Abstract
Hepatocellular carcinoma (HCC) is the most common form of primary liver cancer with high incidence globally. Increasing mortality and morbidity rates combined with limited treatment options available for advanced HCC press for novel and effective treatment modalities. Gene therapy represents one of the most promising therapeutic options. With the recent approval of herpes simplex virus for advanced melanoma, the field of gene therapy has received a major boost. Adeno-associated virus (AAV) is among the most widely used and effective viral vectors today with safety and efficacy demonstrated in a number of human clinical trials. This review identifies the obstacles for effective AAV based gene delivery to HCC which primarily include host immune responses and off-target effects. These drawbacks could be more pronounced for HCC because of the underlying liver dysfunction in most of the patients. We discuss approaches that could be adopted to tackle these shortcomings and manufacture HCC-targeted vectors. The combination of transductional targeting by modifying the vector capsid and transcriptional targeting using HCC-specific promoters has the potential to produce vectors which can specifically seek HCC and deliver therapeutic gene without significant side effects. Finally, the identification of novel HCC-specific ligands and promoters should facilitate and expedite this process.
Introduction
Liver cancer is the fifth most common cancer and causes one third of cancer related deaths worldwide (Siegel et al., Citation2015). With an estimated 746 000 deaths in 2012 alone; its incidence is rising globally (Siegel et al., Citation2015; Zhang et al., Citation2015). Difficulty in the effective management of liver cancer is a result of a combination of poor diagnosis and prognosis and limited therapeutic options.
Hepatocellular carcinoma (HCC), which accounts for over 80% of liver cancer, mostly develops in the cirrhotic liver (El-Serag & Rudolph, Citation2007). Liver cirrhosis may be caused by a number of factors including chronic viral hepatitis, alcohol abuse, inherited metabolic maladies (e.g. hemochromatosis) and nonalcoholic fatty liver disease (Liver & Cancer, Citation2012). The wide range of predisposing conditions coupled with the overlap of hepatic clinical symptoms generally makes early diagnosis of HCC difficult (Forner et al., Citation2012; Liver & Cancer, Citation2012). As a result, most patients are diagnosed at an advanced stage and have a poor prognosis (Forner et al., Citation2012). Even with improved surveillance and detection, overall survival rate for HCC has not improved, highlighting the poor status of current therapy (Liver & Cancer, Citation2012; Siegel et al., Citation2015).
Liver transplant is the best option to cure both HCC and cirrhosis but its suitability is limited by tumor stage and patient’s health status as well as the lack of donors, difficulty to obtain histological parameters before transplant, problems with graft rejection and the possibilities of opportunistic infections due to immunosuppression (Liver & Cancer, Citation2012; Waghray et al., Citation2015). Tumor resection or ablation is an option available for early stage HCC but recurrence or the development of new tumors in the diseased liver is frequently observed (Waghray et al., Citation2015). Additionally, there is a significant risk of mortality and morbidity when using these methods because of the underlying condition of the cirrhotic liver (Forner et al., Citation2012). Sorafenib, a multikinase inhibitor, is the only drug available for systemic therapy of HCC (Llovet et al., Citation2008; Waghray et al., Citation2015); however its efficacy is limited with an overall increase in survival of 2–3 months (Cheng et al., Citation2009; Flaherty & Sun, Citation2009). In addition, it can only be used in patients with preserved liver function (Torrecilla & Llovet, Citation2015). With the incidence of liver cancer growing and a lack of an effective treatment options, there is a pressing need for the development of novel targeted therapies against HCC. Gene therapy, which involves the transfer of a therapeutic gene to diseased cells or tissues using a vector, is one attractive treatment strategy (Amer, Citation2014).
AAV gene therapy for HCC
An ideal gene therapy vector should exhibit properties of low pathogenicity and an ability to maintain sustained levels of therapeutic gene expression in a wide variety of target tissue/cell and induce minimal activation of the immune system. Adeno-associated virus based vectors (AAVs) have many of these characteristics (Luo et al., Citation2015). For HCC gene therapy, a number of preclinical studies have been conducted with AAV vectors to establish their effectiveness (). These strategies include restoration of tumor suppressors, delivery of cytotoxic genes, gene directed enzyme prodrug therapy (GDEPT), inhibiting angiogenesis, and genetic immunotherapy. The principles of each of these strategies have been illustrated in . There are, however, a few limitations of AAV vectors which need to be addressed for effective HCC gene therapy.
Figure 1. Strategies used for gene therapy of HCC. (A) Restoration of tumor suppressor genes or inhibition of oncogenes may restore normal functioning of the tumor cells. (B) Direct administration of recombinant rAAV expressing toxins or apoptotic factors like TRAIL can lead to tumor cytotoxicity and/or apoptosis. (C) GDEPT is a two-step process to induce tumor cell death. Tumor cells are first transduced with rAAV expressing suicide gene followed by systemic administration of prodrug which is metabolized by the transduced cell into a toxic metabolite. (D) Anti-angiogenic gene therapy using AAV vectors can inhibit formation of new blood vessels, ultimately leading to tumor apoptosis and inhibition of metastasis. (E) Delivery of cytokines and immunomodulatory genes either using AAV vector or immune cells transduced with rAAV vectors harboring cytokines (adoptive immunotherapy) to tumor cells triggers an anti-tumor immune response via recruitment of immune cells.
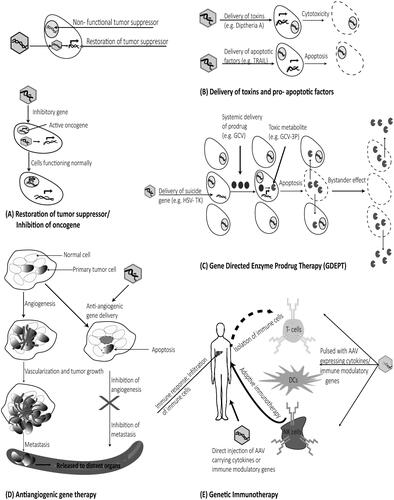
Table 1. Gene therapy studies for HCC using AAV based vectors.
The host immune responses are possibly the biggest obstacle for successful gene therapy; the extent of which depends on the target organ, the route of vector administration, the transgene and the vector itself (Asokan et al., Citation2012). Observations from clinical trials have revealed that immune responses against AAV are comparatively low in immune-privileged organs like the eye and the brain, however, they can be more problematic in other organs such as the liver, muscle or lung (Ferreira et al., Citation2014).
