Abstract
Nano-scaled materials have been proved to be ideal DNA carriers for transgene. Bacterial magnetic particles (BMPs) help to reduce the toxicity of polyethylenimine (PEI), an efficient gene-transferring agent, and assist tissue transgene ex vivo. Here, the effectiveness of the BMP-PEI complex-conjugated foreign DNAs (BPDs) in promoting testes-mediated gene transfer (TMGT) in mouse was compared with that of liposome-conjugated foreign DNAs. The results proved that through testes injection, the clusters of BPDs successfully reached the cytoplasm and the nuclear of spermatogenesis cell, and expressed in testes of transgene founder mice. Additionally, the ratio of founder mice obtained from BPDs (88%) is about 3 times higher than the control (25%) (p < 0.05). Interestingly, the motility of sperms recovered from epididymis of the founder mice from BPD group were significantly improved, as compared with the control (p < 0.01). Based on classic breeding, the ratio of transgene mice within the first filial was significantly higher in BPDs compared with the control (73.8% versus 11.6%, p < 0.05). TMGT in this study did not produce visible histological changes in the testis. In conclusion, nano-scaled BPDs could be an alternative strategy for efficiently producing transgene mice in vivo.
Introduction
Genetically modified laboratory animal models are indispensable for biomedical researches. However, the most popular methods in producing such animals remains low efficiency, which either requires high technologies, expensive instruments, has safety risks or brings adverse effects upon cells and tissues (Smith, Citation2004; Parrington et al., Citation2011), and as a result, are not easily handled by individual laboratories. Previously developed spermatozoa-based gene transfer methods, including testis- and sperm-mediated gene transfer (TMGT and SMGT) can be performed by general researchers to achieve personalized gene modifications in animals (Coward et al., Citation2007; Collares et al., Citation2010; Amaral et al., Citation2011; Campos et al., Citation2011a,Citationb). Referring to TMGT, foreign DNA vehicles need to be excellent in penetrating thick tissues to achieve better output. Besides, although the uptake of foreign DNA by target cells could be improved through either “in vivo” electroporation or pre-hatching the DNA solution with materials such as liposome, calcium phosphate, and DMSO (Sato et al., Citation2002; Coward et al., Citation2007; Campos et al., Citation2011b), the output remains disappointed due to the poor DNA integration ratio and cytotoxicity of the mediators (Sato et al., Citation2002; Hooley et al., Citation2009). Alternatively, cationic macromolecular polymers, such as polyethylenimine (PEI), are attractive non-viral molecules for high transferring efficacy of nucleotides (Read et al., Citation2010; Ma et al., Citation2011; Marszall, Citation2011; Lee et al., Citation2012; Mandal et al., Citation2013). However, the cell toxicity of the material hinders its wide application for years as well (Chollet et al., Citation2002; Tong et al., Citation2005). Therefore, it is necessary to renovate low technique required gene delivery strategies that employs both cheaper and safer materials and has high efficiency (Coward et al., Citation2007; Villemejane & Mir, Citation2009; Patnaik et al., Citation2010; Parrington et al., Citation2011). From our point of view, the key point is to promote foreign DNAs diffusion across the testis interstitial tissue and improve the absorption by germ cells with reduced toxicity based on PEI.
Applying magnetic nanoparticle materials in biomedical science offers major advantages by their unique size and physicochemical properties. Of which, iron oxide nanoparticles, the magnetite (Fe3O4) are by far the most commonly employed particles for biomedical applications (Akbarzadeh et al., Citation2012). Bacterial magnetic particles (BMPs) are comprised by Fe3O4 particles of 45–55 nm in diameter and are enveloped by cytoplasmic membrane, which presents a friendly surface for the particles to penetrate the bio-membrane (Xie et al., Citation2009; Marszall, Citation2011). For instance, BMP-carrier gene vaccine plus a magnetic field results in tumor protection (Tang et al., Citation2012; Stanley et al., Citation2015). BMPs have been used as the carriers for nucleic acids (Ota et al., Citation2003) and have advantages over artificial iron oxide nanoparticles in that they can not only be cheaply produced and safely sterilized (Xiang et al., Citation2007), but that they can easily couple with bioactive macromolecules, anticancer drugs, or liposomes (Matsunaga et al., Citation2007; Rieck et al., Citation2013). Interestingly, combining BMPs to PEIs proves to be helpful for reducing the cytotoxicity of PEI in vitro and in vivo (Marszall, Citation2011; Zuo et al., Citation2012), but whether BMP-PEI complex can be used in TMGT or SMGT remains unclear.
Consequently, it is our interest to clarify if BMP-PEI complex-conjugated foreign DNAs (BPDs) can be used in TMGT as well. This study is then designed to clarify the effect of BPDs on improving TMGT in mice. The results showed that nano-scaled BPDs could be an alternative strategy for efficiently producing transgene mice in vivo.
Materials and methods
Chemicals and animals
All chemicals and reagents were purchased from Sigma-Aldrich Inc. (St. Louis. MO) unless otherwise indicated. DNA solution and injection buffer was purchased from Chemicon International (Merck KGaA, Darmstadt, Germany). Mouse embryo culture media, such as M2, M2 supplemented with hyaluronidase and M16 were purchased from Millipore (Billerica, MA). Equine chorionic gonadotrophin (eCG) was purchased from Ningbo Hormone Product Inc. (Ningbo, ZheJiang, China). PEI (25 k Da, pH 7.2) was diluted with de-ionized water to the working concentration of 2 μg/μl and stored at 4 °C until use.
