., Yachi, K., Kikuchi, H., Tsuchiya, S. (1993). Stability of liposomes in vitro their uptake by rat Peyer’s patches following oral administration. Pharm Res. 10, 1228–31.
- Beutner, K.R. (1995). Valacyclovir: a review of its antiviral activity, pharmacokinetic properties, and clinical efficacy. Antiviral Res. 28, 281–90.
- Borges, O., Borchard, G., Verhoef, J.C., Sousa, A.D., Junginger, H.E. (2005). Preparation of coated nanoparticles for a new mucosal vaccine delivery system. Int J Pharm. 299, 155–66.
- Clark, M.A., Jepson, M.A., Hirst, B.H. (2001). Exploiting M cells for drug and vaccine delivery. Adv Drug Deliv Rev. 50, 81–106.
- Clark, M.A., Blair, H., Liang, L., Brey, R.N., Brayden, D., Hirst, B.H. (2002). Targeting polymerised liposome vaccine carriers to intestinal M cells. Vaccines. 20, 208–17.
- Ermak, T., Giannasca, P.J. (1998). Microparticle targeting to M cells. Adv Drug Deliv Rev. 34, 261–83.
- Ghosh, P.K., Majithiya, R.J., Umrethia, M.L., Murthy, R.S. (2006). Design and development of microemulsion drug delivery system of acyclovir for improvement of oral bioavailability. AAPS Pharm Sci Tech. 7, 1–6.
- Jepson, M.A., Clark, M.A., Hirst, B.H. (2004). M cell targeting by lectins: a strategy for mucosal vaccination and drug delivery. Adv Drug Deliv Rev. 56, 511–25.
- Kreuter, J. (1991). Peroral administration of nanoparticles. Adv Drug Deliv Rev. 7, 71–86.
- McEvoy, G.K. (2005). AHFS Drugs Information, American Society of Health System Pharmacists. Bethesda, MD: Atlantic Books.
- O’Brien, J.J., Campoli-Richards, D.M. (1989). Acyclovir. An updated review of its antiviral activity, pharmacokinetic properties and therapeutic efficacy. Drugs. 37, 233–309.
- Pringault, E. (1999). Emerging concepts in M cell function and Peyer’s patch development. Immunology. 11, 155–6.
- Wagner, S., Lynch, N.J., Walter, W., Schwaeble, W.J., Loos, M. (2003). Differential expression of the murine mannose-binding lectins A and C in lymphoid and nonlymphoid organs and tissues. J Immunol. 170, 1462–5.
- White, K.L., Rades, T., Furneaux, R.H., Tyler, P.C., Hook, S. (2006). Mannosylated liposomes as antigen delivery vehicles for targeting to dendritic cells. J Pharm Pharmacol. 58, 729–37.
- Woodley, J. (2001). Bioadhesion: new possibilities for drug administration? Clin Pharmacokinet. 40, 77–84.
- Young, B., Heath, J.W. (2000). Wheater’s functional histology. 4th ed. London: Churchill Livingstone.
- Zhang, N., Ping, Q.N., Huang, G.H., Xu, W.F. (2005). Investigation of lectin-modified insulin liposomes as carriers for oral administration. Int J Pharm. 294, 247–59.