Neutralizing antibodies (NAbs) to the AAV capsid may be a limiting factor to successful gene therapy. The NAbs resulting from natural exposure to wild-type AAV cross-react with AAV vectors after systemic administration. Calcedo et al. have reported that natural exposure to AAV leads to the development of NAbs very early in life (∼2 years) (Calcedo et al., Citation2011). Similar studies to assess the level of preexisting antibodies against AAV vectors have demonstrated that the frequency of antibodies against AAV could be as high as 70% in healthy individuals (Calcedo et al., Citation2009; Boutin et al., Citation2010). Even though the most frequent of these antibodies are directed against AAV serotype 2, cross-reactivity between wide ranges of serotypes due to conserved amino acid sequences in the viral capsid has been reported (Calcedo & Wilson, Citation2013; Gurda et al., Citation2013). These antibodies not only decrease the efficacy of gene therapy by neutralization but also limit the possibility of vector re-administration.
In addition to the NAbs, capsid specific CD8+ T-cell responses have been observed against AAV in clinical trials (Mingozzi & High, Citation2011,Citation2013; George & Fogarty, Citation2016). Results from clinical gene therapy for hemophilia with AAV expressing factor IX (FIX) indicate that the development of capsid specific T-cell response is dependent on the dose of the vector (Manno et al., Citation2006; High & Aubourg, Citation2011; Mingozzi & High, Citation2011,Citation2013; George & Fogarty, Citation2016). Similar capsid-specific T-cells were observed in a clinical trial against lipoprotein lipase deficiency (LPLD) where nine out of 14 subjects who received a mild dose of T-VEC (1 × 1011 vgs/kg) developed T-cell responses to the vector (Ferreira et al., Citation2014). This activation of cellular immunity leads to the destruction of transduced cells and ultimately to a loss of expression of therapeutic gene. This observation was different from preclinical studies in animal models including mice and non-human primates (Manno et al., Citation2006; Mingozzi & High, Citation2013) where T-cell mediated cellular immune response was not observed. Notably, a canine model of hemophilia showed stable and long-term expression of FIX for over nine years (Niemeyer et al., Citation2009; Mingozzi & High, Citation2011). In contrast, clinical trials have reported a correlation between the levels of capsid specific T-cells in peripheral blood and a decrease in the expression of FIX. Interestingly, only a minimal immune response against the therapeutic gene was observed in these trials (Ferreira et al., Citation2014; Arruda & Samelson-Jones, Citation2015; George & Fogarty, Citation2016).
To address the issue of host immune responses against AAV, a number of potential strategies are under development and have been extensively reviewed elsewhere (Masat et al., Citation2013; Mingozzi & High, Citation2013; Arruda & Samelson-Jones, Citation2015; Tse et al., Citation2015; George & Fogarty, Citation2016). Exclusion of patients with high levels of preexisting antibodies against AAV, increasing the vector dose to overcome NAbs, immunosuppression, plasmapheresis and the use of vector decoys are some of the strategies that are currently under investigation (Mingozzi & High, Citation2013; Tse et al., Citation2015). However, none of these strategies provide a long-term solution. The widespread nature of NAbs against AAV in humans and the poor sensitive methods for the detection of antibodies currently make the exclusion strategy impractical (Calcedo et al., Citation2009; Boutin et al., Citation2010; Mingozzi & High, Citation2013). Increasing the dose of vectors and use of decoys may lead to an increase in antigen load and subsequently, the induction of capsid specific T-cell responses (Mingozzi & High, Citation2013; Arruda & Samelson-Jones, Citation2015). Immunosuppression and plasmapheresis are impractical as a long term solutions as they require frequent intervention which is not feasible in HCC patients with poor liver function (Schlitt et al., Citation2011; Tse et al., Citation2015).
The use of AAV vectors which are specific to HCC at both transductional and transcriptional level might be able to overcome many of these obstacles and produce better therapeutic outcomes. First, capsid modification of the vector to increase HCC tropism may lead to a requirement of lower vector dose addressing the issue of cellular immune response against the capsid. Moreover, any capsid specific T-cell response would lead to the clearance of transduced tumor cells. Additionally, identification and mutation of sites in the capsid recognized by NAbs could solve the problem of preexisting immunity and re-administration. Mutations in the capsid of AAV5 have been shown to increase the resistance to NAbs directed against the wildtype virus (Afione et al., Citation2015). Secondly, the use of tumor specific promoters (TSPs) to limit the expression of therapeutic gene in HCC can minimize off-target effects. HCC specific promoters like alpha fetoprotein (AFP) have been used to express therapeutic genes in HCC-specific manner (Su et al., Citation1996; Ma et al., Citation2010). Steel et al. have shown that orally administered AAV5 is distributed predominantly in the liver (Steel et al., Citation2013). The combination of these AAV serotypes displaying a strong liver tropism like AAV8 (Zincarelli et al., Citation2008) with HCC-specific promoters could lead to an enhanced therapeutic gene expression in a HCC-specific manner.
Modification of AAV vectors
Transductional targeting of AAV
With the increase in understanding of vector biology and vector–host interactions, delineation of viral life cycle has led to the elucidation of steps important for viral tropism. Viral binding to cell surface receptor by capsid–receptor interaction is the first step in this process. Identification of sites on the viral capsid which are responsible for binding cellular receptors has made it possible to explore approaches that could ablate the natural broad tropism of the virus and redirect vectors to target cells (Afione et al., Citation2015; Büning et al., Citation2015; Liu et al., Citation2015). Furthermore, the identification of sites in the AAV capsid that can tolerate mutations, insertions and deletions allow for guided genetic capsid manipulation for cell-type specific redirection. The principles and efficacy of modifying viral capsids either by manipulating these sites or by inserting ligands specific to target-cell receptors have been reported for wide variety of cell targets (Kwon & Schaffer, Citation2008; Sallach et al., Citation2014; Büning et al., Citation2015; Liu et al., Citation2015).
Cellular transduction with AAV vectors starts with the binding of the vector at the cell surface (Bartlett et al., Citation2000) which is largely regulated by the capsid of the virus (Vandenberghe et al., Citation2009). The cell and tissue tropism of a particular serotype is dependent on its capsid as evident from the comparison of hepatic transduction efficiencies of six different serotypes (Grimm et al., Citation2006). The broad tropism inherent with AAV vectors is a disadvantage for gene therapy where targeted transgene expression is required. The modification of the vector capsid is, thus, an important area of research for targeted gene therapy.