Sexually matured (over 4-week-old female and 4-month-old male) FVB/N mice, and outbred ICR mice with normal fertility from the Academy of Military Medical Sciences, Beijing, China, were used for all TMGT experiments. Mice were kept under barrier housing facilities with controlled temperature (24–26 °C) and light (12 h light and 12 h dark cycles), with food and water ad libitum. Briefly, for intra-testicular injection, 24 male FVB/N mice were prepared. The experiments were carried out in accordance with the principles and guidelines for the use of laboratory animals and approved by Institutional Animal Care and Use Committee of the China Agricultural University.
BMPs preparation
The production and purification of BMPs were performed according to the previous report (Sun et al., Citation2008). In brief, the Magnetospirillum gryphiswaldense MSR-1 cells (German Collection of Microorganisms and Cell Cultures, Germany), which is characterized with its ability to accumulate BMPs within the cytoplasm, were submerged, cultured, and then re-suspended in 10 ml of 5 mM phosphate-buffered saline (PBS, pH 7.4) and disrupted by two passes through a French press at 1000 psi (Thermo Electron Corp., Waltham, MA, USA). After examining disrupted cells under an optical microscope, BMPs were collected using neodymium-iron-boron magnets (Nd-Fe-B magnet ⊄ 29 × 8 mm), which were produced by a non-homogeneous magnetic field (0.5 T at the surface). After removing the supernatant, the BMPs were washed 8–10 times with PBS using a mild ultrasonic bath (at 50 W). All of the BMPs were lyophilized by freeze/drying for 20 h, then sterilized by γ-rays (15 k Gy), and stored at −20 °C until further use. Whenever use, the magnetosomes were diluted with PBS (pH 7.4) at a concentration of 10 ng/μl and stored at 4 °C until use.
DNA preparation
The pAcGFP-N1 vector coding for green fluorescent protein (GFP) under the control of the cytomegalovirus (CMV) promoter as a reporter gene was used for transgene assays (). Briefly, the plasmid purchased from CLONTECH Laboratories Inc. (Mountain View, CA, USA). Purified plasmids with a ratio of OD 260/280 over 1.8 was used. The digested 4726 bp DNA fragment was re-suspended in Tris (1 mM) – EDTA (0.1 mM) buffer (pH 7.4). The store concentration was 1 μg/μl.
Figure 1. BPD preparation, intra-testicular injection, and foreign gene expression after TMGT in the testes. (A) The structure of pAcGFP-N1 plasmid as well as the loci of primers (red arrows) designed for PCR procedure. (B, C) Surgical procedures for intra-testicular injection, in which a 27-gauge needle is used to pierce the tunica albuginea of the testis at one of the ends of the long axis to assist penetration of mouth pipette into the tissue (B). Mouth pipette was withdrawn from the testis bit by bit while solution was injected into the testes (C). (D) The clusters of BMPs (red arrow) that have conjugated with PEI-DNAs (red arrow head) observed under TEM. The size distribution of BMPs ranged from 45 to 55 nm. Amplification: × 60 000. (E, F) BPDs were presented within the cells of seminiferous tubule (red arrows). BPDs were observed both within the cytoplasm as well as nuclear of spermatogenic cells (E, bar = 200 nm) and within the tails of sperms (F, bar = 500 nm). (G, H, I) In vivo and ex vivo GFP fluorescence detection. (G) The fluorescence of GFP protein was presented in the testes of BMP-PEI group mouse under KODAK Image Station In-Vivo FX system. (H) No fluorescence was observed in the testes of mice coming from both groups, as compared with the GFP transgene positive mice obtained through conventional pronuclear injection method (black mouse on the right), but can be detected under IVIS QUANTUM FX system when mouse testes were recovered. (J) The expression of GFP protein was shown in testes injected with BPDs and Lipo-DNAs (red arrow) under fluorescence microscope.
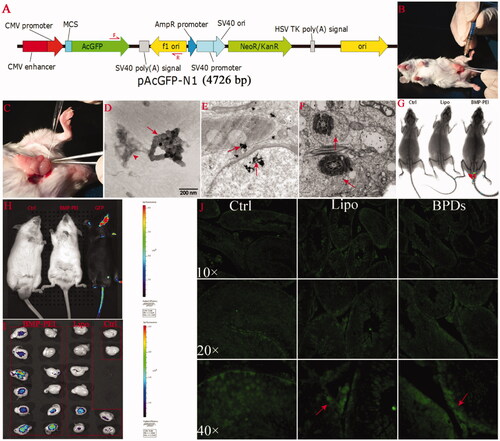
BMP-PEI-DNA complexes (BPDs) preparation
BPDs were prepared according to previous description (Xiang et al., Citation2007; Yang et al., Citation2016). In brief, the ratio among BMPs, PEI and DNA in the complex was fixed to 1:1.6:0.8 (μg/μg/μg). For each testis, immediately before injection, 8 μl (1 μg/μl) of foreign DNA solution for every testis was mixed with 8 μl PEI (2 μg/μl) and incubated for 10 min to form PBDs. Meanwhile, in order to thoroughly re-suspend the magnetosomes, the BMPs solution tube was firstly incubated in a mild ultrasonic bath (at 50 W) for 10–20 s and then immediately put on the ice for cooling, the procedure was repeated 3–4 times until the suspension turned into brown color and looked like half transparency. Then, quickly took 10 μl of BMPs (1 μg/μl) suspension and mixed with PEI-DNA solution mildly with the pipette for 10 s, and incubated the tube for 10 min for self-assemble.