Different serotypes of AAV exploit varieties of cell surface glycans for attachment to the cell surface, and with about 100 different known serotypes of AAV (Kwon & Schaffer, Citation2008), complete delineation of tropism and cell surface receptor for each of these serotypes remains an exciting field of investigation. AAV2, the most widely used AAV serotype for gene therapy, primarily uses cell surface heparan sulfate proteoglycan (HSPG) which is expressed in different cell types (Summerford & Samulski, Citation1998). AAV2 has been shown to efficiently transduce varieties of cells and tissues including hepatocytes, airway epithelium, cardiac muscle, skeletal muscle and brain tissue (Michelfelder & Trepel, Citation2009). In preclinical studies, liver tropism has been reported for AAV serotypes 5 (Davidoff et al., Citation2005; Steel et al., Citation2013), 6 (Jiang et al., Citation2006), 8 (Davidoff et al., Citation2005; Gao et al., Citation2006) and 9 (Sarkar et al., Citation2006). Interestingly, AAV serotype 3, which has been shown to have weak tropism for liver (Zincarelli et al., Citation2008; Cheng et al., Citation2012), exhibited strong tropism for HCC cells (Cheng et al., Citation2012) due in part to hepatocyte growth factor receptor (HGFR), a co-receptor for AAV3 (Ling et al., Citation2010), being overexpressed on HCC (Luo et al., Citation1999; Cheng et al., Citation2012). Exploiting this natural tropism of certain serotypes for HCC-specific transgene expression is a potential strategy of targeted gene therapy as shown by the efficacy of suicide gene therapy of disseminated HCC by AAV8 harboring a liver-specific promoter in combination with a HCC-specific miR122a binding sequence (Della Peruta et al., Citation2015).
Pseudotyping the genome of one AAV serotype into the capsid of another is another strategy that has been employed to achieve cell specific gene expression. A study conducted by Grimm et al. demonstrated that the capsid and not the genome is responsible for liver tropism, and provides the rationale for pseudotyping (Grimm et al., Citation2006). An enhanced expression of transgene using pseudotyped vectors have been reported for different cells and organs including skeletal muscle (Chao et al., Citation2000), dermal fibroblasts (Balaji et al., Citation2013), neurons (Alisky et al., Citation2000), eye (Auricchio, Citation2003), heart (Pacak et al., Citation2006) and more importantly in the liver (Grimm et al., Citation2003). The genome of AAV2 is the most widely studied and engineered of all the serotypes. It has been used for cell-specific therapeutic gene expression using regulatory elements like promoters and enhancers (Weitzman & Linden, Citation2011; Balakrishnan & Jayandharan, Citation2014). AAV2 genome containing therapeutic gene under the control of HCC-specific regulatory elements could potentially be pseudotyped with serotypes like AAV3 which have greater HCC-tropism. Another potential strategy is the generation of mosaic or chimeric capsid by mixing capsid subunits from different serotypes. AAV vectors with chimeric capsids have been reported to have enhanced transduction in endothelial cells (Stachler & Bartlett, Citation2006), muscle and liver (Hauck et al., Citation2003) when compared to parental serotypes.
These strategies, however, fail to address the obstacles posed by the host immune response (Waehler et al., Citation2007; Michelfelder & Trepel, Citation2009) and even if an enhanced HCC-cell transduction is achieved, the issue with general broad tropism of any new serotype would still remain a concern. In addition, the use of pseudotyped and chimeric vectors is limited by the availability of AAV serotypes with natural HCC-tropism. Targeting AAV using HCC-specific ligands is one strategy that might address some of these unresolved problems. The discovery of ligands which specifically bind to HCC (Du et al., Citation2006; Lo et al., Citation2008) will facilitate this process. This ligand-receptor mediated cell targeting can be achieved either by genetic modification of the capsid or by non-genetic means (Waehler et al., Citation2007; Sen et al., Citation2013; Sen, Citation2014).
Non-genetic means of capsid targeting can be achieved with adapter proteins such as bispecific antibodies which can bind to both viral capsid and the target cell (Waehler et al., Citation2007). To transduce megakaryocyte cells which are non-permissive to AAV, a bispecific antibody interacting with both the viral capsid and cell-specific receptor has been tested, and demonstrated a reduction of natural tropism of the virus and increase in selectivity of 70-fold (Bartlett et al., Citation1999). An alternate strategy is the genetic modification of the capsid that facilitates non-genetic targeting of the vector, for example, the insertion of the immunoglobulin binding domain of protein A into the capsid which binds of the Fc region of an antibody while allowing the antibody to retain its targeting motif. This method of targeting has been used in AAV to improve transduction of hematopoietic cell lines, where transduction was dependent on the binding of the inserted domain with cell surface antibodies (Ried et al., Citation2002). Similarly, biophysical probes and targeting ligands have been conjugated in a site-specific manner to biotin accepter peptide (BAP)-modified AAV using the biotin ligase enzyme. The transduction efficiency of the BAP-modified vector with integrin targeting peptide was found to be significantly higher in endothelial cells compared to the wild-type and an enhanced ability to deliver reporter gene to the tumor vasculature was observed in a mouse model of ovarian cancer (Stachler et al., Citation2008). Non-genetic targeting of AAV capsid in this way for HCC itself has not yet been reported. These methods do have limitations of technical complexity, destabilization of the vector complex, and difficulty of high-scale vector production (Büning et al., Citation2015; Liu et al., Citation2015).
With the knowledge of molecular structure of the virus capsid (Xie et al., Citation2002) and identification of sites responsible for interaction with the receptor (Kern et al., Citation2003; Opie et al., Citation2003; Afione et al., Citation2015), genetic insertion of HCC-specific ligands at sites which tolerate manipulation is possible. depicts the principles of this possible method of manufacturing HCC-targeted AAV vectors.
Figure 2. Capsid modification of AAV by insertion of HCC-binding ligands. Insertion of HCC-binding peptides at sites of AAV capsid which tolerate insertions without affecting viral life cycle can change the natural tropism of the vector and retarget it to HCC.

The first study identifying potential sites in AAV2 which could facilitate peptide insertion without disrupting viral life cycle was conducted by Girod et al. Insertion of a L14 peptide which binds to the laminin receptor at amino acid 587 was found to change the natural tropism of the vector in an L14 dependent manner (Girod et al., Citation1999). Melanoma cell line B16F1025, which is non-permissive to wild-type AAV virus, was efficiently transduced with the mutant (Girod et al., Citation1999). Similarly, an enhanced and ligand specific endothelia cell transduction was observed when endothelial-specific ligand was inserted at the same site (Nicklin et al., Citation2001). For Kaposi sarcoma and rhabdomyosarcoma cell lines which express high levels of CD13, a binding ligand was inserted at amino acid position 587 to achieve cell-specific transduction (Grifman et al., Citation2001). In another study, Wu et al. identified additional sites in the AAV2 capsid which tolerated insertion. Insertion of peptide specific to serpin receptor at positions 34 and 138 led to 62 and 15-fold increase in transduction for IB3 cells respectively (Wu et al., Citation2000). Similarly, an increase in the transduction of breast cancer tissues was observed after insertion of peptide ESGLSQS at positions 590 and 589 of AAV8 and AAV9, respectively (Michelfelder et al., Citation2011). As an alternative to genetic insertion of cell-specific peptides, the development of AAV libraries based on capsid DNA shuffling, error prone PCR and directed evolution of known AAV capsid serotypes are being developed (Kotterman & Schaffer, Citation2014). The incorporation of designed ankyrin repeat protein (DARPins) libraries into the capsid has also been examined to target AAV. An ankyrin repeat protein 9.29 which binds with high affinity to human epidermal growth factor receptor/neu (HER2/neu), a receptor tyrosine kinase overexpressed in tumor cells has been fused with AAV capsid to ablate the natural tropism and re-direct the vector to HER2 positive tumor cells (Münch et al., Citation2013). The principles and relative merits and demerits of these strategies have been reviewed in a number of publications (Vandenberghe et al., Citation2009; Kotterman & Schaffer, Citation2014; Büning et al., Citation2015; Liu et al., Citation2015). These methods can not only increase the cell-specific transduction with AAV but can also generate vectors which can evade host immune responses (Maheshri et al., Citation2006; Li et al., Citation2016).