Foreign plasmid pAcGFP-N1 mixed with liposome (lab made and commonly used) was used as the control (Lipo-DNAs in short). The mixture was prepared according to the manufacturer’s instruction. In brief, diluted 1 μl (1 μg/μl) of DNA in 50 μl of PBS (pH 7.4), mixed gently before incubating with liposome. Mixed liposome store solution gently before use, then diluted 3 μl liposome in 50 μl of PBS (pH 7.4), incubated for 5 min at room temperature. Then combined the diluted DNA and diluted liposome solution, mixed gently and incubated for 20 min at room temperature before use.
Whenever performing TMGT, 12 μl of either BPDs, Lipo-DNAs, or PBS (black control) was filled into a capacity sterile mouth pipette of 40 μm in diameter for testis injection assay.
For observing images of formed PBDs complexes under transmission electron microscopy (Tecnai G2 F30, FEI, OR, USA), 20 μl of the complexes were applied to the copper grids at an accelerating voltage of 300 kV.
Testes injection of BPDs
For TMGT experiments, mice were randomly grouped and respectively injected with either BMP-PEI-DNA complexes (BPDs) (19 mice), Lipo-DNA complexes (12 mice) (control), or PBS (3 mice) (pH 7.4) (blank control). The surgical operation protocol was as following. Mice were anesthetized with 40 mg/kg BW of 1% sodium pentobarbital. First, each testis of the individual mouse was gently pulled out from the celiac surgically. Before injection, carefully pierce the tunica albuginea of the testis at one of the ends of the long axis with a 27-gauge needle to assist penetration of mouth pipette into the tissue (). Then mouse pipette was plugged into 2/3 depth of the testis along the long axis through the wound. The suspension of the DNA complex was injected into the testis bit by bit while slowly withdrawing the pipette (). The injected volume was about 20–30 μl for each testis. After injection, the testis was returned into the celiac gently. After the other side of the testis injection was accomplished, the wound was sutured. Later, the mice were put onto a warm bed (25 °C) in supine position and a neodymium-iron-boron magnet with an intensity of 600 mT (Tang et al., Citation2012) was placed onto the surface of the abdomen until the mice recovered (5–10 min after operation).
In vivo and ex vivo examinations of transgene effect
For ex vivo examination, 3 days after surgery, the foreign DNA integration and expression effect in the testes of mice (6 from BPD group, 4 from Lipo-DNA group, and 1 from PBS group) undertaken TMGT were sacrificed. The testes of each mouse were immediately recovered. The GFP protein expression within the testes were examined through IVIS QUANTUM FX system (PerkinElmer, Waltham, MA, USA) according to the instructions of the producer.
For in vivo examination, 7 days after surgery, the foreign DNA integration and expression effect in the testes of mice undertaken TMGT were determined through a KODAK Image Station In-Vivo FX system (Eastman Kodak Company, Rochester, NY). In brief, mice (6 from BPD group, 4 from Lipo-DNA group, and 2 from PBS group) were anesthetized and denuded with 8% Na2S plus 30% ethanol in PBS solution. The examinations were immediately performed according to the instructions of the producer.
BMP particles detection through TEM in founder mice
To justify if BMPs are present inside the spermatogenic cells in TMGT mice, 7 days after surgery, the testes of the founder mice were sliced and observed under TEM (JEM 2100, Electronics Co., Ltd., Japan). Briefly, the testes were fixed with 2% glutaraldehyde and 1% osmium tetroxide, and then sliced and observed under TEM according to the instructions of the producer.
Histochemistry
Thirty days after TMGT, the influence of surgical injection of foreign solutions on testes histological structure of the founder mice was evaluated. For histological examination, the testes of mice injected with BPDs and PBS were fixed, embedded in paraffin, and sectioned to a thickness of 5 μm. After de-waxing, rehydration, the sections were dyed with hematoxylin-eosin staining. The structures of testes were examined under a microscope (Eclipse, Nikon, Japan).
Immunofluorescence
Twenty-five hours after TMGT, the influence of surgical injection of foreign solutions on testes histological structure of the founder mice was evaluated through immunofluorescence examination. Briefly, the testes of mice injected with BPDs and PBS were fixed, embedded in paraffin, and sectioned to a thickness of 5 μm. After de-waxing, rehydration, microwave antigen repair and sealed by serum, the sections were dyed with propidium iodide solution (BioLegend, San Diego, CA, USA) for nucleus and Chk pAb to GFP (Abcam, Cambridge, UK) for GFP protein. The structures and fluorescence of testes were examined under a microscope (Eclipse, Nikon, Japan).