Masheri et al. constructed mutant vectors using capsid mutant library generated by error prone PCR. These vectors were subsequently incubated with pre-immunized rabbit sera and found to have an enhanced resistance to preexisting NAbs compared to the wild-type vector in vivo (Maheshri et al., Citation2006). More recently, a novel approach to generate patient-specific NAb escaping AAV mutants has been reported for muscle gene delivery (Li et al., Citation2016). In this study, NAb escaping AAVs were isolated from patients who had received AAV harboring mini-dystrophin gene during the Phase I clinical trial of Duchenne muscular dystrophy (DMD). The in vivo selection of these mutants resulted in isolation of AAVs that displayed high muscle tropism and superior ability to evade host immune responses (Li et al., Citation2016). For manufacturing AAV vectors with high tropism for liver, Lisowski et al. created and screened AAV capsid mutant library from 10 different serotypes (Lisowski et al., Citation2014). One of the chimeras composed of five different parental capsids displayed improved hepatocyte transduction efficiency. In addition, this mutant was able to transduce HCC cells in culture and in a xenograft model at higher efficiencies compared to any wild type serotype (Lisowski et al., Citation2014). These studies have demonstrated the possibility of integrating capsid modification techniques for the manufacture of clinically relevant AAV vectors to deliver therapeutic genes against HCC.
Transcriptional targeting of AAV
The ablation of natural tropism and retargeting AAV to HCC by capsid modification has the potential to overcome many of the obstacles for successful gene therapy, however, a complete ablation of viral tropism is highly unlikely in practice, and therefore of equal importance is the transcriptional targeting of AAV to maintain target-cell specific therapeutic gene expression to limit off-target effects and toxicity to normal cells. A tightly regulated HCC-specific expression of therapeutic gene can minimize any off-target effects and toxicity of normal cells. With the ease of manipulation of AAV genome, transcription targeting of AAV with HCC-specific promoters can be used for this purpose.
During the process of malignant transformation there are significant changes in the expression of many genes induced by genetic and epigenetic factors. The changes in gene expression can range from over expression of native proteins (e.g. Epidermal Growth Factor Receptor (EGFR), Survivin), mutated proteins (e.g. p53) to reactivation of oncofetal proteins (e.g. AFP, Glypican 3 (GPC3)) resulting in phenotypic as well as the genotypic changes in the cancer. In HCC, there are a number of genes that are reported to be commonly over expressed including: AFP expressed in 86% of HCC; GPC3 expressed in 83–90% of HCC (Kandil & Cooper, Citation2009) and Survivin expressed in up to 90% of HCC (Nassar et al., Citation2009).
The expression of a gene is controlled by a complex interplay of molecular factors that are active in a cell specific manner and show differential activity in response to environmental changes like tumor related hypoxia. Promoters are cis-acting elements generally present at the 5′-end of a gene of interest. Active promoters provide a binding site for transcription factors to bring about efficient gene expression (Forrest et al., Citation2014).
In order to prevent expression of therapeutic transgenes in non-HCC cells and limit toxicity in normal cells, targeting at the level of transcription with these TSPs can be used (; Robson & Hirst, Citation2003). The proof of efficacy of transcriptional targeting using TSPs has been documented for different cancer types including prostate cancer (Figueiredo et al., Citation2006; Coulter et al., Citation2010), breast cancer (Li et al., Citation2005), colorectal cancer (Li et al., Citation2009), non-small cell lung cancer (Pasini et al., Citation2015), melanoma (Lu et al., Citation2005) and ovarian cancer (Casado et al., Citation2001) using adenoviral vectors.
Figure 3. Transcriptional targeting with HCC-specific promoters. Tumor specific promoters are selectively active in cancer cells and are able to regulate the expression of therapeutic gene in a cancer specific manner. The identification and use of HCC-specific promoters could minimize off-target effects by limiting the expression of therapeutic gene in HCC.
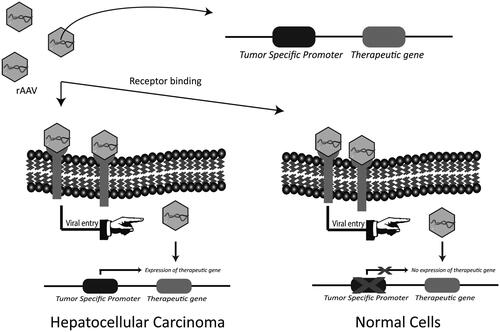
The first reported gene therapy for HCC using AAV vector was performed using an AFP promoter to drive the expression of HSV-TK to selectively kill HCC (Su et al., Citation1996). AFP is an oncofetal protein overexpressed in HCC and commonly used as a diagnostic marker (Forner et al., Citation2012). The use of AFP to selectively kill AFP-positive cells was later reproduced in human xenograft cancers in an athymic mouse model (Su et al., Citation1997). In similar studies, the human telomerase reverse transcriptase (hTERT) promoter, active in about 90% of cancer types including HCC (Kim et al., Citation1994; Iliopoulos et al., Citation2009), has been used for targeted cancer therapy (Abdul-Ghani et al., Citation2000; Gu & Fang, Citation2003). An AAV vector expressing pro-apoptotic factor TRAIL under the control of hTERT promoter was shown to be efficient in killing HCC both in vitro and in vivo. Moreover, minimal transgene expression was reported in normal cells including hepatocytes (Zhang et al., Citation2008; Wang et al., Citation2010). Survivin is another cancer specific promoter which has been used for suicide gene therapy of HCC cells (Qu et al., Citation2013). In another approach for transcriptional targeting, liver specific apoE alpha-1-antitrypsin (apoE-AAT) promoter was combined with cell-cycle-dependent elements (CDE) and cell-cycle genes homology regions (CHR) to achieve HCC-specific expression of luciferase (Sia et al., Citation2013). microRNA has also been used to transcriptionally target HCC. The binding region of HCC-specific miR122a was combined with a liver specific promoter in order to achieve HCC-specific HSV-TK expression mediated by AAV8 (Della Peruta et al., Citation2015). In another study, apoE-AAT was combined with sequences of miR122a for transcriptional targeting of HCC (Fu et al., Citation2012).