Spermatozoa motility analyses
The influence of BPDs and Lipo-DNAs on spermatozoa motility in epididymis of the founder mice was evaluated according to previous reports (Bian et al., Citation2012). In brief, 30 days after TMGT, spermatozoa from vas deference and caudal epididymis of TMGT mice were cultured with mT6 medium at a 37.8 °C incubator under 5% CO2 for 1.5 h to capacitate in vitro. For spermatozoa motility analysis, a CASA system (Version 12 CEROS, Hamilton Thorne Research, Beverly, MA) was used with the following settings: for cell detection: minimal contrast, 50; minimal cell size, 4 pixels; and 60 frames were acquired at a frame rate of 60 Hz. At least 100 tracks were measured for each sample at 37.8 °C with a Slide Warmer (#720230, Hamilton Thorne Research, Beverly, MA). The measured kinetic parameters were: VAP, average path velocity; VCL, curvilinear velocity; VSL, straight line velocity; ALH, amplitude of lateral head displacement; BCF, beat cross frequency; LIN, percentage of linearity (VSL/VCL 100%); STR, percentage of straightness.
Polymerase chain reaction (PCR) procedure
To detect the integration ratio of foreign DNAs in all candidate transgene positive mice, the DNAs from the tail tissue of each mouse mice of the first generation of all founders were extracted by using phenol-chloroform extraction and ethanol precipitation method, as has been reported previously (Zuo et al., Citation2012). The samples were then quantified by measuring the A260/A280 value through a microplate absorbance reader (Bio-Rad 680, Hercules, CA, USA). And the quality of each sample was evaluated by agarose gel electrophoresis. Then, the samples were diluted to 100 ng/μl and stored at −20 °C for later use as templates in PCR.
Each 25 μl PCR amplification system contained the following reagents: 12.5 μl 2 × Mix (Zomanbio, Beijing, China), 9.5 μl dH2O, 0.5 μM of each GFP specific primer (Shanghai Bio-Engineering Co., Shanghai, China), and 2 μl template DNA. PCR protocol was: pre-denaturation at 95 °C for 5 min; 35 cycles of denaturation at 95 °C for 30 s, annealing at 60 °C for 30 s, and extension at 72 °C for 30 s; and a final extension at 72 °C for 5 min. The sequences of the GFP primers were: forward: 5′-TACCCTGGTGAATCGCATCG-3′ and reverse: 5′-TTTCGCCCTTTGACGTTGGA-3′. These primers amplified a 750 bp sequence of the GFP gene. PCR was performed with a Bio-Rad thermocycler (Hercules, CA, USA), and the products were subjected to electrophoresis in a 1.5% agarose gel. The band signals were acquired using Infinity 3026 analysis software. The GFP PCR amplicons were sequenced to confirm that they were identical to the gene sequences. The PCR procedure was double checked in 2 weeks by collecting fresh samples.
Data analysis
Data were analyzed either by t-test and ANOVA (for analysis of sperm motility data only). Values of p < 0.05 were considered statistically significant.
Results
BMP conjugated successfully with PEI-DNA complex
With the help of TEM detection, BMP particles were proved to be able to conjugate with PEI-DNA complexes successfully. As shown in , the diameter of each BMP was approximately 45–55 nm size. In PBS buffer, BMP particles, PEI and DNAs conjugated to each other excellently, where the BMP particles generally formed into clusters (red arrow), and the PEI-DNA complexes conjugated to the cluster of BMPs (red arrow head). The results indicated that BMP cluster could conjugate with PEI-DNA complex steadily.
BPDs can get into nuclear of spermatogenic cells
As was demonstrated by TEM observation of BPDs-injected testes tissue, in seminiferous tubule, the presentation of BPDs within the cytoplasm as well as nuclear of spermatogenic cells were detected (, red arrows). In addition, the BPD clusters were detected in the cytoplasm of sperm tails as well (). The results imply that BPDs could penetrate the membranes of a cell and reach the chromosomes of spermatogenesis cells easily.
Transgene was expressed in testes of both BPD and Lipo-DNA groups
In order to evaluate if foreign DNAs could be expressed within the testes, the GFP proteins within testes of both BPD and Lipo-DNA group mice were detected through both in vivo ( and ) and ex vivo () live image systems. Under KODAK Image Station In-Vivo FX system, the GFP expression was observed while the testes were intact within denuded mice body (). On the other hand, under, IVIS QUANTUM FX system, although GFP expression could not be detected while the testes were intact within the mice, as compared with the GFP transgene positive mice obtained through conventional pronuclear injection method (black mouse on the right) (), the GFP fluorescence was observed after testes were recovered from the body (). Meanwhile, the GFP protein was detected in testes 25 h after injection in both groups except control (). The results showed that the sensitivity of the live fluorescence image in the two systems have respective characters. The GFP protein could express in testes of both BPD- as well as Lipo-DNA-injected mice.
BPDs is superior to Lipo-DNAs in preserving sperm motility
In order to evaluate the toxicity of BMP-PEI-mediated gene modification compared to commercial liposome products, the sperm mobility within epididymic ducts of TMGT mice were examined. In this aspect, multiple parameters for judging the motility of sperms were considered, including curvilinear velocity (VCL), straight-line velocity (VSL), average path velocity (VAP), amplitude of lateral head displacement (ALH), beat cross frequency (BCF), percentage of linearity (LIN = VSL/VCL × 100%), and percentage of straightness (STR = VSL/VAP × 100%). The results showed that almost all of the parameters of BPD groups, except ALH, were most significantly higher than those in Lipo-DNAs and controls (p < 0.01) (). The results approved the fact that BPDs have less toxicity over Lipo-DNAs in preserving sperm motility.