Given the heterogeneity of HCC, the identification of new or multiple transcriptional targets for gene therapy may be required. This may be achieved by screening and identification of active promoters, not functional in normal cells, through the use of publically available microarray data and/or though deep sequencing of patient biopsy samples (Foka et al., Citation2010). Finally, the combination of these two strategies, i.e. transductional and transcriptional targeting could act synergistically and enhance therapeutic outcomes compared to targeting with one approach alone. Reynolds et al. reported a 300 000-fold increase in the selectivity of transgene expression using adenovirus in lung after combinatorial vector targeting (Reynolds et al., Citation2001). The possibility and efficacy of combining these two strategies have been demonstrated with adenoviral vectors in colorectal cancer (Li et al., Citation2009) and ovarian cancer (Barker et al., Citation2003).
Discussions
Developing effective therapies for HCC requires that we face both the biological and practical constraints of treating this disease. HCC develops in an environment of liver disease and is often characterized by cirrhosis and poor liver function. Treatments that are highly effective in killing the tumor may not be clinically appropriate in this setting, particularly if it leads to increased liver toxicity. Targeting treatments specifically to the HCC while sparing the normal/diseased liver may be required for an effective therapy for HCC. Gene therapy, which aims at correcting diseases at the genetic level, may be an alternative to conventional treatments. However, the success of gene therapy depends not only on developing a potent anti-cancer therapeutic strategy but potentially and maybe more importantly, on developing a vector to efficiently and safely deliver the therapeutic transgene.
AAV is arguably the best gene delivery vector at our disposal today. Numerous preclinical and clinical trials have shown the vector to have limited toxicity, minimal immune activation to the transgene and an ability to transduce a wide range of dividing and non-dividing cells to maintain long-term, stable transgene expression. However, these clinical trials have identified a few limitations of AAV vectors which need to be resolved for therapeutic benefits. Moreover, most of the AAV serotypes have a natural tropism for liver which presents a problem when targeting of HCC is required.
Fortunately, the current knowledge of AAV biology has identified key binding sites on the viral capsid and many of the cellular receptors which allow viral entry. Modification of AAV capsid without disruption of its ability to form an intact vector is one of the most promising areas of research currently under development. Using this strategy, AAV vectors with altered tropism and increased specificity for a number of different cell lines have been designed. These reports of transductional targeting of AAV suggest that similar techniques could be used for HCC in order to generate HCC targeted vectors. An additional layer of HCC specificity could be achieved by selecting tumor-specific promoters, specifically active in HCC, to drive the expression of the therapeutic transgene. This method of transcriptional targeting is highly likely to limit the off-target therapeutic gene expression.
The combination of both of these targeting strategies has the potential to overcome many of the limitations of AAV based gene delivery. First, the vector dose required to achieve therapeutic levels of transgene could be significantly lowered as most virus will transduce the tumor thereby limiting the potential for the development of capsid specific T-cell responses. Moreover, the capsid modified vectors have the potential to escape the preexisting NAbs and specifically transduce HCC while avoiding entry in the normal cells which could be of special interest in patients with liver cirrhosis. Finally, the off-target effects could be significantly minimized by using the TSPs in combination with capsid targeting. As more novel and reliable ligands and promoters, specific for HCC, are identified, the prospect of developing rationally designed targeted AAV vectors for HCC becomes closer to reality.
Declaration of interest
The authors have no affiliations or conflict of interest with any individual or organization regarding any financial interest in the subject matter that has been discussed in this manuscript.
Funding
This work was supported by the Gallipoli Medical Research Foundation.
References
- Abdul-Ghani R, Ohana P, Matouk I, et al. (2000). Use of transcriptional regulatory sequences of telomerase (hTER and hTERT) for selective killing of cancer cells. Mol Ther 2:539–44
- Afione S, Dimattia MA, Halder S, et al. (2015). Identification and mutagenesis of the adeno-associated virus 5 sialic acid binding region. J Virol 89:1660–72
- Alisky JM, Hughes SM, Sauter SL, et al. (2000). Transduction of murine cerebellar neurons with recombinant FIV and AAV5 vectors. Neuroreport 11:2669–73
- Amer MH. (2014). Gene therapy for cancer: present status and future perspective. Mol Cell Ther 2:27
- Arruda VR, Samelson-Jones BJ. (2015). Obstacles and future of gene therapy for hemophilia. Expert Opin Orphan Drugs 3:997–1010
- Asokan A, Schaffer DV, Samulski RJ. (2012). The AAV vector toolkit: poised at the clinical crossroads. Mol Ther 20:699–708
- Auricchio A. (2003). Pseudotyped AAV vectors for constitutive and regulated gene expression in the eye. Vision Res 43:913–18
- Balaji S, King A, Dhamija Y, et al. (2013). Pseudotyped adeno-associated viral vectors for gene transfer in dermal fibroblasts: implications for wound-healing applications. J Surg Res 184:691–8
- Balakrishnan B, Jayandharan GR. (2014). Basic biology of adeno-associated virus (AAV) vectors used in gene therapy. Curr Gene Ther 14:86–100
- Barker SD, Dmitriev IP, Nettelbeck DM, et al. (2003). Combined transcriptional and transductional targeting improves the specificity and efficacy of adenoviral gene delivery to ovarian carcinoma. Gene Ther 10:1198–204
- Bartlett JS, Kleinschmidt J, Boucher RC, Samulski RJ. (1999). Targeted adeno-associated virus vector transduction of nonpermissive cells mediated by a bispecific F(ab′gamma)2 antibody. Nat Biotechnol 17:181–6
- Bartlett JS, Wilcher R, Samulski RJ. (2000). Infectious entry pathway of adeno-associated virus and adeno-associated virus vectors. J Virol 74:2777–85
- Boutin S, Monteilhet V, Veron P, et al. (2010). Prevalence of serum IgG and neutralizing factors against adeno-associated virus (AAV) types 1, 2, 5, 6, 8, and 9 in the healthy population: implications for gene therapy using AAV vectors. Hum Gene Ther 21:704–12
- Büning H, Huber A, Zhang L, et al. (2015). Engineering the AAV capsid to optimize vector–host-interactions. Curr Opin Pharmacol 24:94–104
- Calcedo R, Morizono H, Wang L, et al. (2011). Adeno-associated virus antibody profiles in newborns, children, and adolescents. Clin Vaccine Immunol 18:1586–8
- Calcedo R, Vandenberghe LH, Gao G, et al. (2009). Worldwide epidemiology of neutralizing antibodies to adeno-associated viruses. J Infect Dis 199:381–90
- Calcedo R, Wilson JM. (2013). Humoral immune response to AAV. Front Immunol 4:341
- Casado E, Nettelbeck DM, Gomez-Navarro J, et al. (2001). Transcriptional targeting for ovarian cancer gene therapy. Gynecol Oncol 82:229–37
- Chang CM, Lo CH, Shih YM, et al. (2010). Treatment of hepatocellular carcinoma with adeno-associated virus encoding interleukin-15 superagonist. Hum Gene Ther 21:611–21
- Chao H, Liu Y, Rabinowitz J, et al. (2000). Several log increase in therapeutic transgene delivery by distinct adeno-associated viral serotype vectors. Mol Ther 2:619–23
- Chen CA, Lo CK, Lin BL, et al. (2008). Application of doxorubicin-induced rAAV2-p53 gene delivery in combined chemotherapy and gene therapy for hepatocellular carcinoma. Cancer Biol Ther 7:303–9
- Cheng AL, Kang YK, Chen Z, et al. (2009). Efficacy and safety of sorafenib in patients in the Asia-Pacific region with advanced hepatocellular carcinoma: a phase III randomized, double-blind, placebo-controlled trial. Lancet Oncol 10:25–34
- Cheng B, Ling C, Dai Y, et al. (2012). Development of optimized AAV3 serotype vectors: mechanism of high-efficiency transduction of human liver cancer cells. Gene Ther 19:375–84
- Coulter JA, Page NL, Worthington J, et al. (2010). Transcriptional regulation of inducible nitric oxide synthase gene therapy: targeting early stage and advanced prostate cancer. J Gene Med 12:755–65
- Davidoff AM, Gray JT, Ng CY, et al. (2005). Comparison of the ability of adeno-associated viral vectors pseudotyped with serotype 2, 5, and 8 capsid proteins to mediate efficient transduction of the liver in murine and nonhuman primate models. Mol Ther 11:875–88
- Della Peruta M, Badar A, Rosales C, et al. (2015). Preferential targeting of disseminated liver tumors using a recombinant adeno-associated viral vector. Hum Gene Ther 26:94–103
- Du B, Qian M, Zhou Z, et al. (2006). In vitro panning of a targeting peptide to hepatocarcinoma from a phage display peptide library. Biochem Biophys Res Commun 342:956–62
- Du WZ, Yu TX. (2011). Generation of antitumor response against hepatocellular carcinoma by in vitro transduction of dendritic cells with adeno-associated virus expressing α-fetoprotein. Zhonghua Yi Xue Za Zhi 91:2077–80
- El-Serag HB, Rudolph KL. (2007). Hepatocellular carcinoma: epidemiology and molecular carcinogenesis. Gastroenterology 132:2557–76
- Ferreira V, Petry H, Salmon F. (2014). Immune responses to AAV-vectors, the Glybera example from bench to bedside. Front Immunol 5:82
- Figueiredo ML, Sato M, Johnson M, Wu L. (2006). Specific targeting of gene therapy to prostate cancer using a two-step transcriptional amplification system. Future Oncol 2:391–406
- Flaherty KT, Sun W. (2009). Which questions remain unanswered following the successful development of sorafenib in hepatocellular carcinoma? Nat Clin Pract Oncol 6:64–5
- Foka P, Pourchet A, Hernandez-Alcoceba R, et al. (2010). Novel tumor-specific promoters for transcriptional targeting of hepatocellular carcinoma by herpes simplex virus vectors. J Gene Med 12:956–67
- Forner A, Llovet JM, Bruix J. (2012). Hepatocellular carcinoma. Lancet 379:1245–55
- Forrest AR, Kawaji H, Rehli M, et al. (2014). A promoter-level mammalian expression atlas. Nature 507:462–70
- Fu X, Rivera A, Tao L, et al. (2012). Construction of an oncolytic herpes simplex virus that precisely targets hepatocellular carcinoma cells. Mol Ther 20:339–46
- Gao G, Lu Y, Calcedo R, et al. (2006). Biology of AAV serotype vectors in liver-directed gene transfer to nonhuman primates. Mol Ther 13:77–87
- George LA, Fogarty PF. (2016). Gene therapy for hemophilia: past, present and future. Semin Hematol 53:46–54
- Girod A, Ried M, Wobus C, et al. (1999). Genetic capsid modifications allow efficient re-targeting of adeno-associated virus type 2. Nat Med 5:1052–6
- Grifman M, Trepel M, Speece P, et al. (2001). Incorporation of tumor-targeting peptides into recombinant adeno-associated virus capsids. Mol Ther 3:964–75
- Grimm D, Pandey K, Nakai H, et al. (2006). Liver transduction with recombinant adeno-associated virus is primarily restricted by capsid serotype not vector genotype. J Virol 80:426–39
- Grimm D, Zhou S, Nakai H, et al. (2003). Preclinical in vivo evaluation of pseudotyped adeno-associated virus vectors for liver gene therapy. Blood 102:2412–9
- Gu J, Fang B. (2003). Telomerase promoter-driven cancer gene therapy. Cancer Biol Ther 2:S64–70
- Gurda BL, Dimattia MA, Miller EB, et al. (2013). Capsid antibodies to different adeno-associated virus serotypes bind common regions. J Virol 87:9111–24
- Hauck B, Chen L, Xiao W. (2003). Generation and characterization of chimeric recombinant AAV vectors. Mol Ther 7:419–25
- High KA, Aubourg P. (2011). rAAV human trial experience. Methods Mol Biol 807:429–57
- Hong SY, Lee MH, Kim KS, et al. (2004). Adeno-associated virus mediated endostatin gene therapy in combination with topoisomerase inhibitor effectively controls liver tumor in mouse model. World J Gastroenterol 10:1191–7
- Iliopoulos D, Satra M, Drakaki A, et al. (2009). Epigenetic regulation of hTERT promoter in hepatocellular carcinomas. Int J Oncol 34:391–9
- Jiang H, Lillicrap D, Patarroyo-White S, et al. (2006). Multiyear therapeutic benefit of AAV serotypes 2, 6, and 8 delivering factor VIII to hemophilia A mice and dogs. Blood 108:107–15
- Kandil DH, Cooper K. (2009). Glypican-3: a novel diagnostic marker for hepatocellular carcinoma and more. Adv Anat Pathol 16:125–9