Figure 2. The influence of different DNA carriers on sperm motility after TMGT. From chart A through D, different letters in each chart indicate significant differences among groups (ANOVA, p < 0.05). As can be seen, almost all sperm motility parameters were significantly different among BPDs, Lipo-DNAs, and the controls except ALH (B). In addition, the increase of VAP, VSL, VCL, LIN, and STR in BPD groups, which represents the improved sperm motility, was significantly higher than the Lipo-DNA groups.
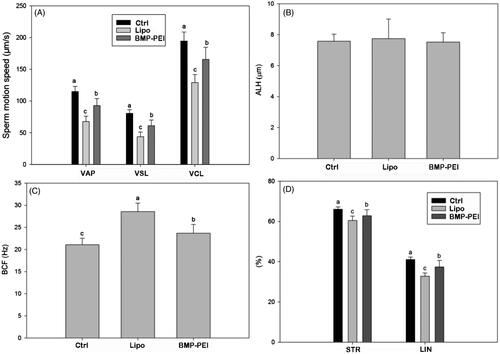
Transgenic efficiency in BPD group is higher than Lipo-DNA group
In order to demonstrate that the injected GFP plasmids could integrate into sperm chromosomes and be passed to the filial, the founder mice of both groups were mated to respective wild type mice. Then the genotypes of the filials of the first generations were examined through PCR procedures (). The results showed that both BPDs and Lipo-DNAs could be passed to the offspring of the founders (). However, the transgenic heterozygous mice obtained from BPDs were almost 6 times higher than the Lipo-DNA treatment (73.8% versus 11.6%, p < 0.05) (). When the heterozygous of the BPD group-derived mice were inbred to each other, transgene homozygous mice were obtained, as PCR results showed (). In addition to that, the GFP fluorescence was observed under KODAK system as well (). Therefore, although the heredity of transgene is not 100%, both BMP-PEI- and liposome-conjugated DNAs could be passed from founder mice to their offspring.
Figure 3. Genotype analysis of the first- and the second generations of founder mice derived from TMGT. (A, B) Demonstration of PCR analysis of plasmid encoding GFP integration in the chromosomes of offspring derived from some of the founder mice tail tissue. A: BPDs; B: liposome. (C) The ratios of heterozygous in the first filial between BPD and Lipo-DNA treatments, of which the ratio in BPDs was 6 times higher than the Lipo-DNA group (73.8% versus 11.6%, p < 0.05). (D) Demonstration of the genotypes of the second generation of founder mice after BPD injection. It showed that transgene can be successfully inherited by the second filial after inbreeding of the first filial. (E) Demonstration of the transgene that was expressed in some of the tissues of the second filial bodies after inbreeding of the first filials, as was approved by Kodak Image Station In-Vivo FX system. These transgene mice came from the founder mice No. 1.
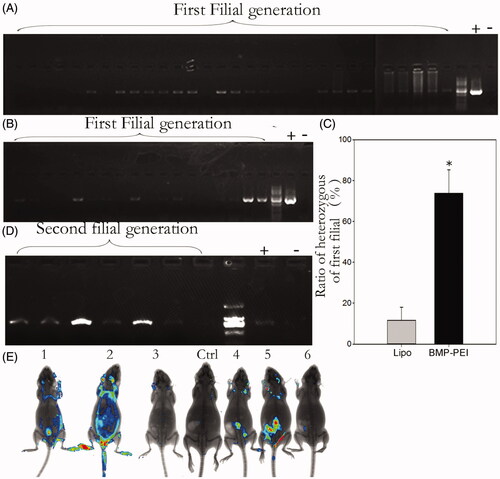
By examine the transgenic positive offspring obtained from founder mice with PCR, the ratio of founder obtained from BPDs versus Lipo-DNAs was confirmed. As a result, the ratio of founders obtained from injection of BPDs is significantly higher than that from Lipo-DNAs (88% versus 25%, p < 0.05) (). Besides, after supervised the reproduction of the heterozygous, the average litter size between the two groups was proved to be similar to each other (). Last, the histochemistry observation assay showed that there were no obvious damages after the testes of mice were performed with TMGT in this study (). Therefore, the results from this study confirmed the hypothesis that BPDs improves transgene efficiency.
Figure 4. The efficiency of TMGT between BPD and Lipo-DNA groups. (A) The ratios of founder mice obtained from different treatments were significantly different from each other, in which founder mice in BPDs were 3 times more than that in Lipo-DNA group. (B) Upon litter size of founders, there was no significant difference between the two groups. * Indicated significant different (p < 0.05).
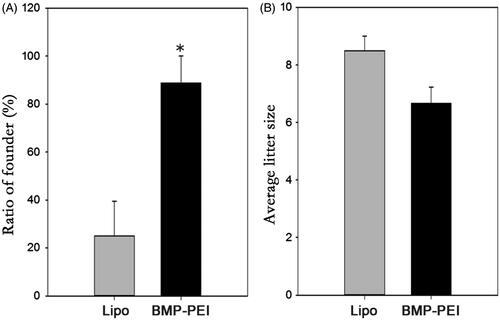
Discussion
In this study, although only few mice were injected with BPDs into their testes, a relatively satisfied output was obtained. As a result, as many as 11 of all 13 injected mice were founders, the production efficiency of which is 3 times more than that of Lipo-DNA group (4 out of 11 mice). The efficiency of producing heterozygous transgene mice from founders was also greatly different between BPDs and the control. Another advantage of BPDs over Lipo-DNAs was its less toxicity to the sperm motility. According to our previous study, BPDs could enhance gene expression of the foreign plasmid in mouse skeleton muscles (Xiang et al., Citation2007). Altogether, we have showed that BMPs could be an excellent nanoparticle that can assist foreign DNAs transgene in mice and have less toxicity to host cells. Besides, after carefully evaluating the economic and labor factors that influence transgene efficiency between TMGT and traditional transgene production protocols, the authors conclude that TMGT through applying BPDs helps to improve transgene efficiency in several aspects (). This method may be especially suitable for individual laboratories which lack experienced technicians and necessary equipment to produce transgene mice through pronuclear injection method.