- Kern A, Schmidt K, Leder C, et al. (2003). Identification of a heparin-binding motif on adeno-associated virus type 2 capsids. J Virol 77:11072–81
- Kim NW, Piatyszek MA, Prowse KR, et al. (1994). Specific association of human telomerase activity with immortal cells and cancer. Science 266:2011–15
- Kota J, Chivukula RR, O'donnell KA, et al. (2009). Therapeutic microRNA delivery suppresses tumorigenesis in a murine liver cancer model. Cell 137:1005–17
- Kotterman MA, Schaffer DV. (2014). Engineering adeno-associated viruses for clinical gene therapy. Nat Rev Genet 15:445–51
- Kwon I, Schaffer DV. (2008). Designer gene delivery vectors: molecular engineering and evolution of adeno-associated viral vectors for enhanced gene transfer. Pharm Res 25:489–99
- Lee K, Yun ST, Kim YG, et al. (2006). Adeno-associated virus-mediated expression of apolipoprotein (a) kringles suppresses hepatocellular carcinoma growth in mice. Hepatology 43:1063–73
- Li C, Wu S, Albright B, et al. (2016). Development of patient-specific AAV vectors after neutralizing antibody selection for enhanced muscle gene transfer. Mol Ther 24:53–65
- Li HJ, Everts M, Yamamoto M, et al. (2009). Combined transductional untargeting/retargeting and transcriptional restriction enhances adenovirus gene targeting and therapy for hepatic colorectal cancer tumors. Cancer Res 69:554–64
- Li X, Zhang J, Gao H, et al. (2005). Transcriptional targeting modalities in breast cancer gene therapy using adenovirus vectors controlled by alpha-lactalbumin promoter. Mol Cancer Ther 4:1850–9
- Liang CM, Zhong CP, Sun RX, et al. (2007). Local expression of secondary lymphoid tissue chemokine delivered by adeno-associated virus within the tumor bed stimulates strong anti-liver tumor immunity. J Virol 81:9502–11
- Ling C, Lu Y, Kalsi JK, et al. (2010). Human hepatocyte growth factor receptor is a cellular coreceptor for adeno-associated virus serotype 3. Hum Gene Ther 21:1741–7
- Lisowski L, Dane AP, Chu K, et al. (2014). Selection and evaluation of clinically relevant AAV variants in a xenograft liver model. Nature 506:382–6
- Liu Y, Siriwon N, Rohrs JA, Wang P. (2015). Generation of targeted adeno-associated virus (AAV) vectors for human gene therapy. Curr Pharm Des 21:3248–56
- Liver EAFTSOT, Cancer EOFRATO. (2012). EASL-EORTC clinical practice guidelines: management of hepatocellular carcinoma. J Hepatol 56:908–43
- Llovet JM, Ricci S, Mazzaferro V, et al. (2008). Sorafenib in advanced hepatocellular carcinoma. N Engl J Med 359:378–90
- Lo A, Lin CT, Wu HC. (2008). Hepatocellular carcinoma cell-specific peptide ligand for targeted drug delivery. Mol Cancer Ther 7:579–89
- Lo CH, Chang CM, Tang SW, et al. (2010). Differential antitumor effect of interleukin-12 family cytokines on orthotopic hepatocellular carcinoma. J Gene Med 12:423–34
- Lu B, Makhija SK, Nettelbeck DM, et al. (2005). Evaluation of tumor-specific promoter activities in melanoma. Gene Ther 12:330–8
- Luo J, Luo Y, Sun J, et al. (2015). Adeno-associated virus-mediated cancer gene therapy: current status. Cancer Lett 356:347–56
- Luo YQ, Wu MC, Cong WM. (1999). Gene expression of hepatocyte growth factor and its receptor in HCC and nontumorous liver tissues. World J Gastroenterol 5:119–21
- Ma H, Liu Y, Liu S, et al. (2005a). Recombinant adeno-associated virus-mediated TRAIL gene therapy suppresses liver metastatic tumors. Int J Cancer 116:314–21
- Ma H, Liu Y, Liu S, et al. (2005b). Oral adeno-associated virus-sTRAIL gene therapy suppresses human hepatocellular carcinoma growth in mice. Hepatology 42:1355–63
- Ma SH, Chen GG, Yip J, Lai PB. (2010). Therapeutic effect of alpha-fetoprotein promoter-mediated tBid and chemotherapeutic agents on orthotopic liver tumor in mice. Gene Ther 17:905–12
- Maheshri N, Koerber JT, Kaspar BK, Schaffer DV. (2006). Directed evolution of adeno-associated virus yields enhanced gene delivery vectors. Nat Biotechnol 24:198–204
- Manno CS, Pierce GF, Arruda VR, et al. (2006). Successful transduction of liver in hemophilia by AAV-factor IX and limitations imposed by the host immune response. Nat Med 12:342–7
- Masat E, Pavani G, Mingozzi F. (2013). Humoral immunity to AAV vectors in gene therapy: challenges and potential solutions. Discov Med 15:379–89
- Michelfelder S, Trepel M. (2009). Adeno-associated viral vectors and their redirection to cell-type specific receptors. Adv Genet 67:29–60
- Michelfelder S, Varadi K, Raupp C, et al. (2011). Peptide ligands incorporated into the threefold spike capsid domain to re-direct gene transduction of AAV8 and AAV9 in vivo. PLoS One 6:e23101
- Mingozzi F, High KA. (2011). Immune responses to AAV in clinical trials. Curr Gene Ther 11:321–30
- Mingozzi F, High KA. (2013). Immune responses to AAV vectors: overcoming barriers to successful gene therapy. Blood 122:23–36
- Münch RC, Janicki H, Völker I, et al. (2013). Displaying high-affinity ligands on adeno-associated viral vectors enables tumor cell-specific and safe gene transfer. Mol Ther 21:109–18
- Nassar A, Cohen C, Siddiqui MT. (2009). Utility of glypican-3 and survivin in differentiating hepatocellular carcinoma from benign and preneoplastic hepatic lesions and metastatic carcinomas in liver fine-needle aspiration biopsies. Diagn Cytopathol 37:629–35
- Nicklin SA, Buening H, Dishart KL, et al. (2001). Efficient and selective AAV2-mediated gene transfer directed to human vascular endothelial cells. Mol Ther 4:174–81
- Niemeyer GP, Herzog RW, Mount J, et al. (2009). Long-term correction of inhibitor-prone hemophilia B dogs treated with liver-directed AAV2-mediated factor IX gene therapy. Blood 113:797–806
- Opie SR, Warrington KH, Agbandje-Mckenna M, et al. (2003). Identification of amino acid residues in the capsid proteins of adeno-associated virus type 2 that contribute to heparan sulfate proteoglycan binding. J Virol 77:6995–7006
- Pacak CA, Mah CS, Thattaliyath BD, et al. (2006). Recombinant adeno-associated virus serotype 9 leads to preferential cardiac transduction in vivo. Circ Res 99:e3–9
- Pasini A, Delmonte A, Tesei A, et al. (2015). Targeting chromatin-mediated transcriptional control of gene expression in non-small cell lung cancer therapy: preclinical rationale and clinical results. Drugs 75:1757–71