Table 1. Comparison of the efficacy of producing founder mice between traditional transgene mice production method and TMGT method.
The difficulty of transferring plasmid DNA into the nucleus of a cell has long been a major problem in the successful production of non-viral transgenic animals (Villemejane & Mir, Citation2009; Kim et al., Citation2010; Campos et al., Citation2011a,Citationb). For instance, although a single injection of circular plasmid DNA encapsulated within a liposome was sufficient for transfection of sperm cells (Sato et al., Citation1999), liposome-mediated transgene seemed to be less effective (4% founder obtained) (Yonezawa et al., Citation2001). According to the study of He et al., a repeated injection of GFP cDNA into the testis at multiple sites resulted in 41% transgenic founder mice and 37% transgenic positive mice in the first generation (He et al., Citation2006). However, Sato and Nakamura indicated that repeated injections (3 and 6 repeated injections, 3 days apart) were not critical for introducing high copy numbers of DNA into offspring (Sato & Nakamura, Citation2004). Furthermore, although DNA-DMSO complex could produce as many as 61%, 55%, and 80% of transgenic founders in mouse and/or rabbit through testes injection, DMSO-containing solution induces testicular degeneration and reduces vascularization around seminiferous tubules (Shen et al., Citation2006; Amaral et al., Citation2011). Notably, the efficiency of producing transgenic founder mice through one injection procedure performed in this study by applying BMPs was 88%, which was higher than existed reports.
According to previous studies, magnetic nanoparticles bound to exogenous DNA localized either within or on the plasma membrane, whereas most liposome-bound exogenous DNA localized on the plasma membrane (Scherer et al., Citation2002). With the help of a magnetic force, BMP may accelerate the accumulation of complexes on the surface of cells and improve nuclear uptake of magnetic particles in the process of magnetofection (Tang et al., Citation2012). This is especially in agree with our study where the clusters of BMP complex were presented inside and outside of membrane of nuclear in spermatogenic cells of the founders’ testes. It indicates that under the magnetic force, although BMP itself could not improve the DNA integration efficiency, BMPs with natural bio-membrane may provide more opportunity for the DNA to get across the membranes of host cells and reach the nuclear, which increases the possibility of foreign DNAs to integrate to the chromosomes and therefore achieve high efficiency (Xiang et al., Citation2007).
As has been reported, the 25 kDa PEI combines a strong DNA compaction capacity with an intrinsic endosomolytic activity, namely “proton sponge effect”, for transporting DNAs toward the nucleus (Read et al., Citation2010; Ma et al., Citation2011). PEI is capable of condensing DNA into nano-size polyplexes and improves gene delivery efficiency (Felgner et al., Citation1997). However, the non-biodegradable C-C or C-N bonds within its structure induce accumulative cytotoxicity in vitro and in vivo (Chollet et al., Citation2002; Lu et al., Citation2006; Xiang et al., Citation2007; Wang et al., Citation2012). And such cationic polymers may interact with serum proteins resulting in their rapid clearance from the bloodstream, which make it not permissible for use in human. One effective way to diminish the toxicity of PEIs is to reduce the positive charges of PEI (Dash et al., Citation1999; Chollet et al., Citation2002; Patnaik et al., Citation2010). Magnetosomes are attractive carriers of therapeutic drugs and genes for medicines for a long time (Tang et al., Citation2012). Whenever BMPs conjugating to the PEIs, the positive charges of PEI can be counteracted by the negative charges on the membrane of BMPs (Fischer et al., Citation1999). Our previous study approved that the BPDs may have no observable influence on the viability of cells in contrast to the cytotoxicity of PEI (Xiang et al., Citation2007). Additionally, DNases present in the seminal fluid can degrade exogenous DNA molecules. Li et al. approved that BMPs-PEI complexes could bind DNA and provide protection from DNase degradation (Xiang et al., Citation2007). Similarly, Kim et al. supplied additional proofs that magnetic nanoparticles is better than liposomes in resisting the activity of DNase I from digesting exogenous DNA binding to sperm (Kim et al., Citation2010). Therefore, BMP may contribute to reduce the toxicity of PEI in our study and promotes transgene.
Although this study obtained proofs of BPDs improved transgenic efficiency in inbred mice strains, it is necessary to verify the effectiveness as well as the applicability among other laboratory animals, such as miniature pigs, nonhuman primates, and large livestock. Recently, a preliminary study of us showed that BPDs was able to deliver foreign DNAs successfully into the testes of sheep (2 out of 4), as were judged from the ejaculated semen 1 month after surgery (data not shown). However, large animals have considerably big gonads, which make it difficult to adjust the volume of solution buffer as well as the concentration of final BPDs. Additional studies also should concentrate on evaluate if BPDs could assist gene modification via nuclease-based gene targeting, including CRISPR/Cas9 as well as NgAgo–gDNA systems (Jinek et al., Citation2012; Mali et al., Citation2013; Pauwels et al., Citation2014; Gao et al., Citation2016; Vassena et al., Citation2016).