- Peng D, Qian C, Sun Y, et al. (2000). Transduction of hepatocellular carcinoma (HCC) using recombinant adeno-associated virus (rAAV): in vitro and in vivo effects of genotoxic agents. J Hepatol 32:975–85
- Qu L, Wang Y, Gong L, et al. (2013). Suicide gene therapy for hepatocellular carcinoma cells by survivin promoter-driven expression of the herpes simplex virus thymidine kinase gene. Oncol Rep 29:1435–40
- Reynolds PN, Nicklin SA, Kaliberova L, et al. (2001). Combined transductional and transcriptional targeting improves the specificity of transgene expression in vivo. Nat Biotechnol 19:838–42
- Ried MU, Girod A, Leike K, et al. (2002). Adeno-associated virus capsids displaying immunoglobulin-binding domains permit antibody-mediated vector retargeting to specific cell surface receptors. J Virol 76:4559–66
- Robson T, Hirst DG. (2003). Transcriptional targeting in cancer gene therapy. J Biomed Biotechnol 2003:110–37
- Sallach J, Di Pasquale G, Larcher F, et al. (2014). Tropism-modified AAV vectors overcome barriers to successful cutaneous therapy. Mol Ther 22:929–39
- Sarkar R, Mucci M, Addya S, et al. (2006). Long-term efficacy of adeno-associated virus serotypes 8 and 9 in hemophilia a dogs and mice. Hum Gene Ther 17:427–39
- Schlitt HJ, Mornex F, Shaked A, Trotter JF. (2011). Immunosuppression and hepatocellular carcinoma. Liver Transpl 17 Suppl 2:S159–61
- Sen D. (2014). Improving clinical efficacy of adeno associated vectors by rational capsid bioengineering. J Biomed Sci 21:103
- Sen D, Balakrishnan B, Gabriel N, et al. (2013). Improved adeno-associated virus (AAV) serotype 1 and 5 vectors for gene therapy. Sci Rep 3:1832
- Shen Z, Wong OG, Yao RY, et al. (2008a). A novel and effective hepatocyte growth factor kringle 1 domain and p53 cocktail viral gene therapy for the treatment of hepatocellular carcinoma. Cancer Lett 272:268–76
- Shen Z, Yang ZF, Gao Y, et al. (2008b). The kringle 1 domain of hepatocyte growth factor has antiangiogenic and antitumor cell effects on hepatocellular carcinoma. Cancer Res 68:404–14
- Sia KC, Huynh H, Chung AY, et al. (2013). Preclinical evaluation of transcriptional targeting strategy for human hepatocellular carcinoma in an orthotopic xenograft mouse model. Mol Cancer Ther 12:1651–64
- Siegel RL, Miller KD, Jemal A. (2015). Cancer statistics, 2015. CA Cancer J Clin 65:5–29
- Stachler MD, Bartlett JS. (2006). Mosaic vectors comprised of modified AAV1 capsid proteins for efficient vector purification and targeting to vascular endothelial cells. Gene Ther 13:926–31
- Stachler MD, Chen I, Ting AY, Bartlett JS. (2008). Site-specific modification of AAV vector particles with biophysical probes and targeting ligands using biotin ligase. Mol Ther 16:1467–73
- Steel JC, Di Pasquale G, Ramlogan CA, et al. (2013). Oral vaccination with adeno-associated virus vectors expressing the Neu oncogene inhibits the growth of murine breast cancer. Mol Ther 21:680–7
- Su H, Chang JC, Xu SM, Kan YW. (1996). Selective killing of AFP-positive hepatocellular carcinoma cells by adeno-associated virus transfer of the herpes simplex virus thymidine kinase gene. Hum Gene Ther 7:463–70
- Su H, Lu R, Chang JC, Kan YW. (1997). Tissue-specific expression of herpes simplex virus thymidine kinase gene delivered by adeno-associated virus inhibits the growth of human hepatocellular carcinoma in athymic mice. Proc Natl Acad Sci USA 94:13891–6
- Summerford C, Samulski RJ. (1998). Membrane-associated heparan sulfate proteoglycan is a receptor for adeno-associated virus type 2 virions. J Virol 72:1438–45
- Torrecilla S, Llovet JM. (2015). New molecular therapies for hepatocellular carcinoma. Clin Res Hepatol Gastroenterol. 39(Suppl 1):S80–S85
- Tse LV, Moller-Tank S, Asokan A. (2015). Strategies to circumvent humoral immunity to adeno-associated viral vectors. Expert Opin Biol Ther 15:845–55
- Tse LY, Sun X, Jiang H, et al. (2008). Adeno-associated virus-mediated expression of kallistatin suppresses local and remote hepatocellular carcinomas. J Gene Med 10:508–17
- Vandenberghe LH, Wilson JM, Gao G. (2009). Tailoring the AAV vector capsid for gene therapy. Gene Ther 16:311–9
- Vanrell L, Di Scala M, Blanco L, et al. (2011). Development of a liver-specific Tet-on inducible system for AAV vectors and its application in the treatment of liver cancer. Mol Ther 19:1245–53
- Waehler R, Russell SJ, Curiel DT. (2007). Engineering targeted viral vectors for gene therapy. Nat Rev Genet 8:573–87
- Waghray A, Murali AR, Menon KN. (2015). Hepatocellular carcinoma: from diagnosis to treatment. World J Hepatol 7:1020–9
- Wang Y, Huang F, Cai H, et al. (2008). Potent antitumor effect of TRAIL mediated by a novel adeno-associated viral vector targeting to telomerase activity for human hepatocellular carcinoma. J Gene Med 10:518–26
- Wang Y, Huang F, Cai H, et al. (2010). The efficacy of combination therapy using adeno-associated virus-TRAIL targeting to telomerase activity and cisplatin in a mice model of hepatocellular carcinoma. J Cancer Res Clin Oncol 136:1827–37
- Weitzman MD, Linden RM. (2011). Adeno-associated virus biology. Methods Mol Biol 807:1–23
- Wu P, Xiao W, Conlon T, et al. (2000). Mutational analysis of the adeno-associated virus type 2 (AAV2) capsid gene and construction of AAV2 vectors with altered tropism. J Virol 74:8635–47
- Xie Q, Bu W, Bhatia S, et al. (2002). The atomic structure of adeno-associated virus (AAV-2), a vector for human gene therapy. Proc Natl Acad Sci USA 99:10405–10
- Xu R, Sun X, Tse LY, et al. (2003). Long-term expression of angiostatin suppresses metastatic liver cancer in mice. Hepatology 37:1451–60
- Yuan L, Zhao H, Zhang L, Liu X. (2013). The efficacy of combination therapy using adeno-associated virus-mediated co-expression of apoptin and interleukin-24 on hepatocellular carcinoma. Tumor Biol 34:3027–34
- Zhang Y, Ma H, Zhang J, et al. (2008). AAV-mediated TRAIL gene expression driven by hTERT promoter suppressed human hepatocellular carcinoma growth in mice. Life Sci 82:1154–61
- Zhang Y, Ren JS, Shi JF, et al. (2015). International trends in primary liver cancer incidence from 1973 to 2007. BMC Cancer 15:94
- Zincarelli C, Soltys S, Rengo G, Rabinowitz JE. (2008). Analysis of AAV serotypes 1–9 mediated gene expression and tropism in mice after systemic injection. Mol Ther 16:1073–80