In conclusion, BPDs applied in this study improve TMGT transgene efficiency, which could be a promising strategy for conveying foreign genes into the testes and achieve transgene effectively.
Declaration of interest
The authors report no declarations of interest.
This work was supported by National Basic Research Program of China (2013CB945501; 2014CB943202) and the Project of State Key Laboratory of Agrobiotechnology (2015SKLAB1-5).
Acknowledgements
The authors thank Dr. Enkui Duan and Dr. Qi Chen (State Key Laboratory of Reproductive Biology, Institute of Zoology, Chinese Academy of Sciences, Beijing China) for their help in spermatozoa motility analysis.
References
- Akbarzadeh A, Samiei M, Davaran S. (2012). Magnetic nanoparticles: preparation, physical properties, and applications in biomedicine. Nanoscale Res Lett 7:144.
- Amaral MG, Campos VF, Seixas FK, et al. (2011). Testis-mediated gene transfer in mice: comparison of transfection reagents regarding transgene transmission and testicular damage. Biol Res 44:229–34.
- Bian F, Mao G, Guo M, et al. (2012). Gradients of natriuretic peptide precursor A (NPPA) in oviduct and of natriuretic peptide receptor 1 (NPR1) in spermatozoon are involved in mouse sperm chemotaxis and fertilization. J Cell Physiol 227:2230–9.
- Campos VF, Komninou ER, Urtiaga G, et al. (2011a). NanoSMGT: transfection of exogenous DNA on sex-sorted bovine sperm using nanopolymer. Theriogenology 75:1476–81.
- Campos VF, Leon PM, Komninou ER, et al. (2011b). NanoSMGT: transgene transmission into bovine embryos using halloysite clay nanotubes or nanopolymer to improve transfection efficiency. Theriogenology 76:1552–60.
- Chollet P, Favrot MC, Hurbin A, et al. (2002). Side-effects of a systemic injection of linear polyethylenimine-DNA complexes. J Gene Med 4:84–91.
- Collares T, Campos VF, Seixas FK, et al. (2010). Transgene transmission in South American catfish (Rhamdia quelen) larvae by sperm-mediated gene transfer. J Biosci 35:39–47.
- Coward K, Kubota H, Parrington J. (2007). In vivo gene transfer into testis and sperm: developments and future application. Arch Androl 53:187–97.
- Dash PR, Read ML, Barrett LB, et al. (1999). Factors affecting blood clearance and in vivo distribution of polyelectrolyte complexes for gene delivery. Gene Ther 6:643–50.
- Felgner PL, Barenholz Y, Behr JP, et al. (1997). Nomenclature for synthetic gene delivery systems. Hum Gene Ther 8:511–2.
- Fischer D, Bieber T, Li Y, et al. (1999). A novel non-viral vector for DNA delivery based on low molecular weight, branched polyethylenimine: effect of molecular weight on transfection efficiency and cytotoxicity. Pharm Res 16:1273–9.
- Gao F, Shen XZ, Jiang F, et al. (2016). DNA-guided genome editing using the Natronobacterium gregoryi Argonaute. Nat Biotechnol 34:768–73.
- He X, Qi B, Liu G, et al. (2006). A novel method to transfer gene in vivo system. Prog Biochem Biophys 33:685–90.
- Hooley RP, Paterson M, Brown P, et al. (2009). Intra-testicular injection of adenoviral constructs results in Sertoli cell-specific gene expression and disruption of the seminiferous epithelium. Reproduction 137:361–70.
- Jinek M, Chylinski K, Fonfara I, et al. (2012). A programmable dual-RNA-guided DNA endonuclease in adaptive bacterial immunity. Science 337:816–21.
- Kim TS, Lee SH, Gang GT, et al. (2010). Exogenous DNA uptake of boar spermatozoa by a magnetic nanoparticle vector system. Reprod Domest Anim 45:e201–6.
- Lee HJ, Nguyen YTC, Muthiah M, et al. (2012). MR traceable delivery of p53 tumor suppressor gene by PEI-functionalized superparamagnetic iron oxide nanoparticles. J Biomed Nanotechnol 8:361–71.
- Lu L, Lin M, Xu M, et al. (2006). Gene functional research using polyethylenimine-mediated in vivo gene transfection into mouse spermatogenic cells. Asian J Androl 8:53–9.
- Ma K, Shen HJ, Shen S, et al. (2011). Development of a successive targeting liposome with multi-ligand for efficient targeting gene delivery. J Gene Med 13:290–301.
- Mali P, Yang L, Esvelt KM, et al. (2013). RNA-guided human genome engineering via Cas9. Science 339:823–6.
- Mandal A, Sekar S, Chandrasekaran N, et al. (2013). Poly(ethylene) glycol-capped silver and magnetic nanoparticles: synthesis, characterization, and comparison of bactericidal and cytotoxic effects. Proc Inst Mech Eng H 227:1224–36.
- Marszall MP. (2011). Application of magnetic nanoparticles in pharmaceutical sciences. Pharm Res 28:480–3.
- Matsunaga T, Suzuki T, Tanaka M, et al. (2007). Molecular analysis of magnetotactic bacteria and development of functional bacterial magnetic particles for nano-biotechnology. Trends Biotechnol 25:182–8.
- Ota H, Takeyama H, Nakayama H, et al. (2003). SNP detection in transforming growth factor-beta1 gene using bacterial magnetic particles. Biosens Bioelectron 18:683–7.
- Parrington J, Coward K, Gadea J. (2011). Sperm and testis mediated DNA transfer as a means of gene therapy. Syst Biol Reprod Med 57:35–42.
- Patnaik S, Arif M, Pathak A, et al. (2010). PEI-alginate nanocomposites: efficient non-viral vectors for nucleic acids. Int J Pharm 385:194–202.
- Pauwels K, Podevin N, Breyer D, et al. (2014). Engineering nucleases for gene targeting: safety and regulatory considerations. Nat Biotechnol 31:18–27.
- Read SP, Cashman SM, Kumar-Singh R. (2010). POD nanoparticles expressing GDNF provide structural and functional rescue of light-induced retinal degeneration in an adult mouse. Mol Ther 18:1917–26.
- Rieck S, Zimmermann K, Wenzel D. (2013). Transduction of murine embryonic stem cells by magnetic nanoparticle-assisted lentiviral gene transfer. Methods Mol Biol 1058:89–96.
- Sato M, Yabuki K, Watanabe T, Kimura M. (1999). Sperm-mediated gene transfer by direct injection of foreign DNA into mouse testis. Transgenics 2:357–69.
- Sato M, Ishikawa A, Kimura M. (2002). Direct injection of foreign DNA into mouse testis as a possible in vivo gene transfer system via epididymal spermatozoa. Mol Reprod Dev 61:49–56.
- Sato M, Nakamura S. (2004). Testis-mediated gene transfer (TMGT) in mice: effects of repeated DNA injections on the efficiency of gene delivery and expression. Transgenics 4:101–19.
- Scherer F, Anton M, Schillinger U, et al. (2002). Magnetofection: enhancing and targeting gene delivery by magnetic force in vitro and in vivo. Gene Ther 9:102–9.
- Shen W, Li L, Pan QJ, et al. (2006). Efficient and simple production of transgenic mice and rabbits using the new DMSO-sperm mediated exogenous DNA transfer method. Mol Reprod Dev 73:589–94.
- Smith KR. (2004). Gene therapy: the potential applicability of gene transfer technology to the human germline. Int J Med Sci 1:76–91.
- Stanley SA, Sauer J, Kane RS, et al. (2015). Remote regulation of glucose homeostasis in mice using genetically encoded nanoparticles. Nat Med 21:92–8.
- Sun JB, Zhao F, Tang T, et al. (2008). High-yield growth and magnetosome formation by Magnetospirillum gryphiswaldense MSR-1 in an oxygen-controlled fermentor supplied solely with air. Appl Microbiol Biotechnol 79:389–97.
- Tang T, Zhang L, Gao R, et al. (2012). Fluorescence imaging and targeted distribution of bacterial magnetic particles in nude mice. Appl Microbiol Biotechnol 94:495–503.
- Tang YS, Wang D, Zhou C, et al. (2012). Bacterial magnetic particles as a novel and efficient gene vaccine delivery system. Gene Ther 19:1187–95.
- Tong AW, Zhang YA, Nemunaitis J. (2005). Small interfering RNA for experimental cancer therapy. Curr Opin Mol Ther 7:114–24.
- Vassena R, Heindryckx B, Peco R. (2016). Genome engineering through CRISPR/Cas9 technology in the human germline and pluripotent stem cells. Hum Reprod Update 22:411–9.
- Villemejane J, Mir LM. (2009). Physical methods of nucleic acid transfer: general concepts and applications. Br J Pharmacol 157:207–19.
- Wang YQ, Su J, Wu F, et al. (2012). Biscarbamate cross-linked polyethylenimine derivative with low molecular weight, low cytotoxicity, and high efficiency for gene delivery. Int J Nanomedicine 7:693–704.
- Xiang L, Wang B, Jin H, et al. (2007). Bacterial magnetic particles (BMPs)-PEI as a novel and efficient non-viral gene delivery system. J Gene Med 9:679–90.
- Xiang L, Wei J, Jianbo S, et al. (2007). Purified and sterilized magnetosomes from Magnetospirillum gryphiswaldense MSR-1 were not toxic to mouse fibroblasts in vitro. Lett Appl Microbiol 45:75–81.
- Xie J, Chen K, Chen X. (2009). Production, modification and bio-applications of magnetic nanoparticles gestated by magnetotactic bacteria. Nano Res 2:261–78.
- Yang W, Bai Y, Wang X, et al. (2016). Attaching biosynthesized bacterial magnetic particles to polyethylenimine enhances gene delivery into mammalian cells. J Biomed Nanotechnol 12:789–99.
- Yonezawa T, Furuhata Y, Hirabayashi K, et al. (2001). Detection of transgene in progeny at different developmental stages following testis-mediated gene transfer. Mol Reprod Dev 60:196–201.
- Zuo B, Du X, Zhao J, et al. (2012). Analysis of microsatellite polymorphism in inbred knockout mice. PLoS One 7:e34555.